Abstract
Improvement of magnetic properties, suggesting the effectiveness of Li0.5Fe2.5O4 (LF) in lower concentration doped in BaTi0.9Zr0.1O3 (BTZr). The composites with a formula of (1-x)BaTi0.9Zr0.1O3 + (x) Li0.5Fe2.5O4 (x = 0.0, 0.05, 0.10 and 0.15) synthesized by conventional solid state reaction method at 1150 °C. X-ray diffraction (XRD) peaks are indexed to the crystal planes for tetragonal perovskite and spinel ferrite observed in the samples. SEM micrographs show dense microstructure resulting in increasing grain size with an increase in ferrite content. Stability of magnetic phase in the higher temperature range, enhancing the Curie temperature with increase in ferrite content.
1 Introduction
The universal magnetic properties of materials such as ferrite, was substituted in ferroelectric, then improve their magnetic properties which explode rapidly due their numerous applications. The applications of ferrite depend on various electrical and magnetic properties. Multiferroic materials exhibit more than one predominant ferroic phase such as ferromagnetism, ferroelectricity, ferroelasticity, etc. in single phase materials investigated in recent years [Citation1,Citation2]. One of the important ferroelectric materials is BaTiO3, investigated for its ferroelectric nature, high dielectric constant and low Curie temperature [Citation3]. The doping of other host ions in BaTiO3 gives to exceptional improvements in its various features such as multifunctional properties [Citation4,Citation5]. These multifunctional properties in magneto-electric systems, have two order parameters with a certain degree of coupling between them [Citation6,Citation7]. The addition of ferrite with barium titanate showed improved stability of magnetic property [Citation8]. A combination of two phases such as a ferrite and piezoelectric phase or magnetostrictive and piezoelectric phases were provide appropriate for ME property [Citation9]. Magneto-electric (ME) effect on the composite materials could be the result of tensor property resulting from both magnetic phase and piezoelectric effects via elastic interaction [Citation10]. The coupling effect for ME also suggests the effect of magnetic field on the magnetization polarization behavior of the material. Change in magnetization at the vicinity of coercivity suggesting the switching of the magnetization correlating switching of the electric field induced from magnetic field entropy [Citation11,Citation12]. The ability to generate the magnetic response in the ME structures with high magnetization under low magnetic field with minimum coercivity. Tunable microwave devices based on the ferrite/ferroelectric showing magnetism based on control of exchange bias or tuning of magnetic states [Citation13,Citation14]. Epitaxial of cobalt ferrite with BaTiO3 showed strong coupling through hetroepitaxy of the lattices of spinel perovskite systems [Citation15] Highest magnetization was observed in BaTiO3-BiFeO3 composites with highest magnetization of 2.3 emu cm−3 attributed to magnetic moments and exchange coupling of the ferromagnetic phases [Citation16]. Lithium substitution showed stable, improved saturation polarization and dielectric nature at low frequencies for higher concentration of lithium in our previous investigation [Citation17]. To improve the further characteristics, the zirconium was accommodated in the perovskite tetragonal crystal structure of the BaTiO3, bringing stability and lowering the leakage current. The ferroelectric BTZr had a piezoelectric strain coefficient, high dielectric constant and which has potential applications in microwave technology. Since the barium titanate doped zirconium (BTZr) has tetragonal and good ferroelectric material with a perovskite structure, it can be easily substituted by lithium ferrite transition metals [Citation18 Citation2013;Citation20]. The ferrite doped to BTZr having magnetic ions such as Fe3+, have been used to substitute Ti4+ for stabilization at elevated temperature phase leading to polymorphic, exhibiting both tetragonal and cubic phases [Citation9,Citation21,Citation22]. In continuation of our previous work [Citation17], to improve the stability and enhancement of Curie temperature, zirconium was added in lower concentration in BaTiO3 and mixed with a lower concentration of ferrite. The objective of the present work is to investigate this significant change observed for magnetic properties and variation of Curie temperature.
2 Experimental
The mixing of LF and BTZr composites were prepared by using conventional solid state reaction method. The Ba2Co3, Zr2O3, TiO2, Li2CO3, and Fe2O3 powders were mixed together by using agate motor. These compositions are weighed as per stoichiometric ratio. The compounds were grinded for 10 h to obtain a homogeneous fine powder and distribution of the ingredients. The samples were calcinated at 900 °C temperature for 3 h. The calcinated powders were again grinded for 3 h, after that binder was added to the mixed calcinated powder and grind to mix uniformly up to become fine powder. The powder is placed into the die set and pressing by applying pressure 5 t for 3–4 min by using hydraulic press, powder formed as pellets. These pellets were sintered temperature at 1150 °C for 3 h. The pellets were used for different characterization.
3 Results and discussions
3.1 X-ray diffraction
The X-ray diffraction pattern of composites of 2θ range between 20–80° observed at room temperature. The X-ray diffraction (XRD) patterns of the BaTi0.9Zr0.1O3 (BTZr), Li0.5Fe2.5O4 (LF), (0.95)BTZr + (0.05) LF, (0.90) BTZr + (0.10) LF and (0.85) BTZr + (0.15) LF. The XRD patterns suggest formation of single phase tetragonal perovskite structure for BTZr, cubic spinel structure for LF and composite phase for composites was shown in .
XRD patterns for the BTZr with LF composites different concentrations shows the phases of the perovskite BTZr with LF in adequate proportions with major phase perovskite BTZr and minor phase spinel LF of composites. These have been described as the presence of the minor phase in these composites is due to minor diffusion between adjacent ferroelectric and ferromagnetic phases with lower percentage of LF [Citation23,Citation24]. XRD peaks are well indexed to the crystal planes for tetragonal perovskite and spinel ferrite exhibiting both the ferroelectric phase and low ferrite in component phases [Citation25]. XRD pattern also shows reducing intensity peaks with the major phases for BTZr and increasing minor phases for increases in ferrite (LF) in composites. No extra peaks are identified, indicating that no impurity phase formed during the sintering process in the composites. The lattice constants have been calculated from the primary peaks of ferroelectric and ferrite phases. The values of lattice parameter for different compositions are presented in . Average crystallite size of composites was calculated by using Debye Scherrer’s relation is presented in .
Table 1 Lattice parameters and average particle size of BTZr and composites.
3.2 Microstructural analysis
Scanning electron micrographs of BTZr and its composites are shown in and grain size was calculated by using imageJ software [Citation26]. It can be viewed that BTZr composites exhibit fully dense microstructure with small pores. The grain of BTZr seems to be clear larger appeared as spherical shaped regions and with increasing percentage of ferrite, composites it seems to be cylindrical region. The ferrite doped composites also exhibit clear grain boundaries and grain growth seems to be continuously increasing. The average grain of the composites increases slightly for x = 0.05, 0.10 and large increase for x = 0.15. The average grain size of (1 − x) BTZr + (x) LF at x = 0, 0.05, 0.10 and 0.15 were estimated nearly around 0.2 μm, 0.3 μm, 0.9 μm and 2 μm respectively.
3.3 Magnetic properties
The magnetic properties of (1 − x) BTZr + (x) LF have been investigated by measuring the magnetic virgin curve (initially increasing magnetization curve by applying filed) and magnetization varies with a magnetic field (M–H loop) at room temperature are shown in respectively. shows the nonmagnetic nature of BTZr composites with ordered magnetic structure and improves with an increase in ferrite content. These composites exhibit saturation magnetization (Ms) increases showing coercivity and remanance tabulated in . Increases the magnetic moment and magnetization may be due to present of the LF grains, which act as centers of the magnetization. The saturation magnetization resulting from the vector sum of all these individual contributions [Citation27,Citation28]. It was also noticed that saturation magnetization values of the composites found to be less than that of pure LF ceramic [Citation17].
Table 2 Ferromagnetic parameters of (1 − x) BTZr + (x) LF composite ceramics.
Fig. 3 Magnetic hysteresis loops of the (1 − x) BTZr + (x) LF composites. Lower inset shows Mr and Hc presence for different concentration of ferrite.
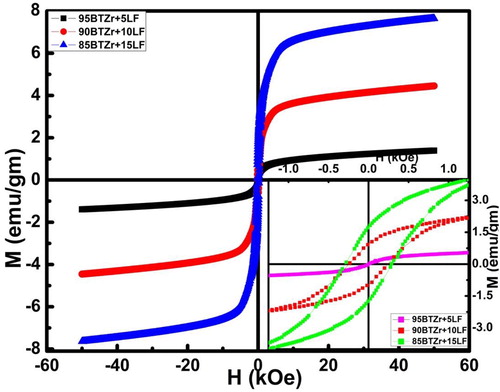
Remnant magnetization of BTZr composites also increases with increase in ferrite concentration and the coercive field (Hc) is also found to shift towards a higher field in composites. This may be due to magnetic domain wall motion and rotation in the composites due to ferrite grains, which contributes [Citation3,Citation17]. The values (Hc,Mr) of composites are tabulated in and these values are less when compared to our previous investigation [Citation17]. This reduction may be due to the Zr4+ was substituted in place of Ti4+ ions in the octahedral sites, gives rise to Fe 2+ or Ti3+ ions in the composition of composites creating a charge fluctuation for multivalent compounds [Citation29]. Non magnetic interaction exists between Zr4+and Fe 3+ but Zr4+ doping at Ti-site of BaTiO3 and Fe–O–Fe in ferrite grains gives disorder interchange interactions [Citation30].
By increasing ferrite content through LF in the composites, may cause the difference of Hc in the composites resulting strain in the ferrite phase by which lattice mismatches occur between ferrite and ferroelectric phases. BTZr in the presence of magnetic field behave as a pore causing the reduction of magnetic parameters observed complete ferrite phase in composites. Magnetization in all composites was saturated for higher magnetic field strength.
After reaching saturation magnetization in a strong magnetic field, the magnetization factor rotates around the nearest field direction and which results in high anisotropy as the field is reduced to zero. The stress and shape of anisotropy are the most important parameters for obtaining the maximum magneto electric output in the composites [Citation31]. By increasing LF phase in composites, increases remnant magnetization due to suppress the stress by applied field, which results in high magnetization.
Saturation magnetization variation over the temperature range for present investigated composites is shown in . Transitions are clearly shown in the figure suggesting the Neel temperature at 330 K (x = 0.05), 440 K (x = 0.10) and 500 K (x = 0.15). shows the clear nature of magnetic phase reduced to zero with increase in temperature. This magnetic phase increases its stability for higher temperature range due to the presence of lithium ferrite grains. Low ferrite content (x = 0.05) showed gradual but systematic transition for the rise in temperature, but higher concentration of ferrite showed abrupt transition, noticed in magnetic phase materials. This variation of magnetization is could be complex interaction between Li ions and Fe ions leading the spin chanting effects in lithium ferrites as reported elsewhere [Citation32 Citation2013;Citation34] and the variations is depends on the nonmagnetic high BTZr content, low LF content.
4 Conclusions
Composite phases of BTZr and LF were confirmed by XRD, exhibits reduced intensity peaks with the major phases for BTZr and rise in minor phases for lithium ferrite in composites. SEM micrograph exhibits higher average grain size, which increases with increase lithium ferrite. Saturation magnetization increases with increase LF suggesting the presence of magnetic phase. Remnant magnetization and coercive field (Hc) of BTZr composites increases with increasing ferrite concentration. Improvement of Curie temperature was evident by the presence of ferrite concentration in BTZr.
Acknowledgements
We thank Dr. P. D. Babu of UGC-DAE Consortium for Scientific Research, Mumbai Centre for extending his PPMS-VSM facility and help in carrying out the magnetization measurements reported in this paper.
Dr. Mukul Gupta UGC-DAE Consortium for Scientific Research, Indore is gratefully acknowledged for extending the facilities XRD.
References
- H.F.ZhangS.W.OrH.L.W.ChanMater. Res. Bull.44200913391346
- A.A.BelikM.AzumaT.SaitoY.ShimakawaM.TakanoChem. Mater.172005269273
- K.SadhanaT.KrishnaveniK.PraveenaS.BharadwajS.R.MurthyScr. Mater.5952008495498
- N.AbdelmoulaH.KhemakhemJ. Alloys Compd.4172006264268
- J.C.BurfootG.W.TaylorPolar Dielectrics and their Applications1979MacmillanLondon
- M.FiebigJ. Phys. D: Appl. Phys.382005R123R152
- N.A.SpaldinM.FiebigScience3092005391392
- K.SadhanaT.KrishnaveniK.PraveenaS.BharadwajS.R.MurthyJ. Alloys Compd.47212009484488
- G.-PingDuZ.-J.HuQ.-FengHanXiao-MeiQinW.-Z.ShiJ. Alloys Compd.4922010L79L81
- C.-W.NanM.I.BichurinS.DongD.ViehlandG.SrinivasanJ. Appl. Phys.1032008031101
- Y.WangJ.HuY.LinC.-W.NanNPG Asia Mater.2220106168
- J.MaJ.HuZ.LiC.-W.NanAdv. Mater.23201110621087
- M.LiuN.X.SunPhil. Trans. R. Soc. A372201420120439
- S.W.YangR.-C.PengT.JiangY.-K.LiuL.FengJ.-J.WangL.-Q.ChenX.-G.LicC.-W.NanAdv. Mater.26201470917095
- H.ZhengJ.WangS.E.LoflandZ.MaL.Mohaddes-ArdabiliT.ZhaoL.Salamanca-RibaS.R.ShindeS.B.OgaleF.BaiD.ViehlandY.JiaD.G.SchlomM.WuttigA.RoytburdR.RameshScience3032004661663
- M.LorenzV.LazenkaP.SchwinkendorfF.BernM.ZieseH.ModarresiA.VolodinM.J.Van BaelK.TemstA.VantommeM.GrundmanJ. Phys. D: Appl. Phys.472014135303135310
- G.Ganapathi RaoK.SamathaS.BharadwajM.P.DasariModern Phys. Lett. B30242016 1650311-10
- R.BottcherH.T.LanghammerT.MullerH.P.AbichtJ. Phys.: Condens. Matter202008 505209-8
- E.MashkinaC.McCammonF.SeifertJ. Solid State Chem.1772004262267
- I.E.GreyC.LiL.M.D.CranswickR.S.RothT.A.VanderahJ. Solid State Chem.1351998312321
- F.T.LinD.M.JiangX.M.MaW.Z.ShiJ. Magn. Magn. Mater.3202008691694
- R.M.GlaisterH.F.KayProc. Phys. Soc.761960763771
- T.TadiY.KimD.SarkarC.KimK.S.RyuJ. Magn. Magn. Mater.3232011564568
- R.S.DevanS.A.LokareD.R.PatilS.S.ChouguleY.D.KolekarB.K.ChouguleJ. Phys. Chem. Solids67200615241530
- M.A.RahmanS.Kumar SahaA.K.M.Akther HossainMater. Res. Express12014026113
- http://imageJ.nih.gov.
- R.S.DevanB.K.ChouguleJ. Appl. Phys.1012007014109
- JyotiRaniK.L.YadavSatyaPrakashMater. Chem. Phys.147201411831190
- Ricardo A.M.GotardoIvair A.SantosLuiz F.CoticaEriton R.BoteroDucineiGarciaJose A.EirasScr. Mater.612009508511
- C.LanY.JiangS.YangJ. Mater. Sci.462011734738
- H.YangyZ.WangyL.SongyJ. Phys. D: Appl. Phys.29199625742578
- P.P.HankareR.P.PatilU.B.SankpalS.D.JadhavI.S.MullaK.M.JadhavB.K.ChouguleJ. Magn. Magn. Mater.321200932703273
- M.KumarS.ShankarG.D.DwivediA.AnshulO.P.ThakurAnup K.GhoshAppl. Phys. Lett.1062015072903
- J.L.DormannA.TomasM.NoguesPhys. Status Solidi A771983611618