Abstract
This study focus on specifying the roles of solvent ethylene glycol (EG) and polymers for synthesis of uniformly distributed magnesium oxide (MgO) nanoparticles with average crystallite size of around 50 nm through a modified polyol method. Based on different characterization results, it was concluded that, Mg2+ ions was precipitated by the −OH and CO32− ions decomposed from urea in ethylene glycol (EG) medium (CO(NH2)2 → NH3 + HNCO, HNCO + H2O → NH3 + CO2), thus forming well crystallized Mg5(CO3)4(OH)2 (H2O)4 precursor which could be converted to MgO by calcination. Surface protectors PEG and PVP have no obvious influences on cyrtsal structure, morphology and size uniformity of as-prepared precursors and target MgO nanoparticles. In comparison with polymers PEG and PVP, solvent EG plays an important role in controlling the morphology and diameter uniformity of MgO nanoparticles.
1 Introduction
Benefiting from the largely increased specific surface area and extremely great proportion of low-coordinated surface sites, nano-scaled magnesium oxide (MgO) is commonly regarded as one of the ideal candidates as absorbents, catalysts and refractory materials and so forth [Citation1 Citation2013;Citation3]. However, these potential applications of MgO nanoparticles are always inhibited by its large aggregations and conglutinations due to its large surface energy [Citation4 Citation2013;Citation7]. Therefore, synthesis of MgO nanoparticles with little agglomerations and good diameter uniformity is still one of the important challenges we are facing now to make its different potential applications valid.
Usually, nano-structured MgO particles are prepared by chemical vapor deposition (CVD), sol–gel, hydrothermal, microwave, and precipitation and so on [Citation8,Citation9]. In addition, it is demonstrated that the reaction medium such as benzyl alcohol and ethanol and so on largely affect the shape and size of MgO particles. Accordingly, due to the largely diminished hydrogen bandings between as-prepared particles, utilization of non-aqueous solvent as solvent endows the particles enhanced diameter uniformity [Citation10 Citation2013;Citation12]. To date, Selvamani et al. synthesized MgO nano-sheets through hydrolysis of MgCl2 with urea aqueous solution in ethylene glycol (EG) medium [Citation13]. MgO with big flower petals was also synthesized by EG-mediated solvothermal method [Citation14]. According to their reports, EG plays an important role in controlling the morphology and size of MgO nanoparticles. However, these methods are still too complicated and time consuming to effectively control the diameter uniformity of MgO nanoparticles. Then, it is wondered if it is possible to develop a much more facile method for preparing uniformly distributed MgO nanoparticles or not.
Fortunately, polyol method meets this emergency demand. Besides Ag, Pt and Au nanoparticles, kinds of metal coxides such as CeO2, TiO2 and BaTiO3 and so on were also synthesized [Citation15 Citation2013;Citation18]. According to these reports, there are two viewpoints for controlling the diameter uniformity of nanoparticles via polyol method. Some researchers believe that, benefiting from the enhanced stereo-hindrance effects induced from the surface protectors such as polyvinylpyrrolidone (PVP), polyvinyl alcohol (PVA), polyethylene glycol (PEG) etc., fine particles with diameter uniformity could be effectively synthesized. While some researchers persisted in that the solvent EG acting as surfactant resolves nanoparticles diameter uniformity controlling difficulty. Nevertheless, up to now, no systematic study concerning the specific influence of them on particles has been published yet. Herein, in this study, taking these factors into consideration, we primarily synthesized MgO nanoparticles via a modified polyol method employing EG as solvent. In order to well understand the formation mechanism, through monitoring the crystal structure and morphology evolutions of precursors during the reaction, roles of EG solvent and surface protectors such as PVP, PEG were experimentally confirmed, respectively.
2 Experimental
2.1 Synthesis of MgO nanoparticles
2.1.1 Using EG as solvent without surface protectors
Firstly, 0.05 mol MgCl2·6H2O and 0.2 mol CO(NH2)2 were dissolved in 100 ml EG under constant stirring at room temperature and heated at 110 °C for 8 h. Secondly, after heating, as-precipitated white precursor was thoroughly washed and dried in oven at 80 °C for 12 h. Finally, as-prepared precursor was calcinated at 400 °C, 500 °C, 600 °C, 700 °C and 800 °C for 3 h, respectively. In order to investigate the role of EG in reaction, the solvent EG was replaced by the same ratio of distilled water. This reaction was performed at 90 °C for 8 h. For comparison, as-prepared precursor in water solvent was consequently calcinated at 700 °C for 3 h to get well crystallized MgO particles.
2.1.2 Effect of surface protectors PVP and PEG
In order to verify the role of polymers for controlling the dispersibility of MgO nanoparticles via polyol method, 2.0 g of PVP (Mw = 10,000, Aldrich) and PEG (Mw = 10,000, Aldrich) were individually added into the same reaction solution and followed by reaction at 110 °C for 8 h. As-prepared precursors were calcinated at 700 °C for 3 h to get target material MgO nanoparticles.
For comparison, the precursors obtained in EG solvent without PVP and PEG, in water without PVP and PEG and in EG solvent with PVP and PEG were denoted as p1, p2, p3-PVP and p3-PEG, respectively. Correspondingly, the MgO particles prepared by calcination of these different precursors as mentioned above were named as MgO1, MgO2, MgO3-PVP and MgO3-PEG.
2.2 Characterizations
Structures and components of specimens were evaluated by X-ray diffraction (XRD) technique on X’pert Pro X-ray diffractometer (Panalytical Company in Holland). Morphology and size of samples were observed by field emission-scanning electron microscopy (FE-SEM, Su8010, Hitachi, Japan) and High resolution TEM (HRTEM, Tecnai G2, FEI). Selected area electron diffraction (SAED) technique was employed to analyze the relationship between morphology and crystal structure on the same instrument with HRTEM. Fourier transform infrared spectroscopy (FT-IR, Nexus, Thermo Nicolet, USA) was used to identify the functional groups on the surface of samples. Thermal analysis was conducted on TG/DTA (TA instrument, SDT Q600, American).
3 Results and discussion
3.1 Characterizations of precursor and MgO particles prepared in EG solvent
XRD pattern of p1 is shown in (a). All peaks can be indexed to the monoclinic crystalline phase of Mg5(CO3)4(OH)2(H2O)4 (JCPDS No.: 70-0361). From this pattern, it is no doubt to conclude that the Mg2+ ions could be initially precipitated as Mg5(CO3)4(OH)2(H2O)4 via this method.
Fig. 1 (a) XRD pattern and (b) TG/DTA curves of p1, (c) XRD patterns of calcinated precursor1 at different temperatures for 3 h and (d) FT-IR spectra of p1 before/after calcinations at 700 °C for 3 h.
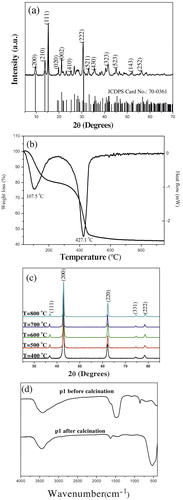
The TG/DTA measurement was conducted in air atmosphere from ambient temperature to 700 °C to analyze the thermal behavior and decomposition performance of as-prepared p1 ( (b)). In TG curve, the marked weight loss 19.71% in the range of 77.4 °C–250 °C is briefly attributed to the physically absorbed water and crystalline water. And the weight loss 37.35% in the range of 250 °C–550 °C is assigned to the decarboxylation of Mg5(CO3)4(OH)2(H2O)4 [Citation13,Citation19]. The overall weight loss between room temperature and 700 °C is around 57.06%, which is in line with the theoretical value 57.08% calculated from the complete decomposition of Mg5(CO3)4(OH)2(H2O)4 precursor1 (Mg5(CO3)4(OH)2 (H2O)4 = 5MgO + 4CO2↑ + 5H2O↑) [Citation12,Citation20]. As seen from the DTA curve of p1 in (b), a broad endothermic peak centered at 107.5 °C results from the desorption of water. Another endothermic peak at 427.1 °C comes from the decarboxylation process during calcination. Thereby, it is no suscipious to conclude that the p1 completes its thermal decomposition around 550 °C.
XRD patterns of the p1 calcinated at 400 °C, 500 °C, 600 °C, 700 °C and 800 °C for 3 h respectively, are shown in (c). Diffraction peaks at 2θ = 36.92°, 42.94°, 62.29°, 74.69° and 78.62° corresponding to the (111), (200), (220), (331) and (222) planes of MgO confirm its face-centered cubic structure (JCPDS No.: 77-2179) [Citation10,Citation11,Citation19]. Particularly, these broadened typical diffraction peaks support that as-prepared MgO1 are of nanometer [Citation21]. Moreover, no characteristic peaks of other impurities are identified in these patterns, which indicate that as-prepared MgO1 particles has high purity [Citation21,Citation22]. Because there is no distinctive difference between the MgO1 samples obtained at 700 and 800 °C, so the optimum calcination temperature for p1 is 700 °C.
(d) shows the FT-IR spectra of p1 before/after calcination at 700 °C for 3 h. Two peaks of these two samples p1 and MgO1 at 3432 and 1643 cm−1, are assigned to the hydroxyl stretching and bending vibrations, respectively. Here the −OH is from the physically adsorbed water and surface hydroxyl groups strongly perturbed by hydrogen bonding [Citation22]. The peak at 543 cm−1 in p1 is assigned to the stretching vibration of Mg–O band. The peak at 696 cm−1 corresponds to the in-plane deformation of C–O. The peak at 854 cm−1 corresponds to the CO3 out-of plane deformation. While the sharp two peaks at 1483 and 1096 cm−1 are attributed to the asymmetric and symmetric stretching vibrations of C–O band, respectively [Citation23,Citation24]. After calcination, the disappeared typical characteristic peaks of CO32− around 696, 854, 1096 and 1483 cm−1 confirm the decomposition of CO32− via calcination, which is in well consistent with the XRD and TG/DTA analysis results. Moreover, the weak peak at 1483 cm−1 in as-prepared MgO1 indicates the existence of surface chemisorbed atmospheric CO2, indicating its acid-based property [Citation13]. MgO1 has a broad band at 3432 cm−1, which implies the presence of four coordinated hydroxyl groups interacting with the low-coordinated surface ions (OLC2− ions) [Citation25].
shows the FE-SEM and HRTEM images of p1 and MgO1. From (a) and (b), it is evident that as-prepared MgO1 nanoparticles calcinated at 700 °C inherits the sphere-like morphology from its precursor p1. Usually, the preferred shape of Mg5(CO3)4(OH)2(H2O)4 is sheet-like as reported [Citation10,Citation13]. Here the sphere-like precursor maybe benefit from the EG medium which largely diminishes the absorbing possibility of OH− on the surface of precursor surface [Citation10]. (b) and (c) confirms that the highly dispersed MgO1 nanoparticles with uniform particle size about 50 nm are prepared via this modified polyol method. HRTEM image ((d)) and SEAD inserted in (d) confirm that as-prepared MgO1 nanoparticles are of fcc structure, and the resolved lattice spacing of approximately 0.217 nm corresponds to the (200) planes of MgO1, which supports their preferred growth along (100). The SEAD diffraction rings from inner to outside, supporting the polycrystalline nature of MgO1, can be identified to (111), (200), (220), respectively [Citation8,Citation10,Citation11,Citation19,Citation26]. This is associated with that obtained from the XRD result in (c). Consequently, this modified polyol method is a suitable method for preparing MgO nanoparticles. Besides that, what is the more important to point out is that, Selvamani et al. has prepared two-dimensional Mg5(CO3)4(OH)2(H2O)4 and MgO nanosheets in EG and water mixture [Citation13]. The only difference between their and our study is that utilization of water or not, which indicates that water plays an important role in controlling the morphology of specimens.
3.2 Influence of solvent
Once changing the solvent EG as the same amount of distilled water, as shown in (a) (patterns a and b are the diffraction patterns of p2 before and after calcination at 700 °C for 3 h, respectively), the observed diffraction peaks at 2θ = 18.5°, 32.9°, 37.9°, 50.8° and 58.7° are indexed to the (001), (100), (101), (102) and (110) planes of Mg(OH)2 (JCPDS card: No.:44-1482) with hexagonal crystal phase. After calcintation at 700 °C for 3 h, as eplicted in (a) pattern b, because of the appearence of the characteristic peaks of MgO, thus it is no doubt to conclude that even though the precursors are totally different, final samples are MgO. As revealed by the FE-SEM images of p2 and MgO2 in (b) and (c), both p2 and MgO2 are largely agglomerated, which mainly results from the hydrogen bands among these particles. Based on these characterization results, consequently, it is concluded that, comparing with distilled water, EG plays an important role in controlling the size uniformity of target material MgO.
3.3 Influence of surface protectors
On the above discussions, it is concluded that the solvent EG plays an important role in decreasing the agglomeration degree of samples. However, researchers disputes with each other on the role of solvent and polymer in polyol method for controlling the dispersibility and size uniformity of different nanoparticles. Normally, not only PEG, but also PVP is widely utilized as surfactant in different synthesize processes [Citation16,Citation17]. discloses the influences of PEG and PVP on crystal structure of precursor before/after calcination at 700 °C for 3 h. Comparing with the p1 diffraction pattern ((a)), from (a), appearence of a broad diffraction peak centered around 2θ = 25° indicates the inhibited crystallization process of p3 which were prepared by adding PEG and PVP into the EG-based reaction system, respectively. After calcination at 700 °C for 3 h, as shown in (b), p3 were totally converted to MgO nanoparticles which was evidenced by the characteristic peaks of MgO at 2θ = 36.92°, 42.94°, 62.29°, 74.69° and 78.62°, respectively. Therefore, it is no doubt to conclude that, by adding polymers in EG solvent, MgO particles could be prepared.
Fig. 4 XRD patterns of p3 with different polymers (a) before and (b) after calcation at 700 °C for 3 h.
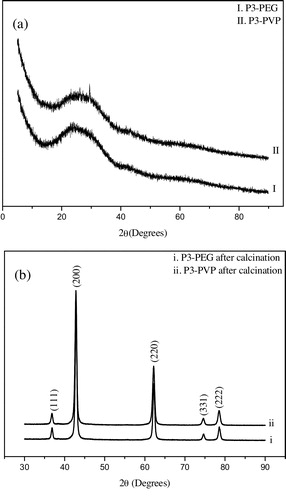
As confirmed in , after calcination at 700 °C for 3 h, sphere-like p3-PEG and p3-PVP were converted to well-crystallized MgO3-PEG (DAV = 50 nm) and MgO3-PVP (DAV = 40 nm) nanoparticles, respectively. However, comparing with that of MgO1 ((b)), MgO-PEG has some large agglomeration. And the diameter uniformity differences of MgO nanoparticles (MgO1, MgO3-PVP) are not distinctive. Therefore, even though it is widely regarded that the dispersibility and uniformity of nanoparticles could be resolved by polymer via a stereo-hindrance effect, it is not valid in this study.
3.4 Formation mechanism
Based on the characterization and discussion results above, we can successfully prepared uniformly dispersed MgO nanoparticles via a modified polyol method without adding any kinds of surfactants or surface protectors. Solvent EG plays an important role in decreasing the agglomeration degree and improving the size uniformity of MgO nanoparticles. What is more important to notice is that, in order to precipitate Mg2+ ions as Mg5(CO3)4(OH)2(H2O)4 precursor, it is necessary to supply the sufficient –OH and CO32− ions in reaction solution. Because both the changes of –OH and CO32− concentrations could be monitored by pH meter, thus it is possible to conclude the formation mechanism of precursor in this method through monitoring the change of pH in reaction solution.
Variation curves of pH and real temperature as a function of heating time are depicted in . This reaction system achieves 110 °C in 125 min and changes opaque around 105 °C. After reaching the set temperature 110 °C, specimens were extracted every 1 h. During the heating period, the solution pH decreases from 8.25 to 6.62. More, on heating to 90 °C, there is a pH fluctuation. The pH decreasing and fluctuation may well be attributed to the decomposition of urea. Accordingly, at the initial stage (20 °C–90 °C), urea was decomposed to HNCO (CO(NH2)2 → NH3 + HNCO), leaving the decreased pH. While from 90 °C to 105 °C, hydrolysis of cyanic acid (HNCO + H2O → NH3 + CO2) leads to pH increase [Citation27]. To date, even though numerous literatures about urea decomposition in aqueous solution are available, few reports are about urea decomposition performance in EG medium [Citation8,Citation9,Citation11,Citation28]. In our study, the crystal water in MgCl2·6H2O supplies the precursor nucleation condition (stage a and b in (a)). In situ decomposition of urea in EG homogeneously releases precipitate ligands OH− and CO32− ions and immediately reacts with Mg2+, forming Mg5(CO3)4(OH)2(H2O)4 precursor.
Fig. 6 (a) Changes of pH and real temperature as a function of heating time, and (b) XRD patterns of extracted samples for different reaction periods at 110 °C.
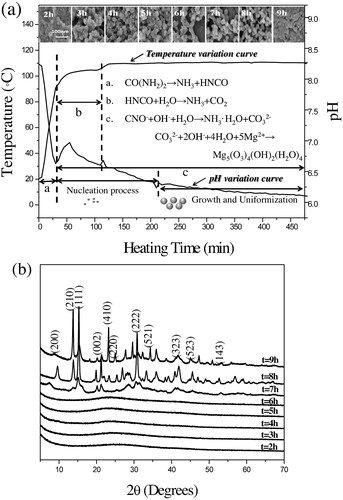
Utilization of solvent EG prefers the formation of spherical precursor Mg5(CO3)4(OH)2(H2O)4 nanoparticles to the sheet-like one, which mainly results from the largely reduced amount of chemisorbed water (stage c in (c) and (b), FE-SEM images of extracted specimens). More, EG only act as reaction solvent, but also plays as surfactant, thus forming uniformly distributed sphere-like MgO nanoparticles. This conclusion is evidenced by the former studies, who synthesized sheet-like MgO nanoparticles by using the mixture of urea aqueous and EG solution or pure water [Citation10,Citation11,Citation13]. More, as shown in (b), during the reaction, although there is little change of morphology, as-prepared precursor starts to crystallize and has higher yields once the reaction time is longer than 7 h. Consequently, according to the characterization results as depicted in , it is facilitated to conclude that the reaction at 110 °C for 8 h is the optimum precipitation condition.
4 Conclusions
In conclusion, spherical MgO nanoparticles without large agglomerations were successfully synthesized by a modified polyol method without adding any other surfacants or surface protectors. Benefiting from the decomposition of urea in EG solvent, Mg2+ ions could be precipitated as uniformly dispersed spherical Mg5(CO3)4(OH)2(H2O)4 precursor. By individually investigating the influences of solvent and polymer PEG and PVP, it is summarized that EG not only acts as solvent, but also plays as surfactant for preparing uniformly distributed sphere-like MgO nanoparticles.
Acknowledgments
This work is financially supported by NSFC (24101210), CAS “light of West China” program, Youth innovation promotion association CAS (2016376), Qinghai Science & Technology Projects (2016-GX-102) and CAS “light of West China” program and the Hundred-Talent Program (Chinese academy of Sciences).
References
- S.HanY.BangH.LeeChem. Eng. J.2702015411417
- S.RackauskasH.JiangJ.B.WagnerS.D.ShandakovT.W.HansenE.I.KauppinenA.G.NasibulinNano Lett.14201458105813
- D.KoziejA.LauriaM.NiederbergerAdv. Mater.262014235256
- B.Z.FangJ.H.KimM.KimJ.S.YuChem. Mater.212009789796
- J.H.LeeSensor Actuators B1402009319336
- Y.F.ZhaoM.WeiJ.LuZ.L.WangX.DuanACS Nano3200940094016
- F.MeshkaniM.RezaeiPowder Technol.1992010144148
- N.C.S.SelvamR.T.KumarL.J.KenndyJ.J.VijayaJ. Alloys Compd.509201198099815
- N.SutradharA.SinhamahapatraB.RoyH.C.BajajI.MukhopadhyayA.B.PandaMater. Res. Bull.46201121632167
- K.K.ZhuJ.C.HuC.KübelR.RichardsAngew. Chem. Int. Ed.45200672777281
- K.ZhuW.HuaW.DengR.M.RichardsEur. J. Inorg. Chem.17201228692876
- A.KumarJ.KumarJ. Phys. Chem. Solids69200827642772
- T.SelvamaniA.SinhamahapatraD.BhattacharjyaI.MukhopadhyayMater. Chem. Phys.1292011853861
- Z.CuiZ.ChenC.CaoW.SongL.JiangChem. Commun.49201360936095
- Y.SunB.GatesB.MayersY.XiaNano Lett.22002165168
- C.HaiK.InukaiY.TakahashiN.IzuT.AkamatsuT.ItohW.ShinMater. Res. Bull.572014103109
- H.B.MaoJ.Y.FengX.MaJ. Nanopart. Res.142012887902
- Y.G.SunB.MayersT.HerricksNano Lett.72003955960
- A.R.BuenoR.F.M.OmanP.M.JardimMicroporous Mesoporous Mater.18520148691
- K.SasakiS.MoriyamaCeram. Int.40201416511660
- A.L.PattersonPhys. Rev.561939978982
- K.MageshwariS.S.MaliR.SathyamoorthyP.S.PatilPowder Technol.2492013456462
- T.KornprobstJ.PlankJ. Non-cryst. Solids3612013100105
- W.BrackB.HeineF.BirkholdM.KruseG.SchochS.TischerO.DeutschmannChem. Eng. Sci.106201418
- M.LiW.GuoH.LiW.DaiB.YangSensor Actuators B Chem.2042014629636
- S.XieX.HanQ.KuangY.ZhaoZ.XieL.ZhengJ. Mater. Chem.21201172637268
- P.WangS.LiuF.ZhouB.YangA.S.AlshammariL.LuY.DengFuel Process. Technol.1262014359365
- H.QinX.TanW.HuangJ.JiangH.JiangCeram. Int.4120151159811604