Abstract
Cordierite ceramic membranes were manufactured from natural clay, oxides and organic wastes as pore forming agents. Mixtures aforementioned materials with the pore-forming agents (up to 10 wt.%) were investigated in the range 1000–1200 °C using thermal analysis, X-ray diffraction, scanning electron microscopy, mercury porosimetry and filtration tests. Physical properties (density, water absorption and bending strength) were correlated to the processing factors (pore-forming agent addition, firing temperature and soaking time). The results showed that cordierite together with spinel, diopside and clinoenstatite neoformed. SEM analysis revealed heterogeneous aspects. The results of the response surface methodology showed that the variations of physical properties versus processing parameters were well described by the used polynomial model. The addition of pore forming agent and temperature were the most influential factors. Filtration tests were performed on the best performing sample. The results allowed to testify that these membranes could be used in waste water treatment.
1 Introduction
Recently, with concentration on the environmental aspect and increasing its demands, porous ceramic materials are extensively studied for their great advantages [Citation1 Citation2013;Citation3] such as surface area, thermal and electrical conductivity, and resistance to both severe chemical environments and high temperatures [Citation4]. They are used in a wide range of applications, namely: sensors [Citation5], catalytic support [Citation6], bio-ceramics [Citation7] and of course filtration of fluids [Citation8]. Beyond the underlying properties for which the porous ceramics are used, there is the economic aspect whose ceramics offer advantages over their metallic or polymeric counterparts.
The filtration membranes are among the abovementioned porous ceramics distinguished materials. These are defined as a perm-selective barrier which will reduce the transfer of a solute relative to another (usually one or solutes relative to water) [9,10]. There are three types of membrane filtration according to the mode used (pore diameter): micro-filtration, ultra-filtration and nano-filtration [Citation11]. Normally, a combination of different pore types (size and morphology) in a designed matrix could be expected to extend the properties of porous ceramics and subsequently outspread the application fields [Citation12]. Because of the environmental interests, membranes are increasingly used mainly in the treatment of pollutants. To overcome these problems, many scientific works have been devoted to the research of new ceramic membranes with high performances and low costs in order to treat large amounts of effluents.
The cordierite (ideal chemical formula: Al3Mg2AlSi5O18) occupies a special place among these ceramics because of its remarkable properties: thermal and chemical stability, thermo-mechanical resistance as well as low thermal expansion properties and dielectric constant [Citation13 Citation2013;Citation19]. Cordierite owes most of these properties to the negative lattice expansion coefficient in the c-axis direction, which provides excellent resistance to thermal shock as well as low thermal conductivity. This makes it suitable to be applied in environments where the temperature is rapidly and severely changed such as refractory products for industrial furnaces and electric heaters [Citation20], heat exchangers for gas turbines, thermal shock-resistant tableware, and monolithic catalyst supports for diesel cars [Citation11]. Porous cordierite has been also investigated as membrane filters and/or membrane supports in some works [Citation21 Citation2013;Citation24]. However, no elaborate and comprehensive research has been done to prepare porous cordierite filters. The use of cordierite in this field will lead to a technological advancement that will give a value added to natural mineral that exists all over the world and which are not enough or well used [Citation25].
The manufacture of porous ceramic materials requires the use of pore-forming agents (temperature-sensitive components) for the pore-creation during heat treatment. These additives thermally decompose generating a network of pores which is supplementary to that formed by the matrix itself. Several pore generators have been reported in the previous works such as micro-cellulose described by Rat’ko et al. [Citation26], and flower employed by Ivanets [Citation27,Citation28], starch used by Lorente-Ayza et al. [Citation29,Citation30] and sawdust related by Bose and Das [Citation31]. The most widely used in this field are sawdust and starch as they decompose readily at around 600 °C, cheap and environmentally friendly. This being said, as materials derived from organic matter subjected to different treatments throughout the manufacturing process, they have a wide range of properties which can affect the resulting membranes.
The aim of this study is to investigate the feasibility of porous cordierite membrane manufacturing. The technological properties were discussed according to the processing factors. These membranes are planned to be used for waste water treatment.
2 Materials and experimental techniques
2.1 Materials
A naturally occurring clay (stevensite clay) (GH) extracted from a local clay deposit was chosen as main material for the cordierite phase synthesis together with aluminum hydroxide and silica gel (commercial) to have the favorable stoichiometry (5 SiO2, 2 Al2O3, 2 MgO) for the formation of this phase. and give the chemical and the mineralogical composition of GH.
Table 1 Chemical and mineralogical compositions (wt.%) of the raw clay.
Organic wastes (sawdust, starch and ion exchanger resin) were used in this study as pore forming agents. Sawdust was supplied by a local carpentry factory while starch and ion exchanger resin were recovered from the laboratory. All the raw materials used were sieved through a 100 μm sieve. Some properties of pore-forming agents are shown in Figs. (granulometry) and (a and b) (microstructure).
2.2 Experimental procedure
Blends of dried clay, aluminum hydroxide and silica gel were homogenously mixed in stoichiometric amounts. The mixtures were heated for 2 h at 1230 °C, at a heating rate of 10 °C/min, in an electric furnace (Nabertherm), and then cooled to room temperature in the switched off furnace. The so obtained powders were well grounded in an agate mortar and were amended by the above-mentioned organic additives (up to 10 wt.%). Cylindrical specimens ((D = 40 mm, h = 3 mm) for filtration tests and (D = 40 mm, h = 3 mm) for mechanical properties) were shaped in a cylindrical mold by uniaxial pressing and fired in the range [1000–1200 °C] for 1–4 h at 10 °C/min in order to harden the ceramic bodies and form pores by decomposition of the added organic matters. Three mixtures were obtained: sawdust containing blends (BSB), mixtures within resin (BR) and starch containing blends (BAM).
2.3 Techniques
Phase identification was performed by X-ray diffraction analysis using a Philips X’Pert MPD diffractometer operating with a copper anode (Kα = 1.5418 Å). Scanning electron microscopy (SEM) observations were carried out on gold coated shards using a JEOL JSM-5500 apparatus equipped with an EDS detector. Thermal curves (DTA-TG) were obtained using a Setaram Setsys 24 apparatus functioning in air at 10 °C/min. Mercury porosimetry, used to assess the porosity of samples, was performed with a Quantachrome porosimeter PoreMaster. Water absorption was estimated by the difference of the weight between the heated samples and water saturated ones (immersion in boiled water for 2 h) [Citation32]. Density values were determined using a pycnometer. The compressive strength was performed with an Instron 3369 apparatus. The load and loading used speed were 50 kN and 0.1 mm/min, respectively. For this trial, five heated samples were studied. Filtration tests were conducted on a laboratory microfiltration pilot [Citation33].
2.4 Modeling
The effects of processing factors (additive amount (τ), firing temperature (T) and soaking time (t)) were assessed using the following equation [Citation34,Citation35]:where: X1, X2 and X3 are the coded variables corresponding to τ, T and t respectively and k is the number of factors.
τ0, T0 and t0 are the sawdust addition, firing temperature and soaking time at the centers of the experimental range (τ0 = 12.5 wt.%, T0 = 1000 °C and t0 = 2.5 h). Δt, ΔT and Δt are the variation steps of the considered variables (Δτ = 7.5 mass%, ΔT = 100 °C and Δt = 1.5 h). b0 is a constant, and b1, b2 and b3 are the weights of the effects of sawdust addition, temperature and soaking time respectively. bij express the weight of the interactions effect between i and j factors, and aii is considered as curve shape parameter. The coefficients were calculated by the software Nemrod using the least-squares regression [Citation36,Citation37]. For this purpose, multiple experiments (16) were performed according to the Dohlert matrix. The test at the center was multiplied (repeated 3 times) in order to minimize the experimental error. The experiments proposed by the model and the experimental values of physical properties are shown in . The accuracy and validity of the used model was confirmed by the analysis of variance (ANOVA) [Citation38].
Table 2 Experimental design matrix (Doehlert) and measured values of the studied properties.
3 Result and discussion
3.1 Thermal behavior and microstructural investigations
X- ray diffraction pattern of the heated materials (A & B) showed that dolomite together with stevensite collapsed likely at the first thermal cycle whose purpose was to form cordierite powders. In fact, cordierite together with diopside, spinel and clinoenstatite neoformed but in slightly different proportions for each mixture. Therefore, It can be inferred that the solid-state reaction leading to cordierite formation also favored the development of the two supplementary phases mentioned above. As reported from previous works [Citation39], spinel phase likely resulted from the incomplete reaction between Al2O3 and MgO. As far as the X-ray diffraction was concerned no vitreous phase was in the heated materials.
Fig. 4 X-ray diffraction patterns of blends heated at 1000 °C.
(A) BAM; (B): (b) BSB; (c) BR.
C: cordierite (PDF # 84-1222); S: spinel (PDF # 41-1370); d: diaposide (PDF # 77-0437); L: clinoenstatite (PDF # 13-0415).
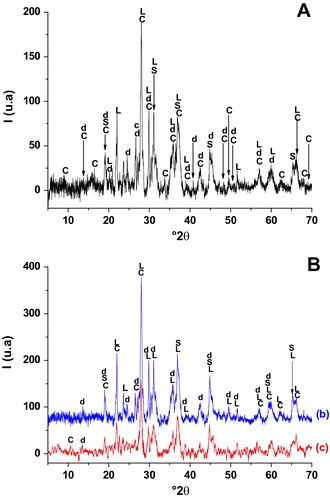
In order to highlight phases transition phenomena, identify the decomposition processes and the resulting products thermal analysis (DTA) was performed and the corresponding results are displayed in . The results showed that both BAM and BSB mixtures (A) experienced two transitions at 106 and 402 °C corresponding to hygroscopic water loss [Citation40,Citation41] and organic matter decomposition respectively [Citation42]. The second endothermic peak involved a marked loss of weight (TG curve—not given) which can be associated with carbon dioxide release [Citation43]. TDA curves revealed that negligibleamount of organic phases remained after the heat treatment. Regarding BR blends (B), samples have undergone five transformations at 112, 445, 472, 524 and 603 °C. First endothermic phenomena was assigned to dehydration (Water departure) and the others to the resin decomposition in various stages.
displayed the termo-dilatometric behavior or the studied blends. It is worth noting that most contraction (both shrinkage and expansion) were localized in the ranges 100–200 °C, 400–600 °C and 850–1050 °C. The shrinkage located in the first range was related to hydration water while the second corresponded to the additive decomposition. The last one positioned at high temperatures was attributed to the neoformation process. The difference in responses of the considered mixtures can be explained by the behavior of each additive in the pore formation and consequently in the sintering process. These results are in agreement with those of thermal analysis.
The SEM observations were carried out on a typical fracture surface of the manufactured membranes. It revealed that the samples present a granular structure with different shape and size (a). Clusters of about 0.55 μm were scattered among the matrix (b). The so-called clusters were composed of SiO2 and MgO with a mass ratio around 0.44 wt.% leading probably to the formation of the “clinoenstatite” (a & b). These outcomes confirmed the results obtained from the X-ray pattern. In regards to SEM analysis, the magnesium rich aluminosilicates are not discarded. As a result of heating most particles coalesced and started to melt (a). Also, molten zones apeared all along the sample’s bodies. At the same time, mass of cellular grains took place (b). In addition, the mineralization of what appears to be a Mg-calc-aluminosilicate phase was observed. This latter seems probably originated from the decomposition of dolomite and a portion of clay minerals. Such contestations are in conjunction with the data of the diffraction X-ray.
3.2 Porosity measurement and application to the filtration of textile waste water
Cumulative porosity percentage and incremental porosity relevant to the studied mixtures are displayed in . As can be seen, both BSB and BR blends exhibited a multimodal distribution of pore size (more than two different peaks or overlapped ones) (a & b), while BAM mixtures showed a bimodal one (two diverse or overlapping peaks) (c). Such note means the existence of several categories of pore size distribution.
Referring once again to , all mixtures consisted of macro- and mesopores (BSB: 0.09, 0.4, and 3.8 μm; BR: 0.08, 0.6, and 3.5 μm; BAM: 0.08 and 3.3 μm) and showed extended distribution of macropores, as observed from the above mentioned figures. The appearance of bi- and multimodal distribution suggested the existence of two different pores sources. Indeed, several studies have reported that carbonates contribute to the formation of pores during their decomposition at average temperatures (800–1000 °C). These results agreed with the fact that the organic additive together with dolomite acted simultaneously in the pore formation process. It should be noted that the emergence of these pore-populations can facilitate the membrane resistance against mass transfer and thereby improve the ability of the membrane.
a showed classic filtration experiments for the studied membranes. The material flow becomes stable after 40 min suggesting that the flow no longer passes easily through the membrane. This behavior may be related to the phenomenon of clogging of the membrane after the passage of a large quantity of material through it. Changes of the distilled water flux versus the pressure membrane are displayed in b. A linear course of the water flow through the membrane is noticed and it increased with increasing pressure. Taking into consideration the slopes of the lines of trend curves, the values of the membranes permeability are reported in . These values showed that the water flux depended on the applied pressure, i.e: the water flux increased with the increase of applied pressure.
Table 3 Linear fitting of water flux plots versus trans-membrane pressure.
3.3 Effects of experimental factors on the physical properties of the studied membranes
This part of the study will be devoted to the starch containing mixture.
Analysis of ANNOVA () and plots of measured values versus calculated ones (not given) were used to confirm the validity and precision of the model. As can be seen, the results pointed out that Fisher-ratio was well above 1 (F-ratio ≫ 1) and the signification surpassed 99.9%. Furthermore, values of standard deviation (SD) were very low and R2 values approached unity. These results confirmed that the adopted polynomial model described well the variation of the studied properties against the experimental factors.
Table 4 Analysis of variance (ANOVA) and values of correlation coefficient (R2) for considered responses.
The resulted equations for density (Y1), water absorption (Y2) and compressive strength (Y3) are reported hereafter:
Taking into consideration values of linear coefficients shown in the above equations, the weight of the effects of the considered parameters followed the order: t > τ > T for density, τ > T > t for water absorption and T > τ > t for compressive strength. Moreover, the increase of temperature had a positive impact (↑Y1 & ↑Y3) on density and compressive strength while it reduced the water absorption. The increment of starch addition and soaking time had a negative effect on both density and compressive strength whereas it affected positively the water absorption. Indeed, the increase of temperature favored the melt formation and its development together with the diffusion process leading to the matrix consolidation [Citation44,Citation45]. The negative impact of starch addition is probably due to the pore forming process whereas the effect of time might be linked to the conjugated neoformation and vitrification process.
The analysis of together with the abovementioned equations showed that the effects of the interactions between the factors were sizable. In this regard, it is noteworthy that the simultaneous increase of the starch addition and temperature had considerable antagonistic effect on the density, probably because of the multiplicity of pores during the heat treatment. The negative effect of these factors on density is well displayed by the response surface of a. On the other hand, τ and t manifested synergistic effect in the case of water absorption, i.e., the simultaneous increase of these two parameters leaded to the increase of water absorption (b'). As for compressive strength, τ and T, T and t exhibited synergistic effects also. These effects can be visualized from a and c.
4 Conclusion
The study of the characterization of new ceramic membranes based on cordierite powders obtained from stevensite clay and pore forming agents, allowed to conclude that: (i) Cordierite, spinel, diopside and clinoenstatite neoformed in all mixtures with different amount for each one. Spinel derived from the incomplete reaction between Al2O3 and MgO while diopside and clinoenstatite formed from the breakdown products of dolomite and clay mineral (stevensite). (ii) SEM micrographs exhibited heterogeneous aspects. (iii) Added organic watse played the role of a pore forming agent even with an amount as low as 2 wt.%. iv) It was possible to manufacture cordierite ceramic membranes and they should find application for economic treatment of wastewater. (v) The use of the design of experiments with the response surface methodology, allowed to assess the effects of the processing parameters on the considered properties of membranes. Temperature and starch addition were the most influential factors. The increase of temperature had a positive effect on the mechanical property (compressive strength) while the growth of the starch addition reduced the mechanical resistance of the samples. Soaking time had a mitigated effect.
References
- S.MasmoudiR.Ben AmarA.LarbotH.El FekiA.B.SalahL.CotJ. Membr. Sci.247200519
- S.KhemakhemR.Ben AmarA.LarbotDesalination2062007210214
- S.KhemakhemA.LarbotR.Ben AmarCeram. Int.3520095561
- I.NettleshipKey Eng. Mater.122–1241996305324
- H.J.M.BouwmeesterA.J.BurgraafA.J.BurgraafL.CotJ. Membr. Sci.127199712
- Z.R.IsmagilovR.A.ShkrabinaN.A.KoryabkinaA.A.KirchanovH.VeringaP.PexReact. Kinet. Catal. Lett.601997225231
- N.Ozgür EnginA.C.TasJ. Eur. Ceram. Soc.19199925692572
- Y.M.JoR.B.HutchisonJ.A.RaperPowder Technol.9119975562
- L.MessaoudiA.LarbotM.RafiqL.CotInd. Céram. Verrière1995831835
- Y.DongX.FengD.DongS.WangJ.YangJ.GaoX.LiuG.MengJ. Membr. Sci.30420076575
- X.MaoS.WangS.ShimaiCeram. Int.342008107112
- J.TakahashiM.NatsuisakaS.ShimadaJ. Eur. Ceram. Soc.222002479485
- C.-M.HungPowder Technol.20020107883
- J.A.LabrinchaC.M.AlbuquerqueJ.M.FerreiraM.J.RibeiroJ. Eur. Ceram. Soc.262006825830
- M.J.RibeiroS.BlackburnJ.M.FerreiraJ.A.LabrinchaJ. Eur. Ceram. Soc.262006817823
- D.NjoyaA.ElimbiD.FouejioM.HajjajiJ. Build. Eng.8201699106
- I.M.LachmanR.D.BagleyR.M.LewisAm. Ceram. Soc. Bull.601981202
- N.E.HipedingerA.N.ScianE.F.AgliettiCem. Concr. Res.342004157164
- Y.DongX.LiuQ.MaG.MengJ. Membr. Sci.2852006173181
- X.ZhangD.FangB.LinY.DongG.MengX.LiuJ. Alloys Compd.4872009631638
- N.SaffajS.Alami YounssiA.AlbizaneA.MessouadiM.BouhriaM.PersinM.CretinA.LarbotSep. Purif. Technol.362004107114
- R.J. Ciora Jr and P.K. Liu, U.S. Patent No 5,773,103 (1998).
- Z.-Y.DengT.FukasawaM.AndoG.-J.ZhangT.OhjiActa Mater.49200119391946
- A.I.Rat’koA.I.IvanetsS.M.AzarovInorg. Mater.442008778784
- А.I.IvanetsТ.А.AzarovaV.E.AgabekovS.М.AzarovC.BatsukhD.BatsurenV.G.ProzorovichA.A.Rat’koCeram. Int.4220161657116578
- А.I.IvanetsА.I.RatТ.А.AzarovaS.М.AzarovS.H.Al-KhowaiterО.Al-HarbiS.V.ShemchonokV.А.DobyshV.А.TarasevichV.Е.AgabekovCeram. Int.4020141234312351
- M.M.Lorente-AyzaE.SánchezV.SanzS.MestreCeram. Int.4120151306413073
- M.-M.Lorente-AyzaM.J.OrtsV.Pérez-HerranzS.MestreJ. Eur. Ceram. Soc.35201523332341
- S.BoseC.DasMater. Lett.1102013152155
- A. Standard, C 373-88Stand. Am. Soc. Test. Mater.1999
- N.SaffajM.PersinS.A.YounsiA.AlbizaneM.CretinA.LarbotAppl. Clay Sci.312006110119
- A.KhalfaouiM.HajjajiS.KacimA.BaçaouiJ. Am. Ceram. Soc.89200615631567
- D.NjoyaM.HajjajiD.NjopwouoAppl. Clay Sci.652012106113
- D.MathieuJ.NonyR.Phan-Tan-LuuNew efficient methodology for research using optimal design (NEMROD) softwareLPRAI Marseille2000
- D.MathieuJ.NonyR.Phan-Tan-LuuNew Efficient Methodology for Research using Optimal Design (NEMROD) Software-LPRAIMarseille Fr.1998
- D.MathieuR.Phan-Tan-LuuL’entreprise211997211278
- W.ShiB.LiuX.DengJ.LiY.YangJ. Eur. Ceram. Soc.34201634653472
- C.M.F.VieiraR.SánchezS.N.MonteiroConstr. Build. Mater.222008781787
- M.HajjajiS.KacimM.BoulmaneAppl. Clay Sci.212002203212
- D.Eliche-QuesadaF.A.Corpas-IglesiasL.Pérez-VillarejoF.J.Iglesias-GodinoConstr. Build. Mater.342012275284
- M.LoutouM.HajjajiM.MansoriC.FavottoR.HakkouJ. Environ. Manage.1302013354360
- M.LoutouM.HajjajiM.MansoriC.FavottoR.HakkouJ. Asian Ceram. Soc.420161118
- M.LoutouM.HajjajiM.MansoriC.FavottoR.HakkouJ. Environ. Manage.1772016169176