Abstract
The use of Persea americana has been studied as an alternative source of activated carbon for the removal of dyes from wastewater. Chemical activation using phosphoric acid was employed for the preparation of the activated carbon (C-PAN). The BET surface area and the total pore volumes were found to be 1593 m2/g and 1.053 cm3/g, respectively. This study investigates the effect of some parameters like, dye concentration, adsorbent dose, contact time and pH for the best comprehension of the adsorption manner. Adsorption kinetic follows pseudo-second order kinetic model. Langmuir and Freundlich isotherms models were used to analyze the adsorption equilibrium data and the best fits to the experimental data were provided by Langmuir model. Maximum adsorption capacity is equal to 400 mg/g of Basic Yellow 28 onto the activated carbon derived from Persea americana. Thermodynamic parameters, such as standard Gibbs free energy (ΔG0), standard enthalpy (ΔH0) and standard entropy (ΔS0) has been calculated. The adsorption process was found to be spontaneous and exothermic process.
1 Introduction
Among the different pollutants of aquatic ecosystems, dyes are a large and important group of industrial chemicals for which world production in 1978 was estimated at 640,000 tons of which about 20–30% are wasted in industrial effluents during the textile dyeing and finishing processes (CitationClarke and Anliker, 1980). Moreover, most of these dyes can cause allergy, dermatitis, skin irritation and also provoke cancer and mutation in humans (Clarke and Anliker, Citation1980; Baughman and Perenich, Citation1988). Also, the presence of very small amounts of dyes in water less than one ppm for some dyes is highly visible, very difficult to biodegrade, extremely difficult to eliminate in natural aquatic environments and undesirable (Banat et al., Citation1996; Robinson et al., Citation2001). Currently, much attention has been paid to the removal of dyes from industrial wastewater (Gupta et al., Citation2013, Citation2012a; Jain et al., Citation2003; Mittal et al., Citation2010a, Citation2009a).
Many treatment processes have been applied for the removal of dyes from wastewater such as: Fenton process (CitationBehnajady et al., 2007), photocatalytic degradation (Gupta et al., Citation2012b, Citation2011; Saleh and Gupta, Citation2012; Saravanan et al., Citation2016, Citation2015a,Citationb, Citation2014), sono-chemical degradation (CitationAbbasi and Asi, 2008), photo-Fenton processes (CitationGarcia-Montano et al., 2007), chemical coagulation/flocculation, ozonation, cloud point extraction, oxidation, nano-filtration, chemical precipitation, ion exchange, reverse osmosis and ultra-filtration (Lorenc-Grabowsk and Gryglewic, Citation2007; Malik and Saha, Citation2003; Malik and Sanyal, Citation2004; Banat et al., Citation1996; Tawfik et al., Citation2012). Adsorption techniques have gained favor due to their simplicity in operation, cost-effectiveness and efficiency in the removal of pollutants too stable for conventional methods (Mittal et al., Citation2010b, Citation2009b; Gupta and Nayak, Citation2012; Gupta et al., Citation2015a,Citationb, Citation2012b; Saleh and Gupta, Citation2014). More recently, new researches on the effect of the ultrasound on the adsorption–desorption process have been reported (Asfaram et al., Citation2017; Alipanahpour Dil et al., Citation2017; Ghaedi et al., Citation2015; Mazaheri et al., Citation2016). Almost all the work related to adsorption techniques for color removal from industrial effluents was based on studies using natural and abundant materials. Activated carbon (Regti et al., Citation2016a,Citationb, Citation2017b; Gupta et al., Citation1997, Citation2013; Saleh and Gupta, Citation2014) is still the most popular and widely used adsorbent. Activated carbon can be prepared using a variety of chemical (CitationAttia et al., 2008) and physical (CitationHeibati et al., 2015) activation methods and in some cases using a combination of both types of methods (CitationAlbero et al., 2009). Chemical activation is the process where the carbon precursor is firstly treated with aqueous solutions of dehydrating agents such as phosphoric acid, zinc chloride, sulfuric acid, and potassium hydroxide. Afterwards, the carbon material is dried at 373–393 K to eliminate the water traces followed by its heating between 673 K and 1073 K under nitrogen atmosphere (CitationFaria et al., 2008). The physical activation consists of a thermal treatment of previously carbonized material with suitable oxidizing gases, such as air at temperatures comprised between 623 K and 823 K by using steam and/or carbon dioxide (CitationHeibati et al., 2015).
We have already used the potential efficiency of animal bone meal material (El Haddad et al., Citation2012, Citation2013a,Citationb), calcined mussel shells (El Haddad et al., Citation2014a,Citationb), calcined eggs shells (CitationSlimani et al., 2014) and activated carbon derived from Medlar nuts (CitationRegti et al., 2017a) as new low cost adsorbents to remove anionic and cationic dyes from aqueous solutions. For ongoing our program research for the decolourization solutions, we have used activated carbon derived from natural and low cost materials (Persea americana Nuts). Other studies have been performed using activated carbon prepared from agricultural wastes for the removal of dyes from aqueous solution (Gupta et al., Citation2015a,Citationb).
In this current study, the removal of Basic Yellow 28 dye from aqueous solutions onto activated carbon derived from Persea americana nuts was investigated. The choice of this agricultural wastes returns to the increased consumption of avocado fruit. In this fact, the effect of different parameters such as pH, contact time, adsorbent dose, initial dye concentration and temperature were investigated. The removal rate kinetics, thermodynamics and isotherms for Basic Yellow 28 adsorption onto treated Persea americana were also studied.
2 Materials and methods
2.1 Materials
Persea americana Nuts (PAN) used for the preparation of the activated carbon, was collected, washed with distilled water and dried at room temperature for several dyes. The dried material was then milled and separated by manually shaking stainless steel mesh screens with the opening of standard 0.45 mm. an appropriate weight of PAN was immersed in 25 mL of concentrated phosphoric acid with a mass ratio (1:4) for 12 h and then dried in an oven for 24 h at 105 °C. The PAN was placed in a sealed ceramic oven and pyrolysed at 500 °C and remained constant for 2 h. The resulting activated carbon (C-PAN) was filtered and rinsed with distilled water until the washings were free of excess acid. The final product of C-PAN was dried at 105 °C for 24 h, crushed to get the particles size of 200–300 µm and kept in desiccators for further study.
Adsorption–desorption isotherms of nitrogen at −196 °C were measure with an automatic gas sorption analyzer (NOVA-1000, Quanta Chrome Corporation, USA) in order to determine surface areas and total pore volumes.
The Characterization of C-PAN was achieved by FT-IR spectroscopy and X-ray powder diffraction measurements. FT-IR spectra (4000–450 cm−1 range) were recorded with a Nicolet 5700 FT-IR spectrometer (Thermo Corp, USA) on samples prepared as KBr pellets. The polycrystalline sample of adsorbent was lightly ground in an agate mortar, pestle and filled into 0.5 mm borosilicate capillary prior to being mounted and aligned on an Empyrean PANalytical powder diffractometer (B.V Company) using Cu Kα radiation (λ = 1.54056 Å). Three repeated measurements were collected at room temperature in the 10° < 2θ < 60° range with a step size of 0.01°. Scanning Electronic Microscopy (SEM) images were obtained with (HITACHI-S4100, JAPAN) equipment operated at 20 kV.
De-ionized water was used throughout the experiments for solutions preparation. The adsorption studies for evaluation of the C-PAN adsorbent for the removal of the Basic Yellow 28 dye from aqueous solutions were carried out in triplicate using the batch contact adsorption method. Basic Yellow 28 dye used in this study abbreviated as BY28 was purchased from Sigma–Aldrich (Germany). Chemical structure of BY28 is shown in .
2.2 Adsorption experiments
For the adsorption experiments, fixed amounts of adsorbents (0.2 g/L–1.0 g/L) were placed in a 100 mL glass Erlenmeyer flask containing 50 mL of dye solution at various concentrations (40–100 mg/L), which were stirred during a suitable time (5–65 min) from 303 to 333 K. The pH of the dye solutions ranged from 2 to 12 was adjusted by 0.1 M HCl or 0.1 M NaOH to investigate the effect of pH on adsorption processes. Subsequently, in order to separate the adsorbents from the aqueous solutions, the samples were centrifuged at 3600 rpm for 10 min, and aliquots of 1–10 mL of the supernatant were taken. At predetermined time, the residual dye concentration in the reaction mixture was analyzed by centrifuging the reaction mixture and then measuring the absorbance by UV–Visible spectroscopy of the supernatant at the maximum absorbance wavelength of the sample at 436 nm (CitationZermane et al., 2010).
The amount of equilibrium adsorption qe (mg/g) was calculated using the following expression:(1) where Ce (mg/L) is the liquid concentration of dye at equilibrium, C0 (mg/L) is the initial concentration of the dye in solution. V is the volume of the solution (L) and W is the mass of biosorbent (g). The BY28 removal percentage (%) can be calculated as follows:
(2) where C0 is the initial dye concentration and Ce (mg/L) is the concentrations of dye at equilibrium.
3 Results and discussion
3.1 Characterization of C-PAN adsorbent
In order to investigate the surface characteristic of C-PAN adsorbent, FT-IR and XRD spectrums were recorded. As shown in FT-IR spectrum in , the frequencies of the absorption bands of C-PAN are 876, 1074, 1149, 1563, 2916 and 3415 cm−1. The absorption band at 3415 cm−1 is attributed to the hydroxyl groups (O—H) vibration, bands at 2916 and 1563 cm−1 are assigned for P=O group, while bands at 1149 and 1074 cm−1 are attributed for P—O group and finally band at 876 cm−1 is attributed for P—H group (CitationLiu et al., 2012 CitationWang et al., 2011). These function groups are due to the present of H3PO4 acid as an activation agent in the preparation of C-PAN.
shows an X-ray powder diffraction pattern of C-PAN. An amorphous peak with the equivalent Bragg angle at 2θ = 24.6 was recorded, together with other peaks recorded at 2θ = 17.5°, 31.2° and 47.5°. Thus, as presented in , a wide and high intensity peak between 20 and 30° centered at 25° indicates the amorphous nature of C-PAN adsorbent. Furthermore, XRD data gave clear evidence to the high purity of the amorphous C-PAN.
The surface morphology of the C-PAN adsorbent was examined. shows the SEM image indicating that the surface contains many pores of different size and different shape could be observed, revealing the potential adsorption power and suggesting that dyes were adsorbed on the mesopore or microspore. The BET surface area and the total pore volumes of the obtained Persea americana activated carbon were found to be 1593 m2/g and 1.053 cm3/g, respectively.
3.2 Effect of C-PAN dosage on the adsorption capacity
The effect of the C-PAN dosage on the uptake (mg/g) is shown in . The adsorbent dosage was varied from 0.2 g/L to 1 g/L using V = 50 mL of BY 28 (100 mg/L) at ambient temperature. The adsorption capacities increases with increasing time and the response becomes constant for all amounts studied. The maximum uptake (q = 325 mg/g) was observed with the dosage of 0.2 g/L. The capacity of adsorption decreased slightly with the higher dosage of the adsorbent. The reason may be the interparticle interaction, such as aggregation, resulting from high adsorbent dose, aggregation would lead to a decrease in the total surface area of the adsorbent and on an increase in diffusional path length, while, at equilibrium, the adsorption efficiency (%) increases from 65% to 98% by increasing the C-PAN dose from 0.2 g/L to 1 g/L due to the increase in the number of sites available for dye adsorption, this is in agreement with our previous observations on BY28 dye removal by calcined bones biosorbent (El Haddad et al., Citation2013a,Citationb).
3.3 Effect of contact time and BY28 dye concentration
In order to achieve the effect of contact time and initial dye concentration (40–100 mg/L) with 0.2 g/L of C-PAN and temperature fixed at 25 °C on the adsorption capacity of BY28 by C-PAN, different experiments were realized. depicts these results. It was observed that the adsorption capacity of BY28 is increased with increasing the contact time of all initial dye concentrations. Furthermore, the adsorption capacity dye is increased with the increase of initial dye concentration. Therefore, at higher initial dye concentration, the number of molecules competing for the available sites on the surface of C-PAN was high, hence, resulting in higher basic yellow adsorption capacity.
Figure 6 Effect of initial dye concentration and contact time on the removal of BY 28 from aqueous solution. C-PAN: 0.2 g/L; V = 50 mL and temperature: 25 °C.
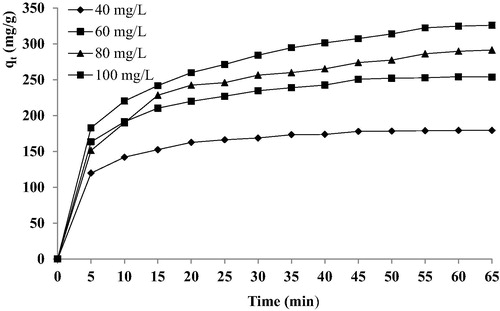
Through these results, it appears that for the first 15 min, the adsorption capacity uptake was rapid then it proceeds at a slower adsorption rate and finally it attains saturation at 30 min. The obtained removal curves were single, smooth and continuous, indicating monolayer coverage of dye on the surface of adsorbent. Analogue results are shown in many works (Mittal et al., Citation2013; El Haddad et al., Citation2012).
3.4 Effect of pH on the removal of BY28 dye onto C-PAN
shows the variation of dye removal versus pH. In this fact, to study the effect of initial pH of aqueous dye solution, we have used a concentration of BY28 dye at 100 mg/L and 0.4 g/L of C-PAN adsorbent, keeping the temperature at 25 °C and at different pH values in the range 2–12. The amount of dye adsorbed onto C-PAN was found to be constant for all pH values being studied. The experiments carried out at different pH show that there was no significant change in the percent removal dye over the entire pH range. This indicates the strong affinity of the dye to C-PAN and that either H+ or OH− ions could influence the dye adsorption capacity. Whatever the pH of the dye solution, the dye removal % is constant and equal to 98%. The constant removal efficiency of BY28 dye by C-PAN adsorbent indicates that the ion exchange between the moieties of BY28 and C-PAN was not the only one mechanism for dye removal in this system. Other type of interactions may involve too. In this fact, the C-PAN can also interact with BY28 dye molecules by hydrogen bonding and van der Waals interactions. A similar result was obtained and previously achieved.
3.5 Thermodynamic study
Thermodynamic data reflect the feasibility and favorability of the adsorption process. Parameters such as free energy change (ΔG0), enthalpy change (ΔH0) and entropy change (ΔS0) can be determined by the change of equilibrium temperature. The free energy change of the adsorption reaction is given by (Smith and Van Ness, Citation1987; Khormaei et al., Citation2007):(3) where ΔG0 is the free energy change (kJ/mol), R is the universal gas constant (8.314 J/mol K), T is the absolute temperature (K) and KC states the equilibrium constants (qe/Ce). The values of ΔH0 and ΔS0 can be calculated from the following equation:
(4) where KC is plotted against 1/T, a straight line with the slope (−ΔH0/R) and intercept (ΔS0/R) are given. The calculated thermodynamic parameters are presented in .
Table 1 Thermodynamic data for the adsorption of BY 28 onto C-PAN.
The positive values of ΔG0 (0.367 kJ/mol, 0.442 kJ/mol, 0.499 kJ/mol and 0.570 kJ/mol) at all temperatures (303 K, 313 K, 323 K and 333 K respectively) confirmed the thermodynamic feasibility. The increasing temperature from 303 to 333 K causes increasing of values of ΔG0 indicating a decrease in feasibility of adsorption at higher temperatures.
Values of ΔH0 and ΔS0 were obtained as −3.81 kJ/mol and −15.38 J/mol K, respectively. The negative value of ΔH0 shows that the adsorption is exothermic in nature. The negative value of ΔS0 suggests that the degree of randomness state at the solid/solution interface increased during the BY28 dye onto C-PAN.
3.6 Adsorption isotherms
Adsorption isotherms are basic requirements for the design of adsorption systems. It can express the relationship between the amounts of adsorbate (BY28) by unit mass of adsorbent (C-PAN) at a constant temperature. Herein, we analyzed our experimental data by Langmuir and Freundlich isotherms models. The best-fitting model was evaluated using the correlation coefficient. In this case, Langmuir and Freundlich isotherm models are applied for giving the experimental data during the isothermal adsorption studies. The linear form of Langmuir isotherm is expressed as (CitationSafa and Bhatti, 2011):(5) where Ce (mg/L) is the equilibrium concentration of BY28 dye, qe (mg/g) is the amount of BY28 adsorbed par unit mass of adsorbent. KL (L/mg) and qm (mg/g) are Langmuir constants related to rate of adsorption and adsorption capacity, respectively. A straight line with slope of 1/qm and intercept of 1/qmKL is obtained when Ce/qe is plotted against Ce. shows the values of these parameters. The essential characteristics of Langmuir equation can be expressed in terms of dimensionless separation factor, RL defined as:
(6) where C0 is the initial concentration of BY28 dye, the RL value implies whether the adsorption is:
Table 2 Adsorption isotherm constants for removal of BY 28 onto C-PAN adsorbent at ambient temperature.
Unfavorable: RL > 1
Linear: RL = 1
Favorable: 0 < RL < 1
Irreversible: RL = 0.
As depicted in , RL values versus the initial dye concentration at ambient temperature are calculated. It was observed that all the RL values obtained were comprised between 0 and 1, showing that the adsorption of BY28 onto C-PAN was favorable. The RL values decrease upon increasing the initial dye concentration, which indicates that the adsorption was more favorable at higher BY28 concentration. The maximum adsorption capacity was calculated (400 mg/g) under ambient temperature.
The linear form of Freundlich isotherm is expressed as (CitationDeniz and Karaman, 2011):(7) where Kf and n are Freundlich isotherm constants. Kf (mg/g) (L/g) is an indication of the adsorption capacity, while 1/n is the adsorption intensity. The plot of log (qe) versus log (Ce) that should give a straight line with a slope of (1/n) and intercept of log (Kf) and all data are shown in . In this case, the value found for n was superior to 1, which proves that the adsorption of BY28 onto C-PAN is favorable. The best fit of experimental data was obtained with the Langmuir model with r2 value 0,997. This result suggests the monolayer and homogenous adsorption.
3.7 Adsorption kinetic
The adsorption kinetics gives the idea about mechanism of adsorption, from which efficiency of process estimated. In order to investigate the adsorption process of BY28 onto C-PAN adsorbent, the data obtained from adsorption kinetic experiments were simulated pseudo-first order and pseudo-second order models. The pseudo-first order equation is generally represented as follows (CitationOzcan et al., 2007):(8) where, qe is the amount of dye adsorbed at equilibrium (mg/g), qt is the amount of dye adsorbed at time t (mg/g), k1 is the first-order rate constant (min−1) and t is time (min).
The values of k1, qe calculated from the equation and the correlation coefficient (r2) values of fitting the pseudo-first order rate model at different concentrations are presented in . The linearity plots of log (qe–qt) versus time at different initial dye concentrations () suggested that the process of dye adsorption did not follow the pseudo-first order rate kinetics. Also from , it is indicated that the values of the correlation coefficients are not high for the different dye concentrations. Furthermore, the estimated values of qe calculated from the equation qe (cal) differ substantially from those measured experimentally qe (exp). That gives confirmation that the adsorption process of BY28 onto C-PAN did not obey the pseudo-first order model.
Figure 8 Pseudo-first order plots for different initial dye concentrations removal using C-PAN adsorbent. C-PAN adsorbent: 0.2 g/L; temperature 25 °C.
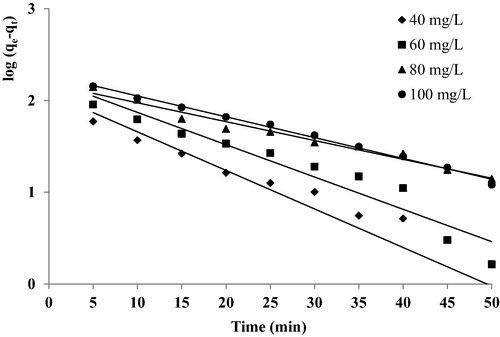
Table 3 Pseudo-first order and pseudo-second order kinetic parameters for BY 28 removal using C-PAN adsorbent.
The pseudo-second order equation is generally represented as follows (CitationMoussavi and Mahmoudi, 2009):(9) where, k2 is the pseudo-second order rate constant (g/mg.min). A plot of t/qt and t should give a linear relationship if the adsorption follows pseudo-second order model. To understand the applicability of the model, a linear plot of t/qt versus time under different dye concentrations were plotted in . The values of k2, qe and correlation coefficients (r2) were calculated from the plot and are given in . The qe (cal) determined from the model along with correlation coefficients indicated that qe (cal) is very close to qe (exp) and correlation coefficient is also greater than 0.99. As a matter of consequence, the system BY28-C-PAN could be well described by the pseudo-second order model.
3.8 Comparison with other derived activated carbon
The value of maximum adsorption capacity qm (mg/g) is of importance to identify which adsorbent shows the highest adsorption capacity and is useful in scale-up considerations. Some studies have been conducted using various types of activated carbon derived from many materials for removing dyes from aqueous solutions. depicts a comparison of the adsorption capacity of C-PAN with that reported for other adsorbents. It can be seen from the that the C-PAN adsorbent show a comparable adsorption capacity with the respect to other adsorbents, revealing that the C-PAN is suitable for the removal basic dye from aqueous solutions.
Table 4 Comparison of maximum monolayer adsorption capacities of C-PAN with those of various AC adsorbents.
3.9 Regeneration of C-PAN
The economic aspect and environmental of the use of adsorbent materials, makes important the reuse of biosorbents, () displays the difference in adsorption capacity(mg/g) of first and five cycles, the adsorption capacity was reduced from 235 to 37.3 mg/g, its due to the reduction in the specific surface of this material. Therefore, C-PAN shows excellent adsorption performance and regeneration, and its use can be extended to environmental applications for wastewater treatment.
4 Conclusion
The results of this study show that C-PAN obtained from the nuts of Persea americana can be successfully used as a low cost and eco-friendly adsorbent for the removal of basic yellow 28(BY28) from aqueous solution and The following conclusions have been drawn from the above investigations:
– | The adsorption process was influenced by a number of factors such as adsorbent dose, pH, contact time, and the initial BY28 concentration. |
– | The uptake (mg/g) was observed to increase with increasing initial dye concentration and decrease with increasing adsorbent dose. The adsorption parameters for the Langmuir and Freundlich isotherms were determined and the equilibrium data were best described by the Langmuir isotherm model with acceptable r2, which indicates that a homogeneous adsorption takes place between BY28 dye and C-PAN. |
– | The pseudo-second order equation was best described for the kinetics of the C-PAN adsorption system due to its high r2. In addition, the theoretical qe generated by the pseudo-second order equation is in good agreement with the experimental qe value. |
– | Thermodynamic studies indicate that the adsorption process is exothermic and spontaneous in nature. |
Notes
Peer review under responsibility of University of Bahrain.
References
- M.AbbasiN.R.AsiSonochemical degradation of Basic Blue 41 dye assisted by nano TiO2 and H2O2J. Hazard. Mater.1532008942947
- A.S.AlberoJ.S.AlberoA.S.EscribanoF.R.ReinosoEthanol removal using activated carbon: effect of porous structure and surface chemistryMicroporous Mesoporous Mater.12020096268
- E.Alipanahpour DilM.GhaediA.AsfaramS.HajatiF.MehrabiA.GoudarziPreparation of nanomaterials for the ultrasound-enhanced removal of Pb2+ ions and malachite green dye: chemometric optimization and modelingUltrason. Sonochem.342017677691
- A.AsfaramM.GhaediaS.HajatiA.GoudarziE.Alipanahpour DilScreening and optimization of highly effective ultrasound-assisted simultaneous adsorption of cationic dyes onto Mn-doped Fe3O4-nanoparticle-loaded activated carbonUltrason. Sonochem.342017112
- A.A.AttiaB.S.GirgisN.A.FathyRemoval of methylene blue by carbons derived from peach stones by H3PO4 activation: batch and column studiesDyes Pigm.762008282289
- S.AttoutiB.BestaniN.BenderdoucheD.LaurentApplication of Ulva lactuca and Systoceira stricta algae-based activated carbons to hazardous cationic dyes removal from industrial effluentsWater Res.47201333753388
- I.M.BanatP.NigamSinghR.MarchantMicrobial decolorization of textile dye containing effluents: a reviewBioresour. Technol.581996217227
- G.BaughmanT.A.PerenichFate of dyes in aqueous systems: solubility and partitioning of some hydrophobic dyes and related compoundsEnviron. Toxicol. Chem.71988183199
- M.A.BehnajadyN.ModirshahlaF.GhanbaryA kinetic model for the decolorization of C. I. Acid yellow 23 by Fenton processJ. Hazard. Mater.148200798102
- E.A.ClarkeR.AnlikerOrganic dyes and pigmentsO.HutzingerThe Handbook of Environmental Chemistry. Anthropogenic Compounds31980Springer-VerlagHeidelberg181215
- F.DenizS.KaramanRemoval of Basic Red 46 dye from aqueous solution by pine tree leavesChem. Eng. J.17020116774
- M.El HaddadR.MamouniR.SlimaniN.SaffajM.RidaouiS.ElAntriS.LazarAdsorptive removal of Reactive Yellow 84 dye from aqueous solutions onto animal bone mealJ. Mater. Environ. Sci.3201210191026
- M.El HaddadR.SlimaniR.MamouniR.LaamariS.RafqahS.LazarEvaluation of potential capability of calcined bones on the biosorption removal efficiency of safranin as cationic dye from aqueous solutionsJ. Taiwan. Inst. Chem. Eng.4420131318
- M.El HaddadR.SlimaniR.MamouniS.ElAntriS.LazarRemoval of two textile dyes from aqueous solutions onto calcined bonesJ. Assoc. Arab. Univ. Basic Appl. Sci.1420135159
- M.El HaddadA.RegtiR.LaamariR.SlimaniR.MamouniS.El AntriS.LazarCalcined mussel shells as a new and eco-friendly biosorbent to remove textile dyes from aqueous solutionsJ. Taiwan. Inst. Chem. Eng.452014533540
- M.El HaddadA.RegtiR.SlimaniS.LazarAssessment of the biosorption kinetic and thermodynamic for the removal of safranin dye from aqueous solutions using calcined mussel shellsJ. Ind. Eng. Chem.202014717727
- P.C.FariaJ.M.OrfãoJ.L.FigueiredoF.R.PereiraAdsorption of aromatic compounds from the biodegradation of azo dyes on activated carbonAppl. Surf. Sci.254200834973503
- K.Y.FooB.H.HameedMicrowave assisted preparation of activated carbon from pomelo skin for the removal of anionic and cationic dyesChem. Eng. J.1732011385390
- J.Garcia-MontanoN.RuizI.MunozX.DomenechJ.A.Garcia-HortalF.TorradesJ.PearlEnvironmental assessment of different Photo-Fenton approaches for commercial reactive dye removalJ. Hazard. Mater.1382007218225
- M.GhaediH.Zare KhafriA.AsfaramA.GoudarziResponse surface methodology approach for optimization of adsorption of Janus Green B from aqueous solution onto ZnO/Zn(OH)2-NP-AC: kinetic and isotherm studySpectrochim. Acta. A1522015233240
- V.K.GuptaA.NayakCadmium removal and recovery from aqueous solutions by novel adsorbents prepared from orange peel and Fe2O3 nanoparticlesChem. Eng. J.18020128190
- V.K.GuptaS.K.SrivastavaD.MohanS.SharmaDesign parameters for fixed bed reactors of activated carbon developed from fertilizer waste for the removal of some heavy metal ionsWaste Manage.171997517522
- V.K.GuptaR.JainA.NayakS.AgarwalM.ShrivastavaRemoval of the hazardous dye Tartrazine by photodegradation on titanium dioxide surfaceJ. Hazard. Mater.31201110621067
- V.K.GuptaI.AliT.A.SalehA.NayakS.AgarwalChemical treatment technologies for waste-water recycling-an overviewRSC Adv.2201263806388
- V.K.GuptaR.JainA.MittalA.TawfikA.SalehA.NayaS.AgarwalS.SikarwaPhotocatalytic degradation of toxic dye amaranth on TiO2/UV in aqueous suspensionsMater. Sci. Eng. C3220121217
- V.K.GuptaR.KumarA.NayakT.A.SalehM.A.BarakatAdsorptive removal of dyes from aqueous solution onto carbon nanotubes: a reviewAdv. Colloid. Interface. Sci.193–19420132434
- V.K.GuptaA.NayakS.AgarwalBioadsorbents for remediation of heavy metals: current status and their future prospectsEnviron. Eng. Res.2012015118
- V.K.GuptaA.NayakB.BhushanS.AgarwalA critical analysis on the efficiency of activated carbons from low-cost precursors for heavy metals remediationCrit. Rev. Environ. Sci. Technol.452015613668
- B.HeibatiS.Rodriguez-CoutoM.Al-GhoutiM.AssifI.TyagiS.AgarwalV.K.GuptaKinetics and thermodynamics of enhanced adsorption of the dye AR 18 using activated carbons prepared from walnut and popular woodsJ. Mol. Liq.208201599105
- A.K.JainV.K.GuptaA.BhatnagarA comparative study of adsorbents prepared from industrial wastes for removal of dyesSep. Sci. Technol.3822003463481
- M.KhormaeiB.NasernejadM.EdrisiT.EslamzadehCopper biosorption from aqueous solutions by sour orange residueJ. Hazard. Mater.1492007269274
- H.LiuJ.ZhangN.BaoC.ChengL.RenC.ZhangTextural properties and surface chemistry of lotus stalk-derived activated carbons prepared using different phosphorus oxyacids: adsorption of trimethoprimJ. Hazard. Mater.2352012367375
- E.Lorenc-GrabowskG.GryglewicAdsorption characteristics of Congo red on coal-based mesoporous activated carbonDyes Pigm.7420073440
- P.K.MalikS.K.SahaOxidation of direct dyes with hydrogen peroxide using ferrous ion as catalystSep. Purif. Technol.312003241250
- P.K.MalikS.K.SanyalKinetics of decolorization of azo dyes in wastewater by UV/H2O2 processSep. Purif. Technol.362004167170
- H.MazaheriM.GhaediA.AsfaramS.HajatiPerformance of CuS nanoparticle loaded on activated carbon in the adsorption of methylene blue and bromophenol blue dyes in binary aqueous solutions: using ultrasound power and optimization by central composite designJ. Mol. Liq.2192016667676
- A.MittalA.KaurA.MalviyaJ.MittalV.K.GuptaAdsorption studies on the removal of coloring agent phenol red from wastewater using waste materials as adsorbentsJ. Colloid Interface Sci.3372009345354
- A.MittalJ.MittalA.MalviyaV.K.GuptaAdsorptive removal of hazardous anionic dye “Congo red” from wastewater using waste materials and recovery by desorptionJ. Colloid Interface Sci.34020091626
- A.MittalJ.MittalA.MalviyaV.K.GuptaRemoval and recovery of Chrysoidine Y from aqueous solutions by waste materialsJ. Colloid Interface Sci.3442010497500
- A.MittalJ.MittalA.MalviyaD.KaurV.K.GuptaDecoloration treatment of a hazardous triarylmethane dye, light green SF (Yellowish) by waste material adsorbentsJ. Colloid Interface Sci.3422010518527
- A.MittalD.JhareJ.MittalAdsorption of hazardous dye Eosin Yellow from aqueous solution onto waste material De-oiled Soya: isotherm, kinetics and bulk removalJ. Mol. Liq.1792013133140
- A.A.MohdA.A.NurS.B.OlugbengaKinetic, equilibrium and thermodynamic studies of synthetic dye removal using pomegranate peel activated carbon prepared by microwave-induced KOH activationWater Resour. Ind.620141835
- G.MoussaviM.MahmoudiRemoval of azo and anthraquinone reactive dyes from industrial wastewaters using MgO nanoparticlesJ. Hazard. Mater.1682009806812
- V.O.NjokuK.Y.FooM.AsifB.H.HameedPreparation of activated carbons from rambutan (Nephelium lappaceum) peel by microwave-induced KOH activation for acid yellow 17 dye adsorptionChem. Eng. J.2502014198204
- A.OzcanC.OmerogluY.ErdoganA.S.OzcanModification of bentonite with a cationic surfactant: an adsorption study of textile dye Reactive BlueJ. Hazard. Mater.1402007173179
- A.RegtiH.Ben El AyouchiaM.R.LaamariH.AnaneS.E.StiribaM.EL HaddadExperimental and theoretical study using DFT method for the competitive adsorption of two cationic dyes from wastewatersAppl. Surf. Sci.3902016311319
- A.RegtiM.R.LaamariS.E.StiribaM.EL HaddadRemoval of Basic Blue 41 dyes using Persea americana-activated carbon prepared by phosphoric acid actionInt. J .Ind.Chem.201610.1007/s40090-016-0090-z
- A.RegtiA.El KassimiM.R.LaamariM.EL HaddadCompetitive adsorption and optimization of binary mixture of textile dyes: a factorial design analysisJ. Arab. Univ. Basic. Appl. Sci.24201719
- A.RegtiM.R.LaamariS.E.StiribaM.EL HaddadUse of response factorial design for process optimization of basic dye adsorption onto activated carbon derived from Persea speciesMicrochem. J.1302017129136
- T.RobinsonG.McMullanR.MarchantP.NigamRemediation of dyes in textile effluent: a critical review on current treatment technologies with a proposed alternativeBioresour. Technol.772001247255
- Y.SafaH.N.BhattiKinetic and thermodynamic modeling for the removal of Direct Red-31 and Direct Orange-26 dyes from aqueous solutions by rice huskDesalination2722011313322
- T.A.SalehV.K.GuptaPhoto-catalyzed degradation of hazardous dye methyl orange by use of a composite catalyst consisting of multi walled carbon nanotubes and titanium dioxideJ. Colloid Interface Sci.3712012101106
- T.A.SalehV.K.GuptaProcessing methods, characteristics and adsorption behavior of tires derived carbons: a reviewAdv. Colloid Interface Sci.211201492100
- R.SaravananV.K.GuptaE.MosqueraF.GraciaPreparation and characterization of V2O5/ZnO nanocomposite system for photocatalytic applicationJ. Mol. Liq.1982014409412
- R.SaravananF.GraciaM.M.KhanV.PoornimaV.K.GuptaA.NarayananZnO/CdO nanocomposites for textile effluent degradation and electrochemical detectionJ. Mol. Liq.2092015374380
- R.SaravananM.Mansoob KhanV.K.GuptaV.MosqueraF.GraciaV.NarayananA.StephenZnO/Ag/CdO nanocomposite for visible light-induced photocatalytic degradation of industrial textile effluentsJ. Colloid Interface Sci.4522015126133
- R.SaravananE.SacariF.GraciaM.M.KhanE.MosqueraV.K.GuptaConducting PANI stimulated ZnO system for visible light photocatalytic degradation of colored dyesMol. Liq. J.221201610291033
- R.SlimaniI.El OuahabiM.El HaddadA.RegtiR.LaamariS.El AntriS.LazarCalcined eggshells as a new biosorbent to remove basic dye from aqueous solutions: thermodynamics, kinetics, isotherms and error analysisJ. Taiwan. Inst. Chem. Eng.45201415781587
- J.M.SmithH.C.Van NessIntroduction to Chemical Engineering Thermodynamicsfourth ed.1987McGraw-HillSingapore
- A.TawfikSalehV.K.GuptaColumn with CNT/magnesium oxide composite for lead(II) removal from waterEnvir. Sci . Poll. Res.19201212241228
- Z.WangE.NieJ.LiY.ZhaoX.LuoZ.ZhengCarbons prepared from Spartina alterniflora and its anaerobically digested residue by H3PO4 activation: characterization and adsorption of cadmium from aqueous solutionsJ. Hazard. Mater.18820112936
- F.ZermaneO.BourasM.BauduJ.P.BaslyCooperative coadsorption of 4-nitrophenol and basic yellow 28 dye onto an iron organo–inorgano pillared montmorillonite clayJ. Colloid Interface Sci.3502010315319