Abstract
A spectrofluorimetric method based on complex formation between Zn2+ ions and 5-(4-flourophenyl)-quinolin-8-ol has been developed for the rapid determination of trace levels of zinc ions. The method is sensitive and selective. The complex formed emit strongly at 535 nm with excitation wavelength at 394 nm. Complexation formation was optimized by studying the effect of pH, molar ratio of the metal to the ligand and the type of buffers. The study indicated that maximum complexation resulted when the pH of the mixture was maintained at 8.0 with 0.2 M carbonate buffer and metal to ligand was at a stoichiometric ratio of 1:2. Under the optimum conditions, the detection limit was 3 ppb. Appropriate validation of the method, yielded acceptable relative standard deviations of less than 2% (n = 5). The method was applied to the trace determination of Zn2+ ions in tap water, mineral water, fresh milk and cream samples successfully.
1 Introduction
Zinc exists naturally in water, soil and air. Its concentration ranges from 10−10 M to 10−6 M (CitationDeZuane, 1990) in uncontaminated water. Zinc has many biological functions and importance. It is required for the synthesize of DNA, functioning of 200 different enzymes and for the production of enzymes that initiate cell division (CitationVallee and Falchuk, 1993). It is a powerful antioxidant and is hence used in the prevention and treatment of cancer (CitationTabrizi, 2007). Zinc is essential for the proper maintenance of ideal hormone levels and endocrine function (CitationJiang and Guo, 2004), and is used to prevent diabetes due to its more sensitivity to insulin (CitationDutra et al., 2006). It has been recently reported that zinc is involved in acute cerebral ischemia-induced blood–brain barrier (BBB) disruption (CitationLi et al., 2016).
Zinc is found in higher concentration in red meat while fowl and fish usually provide significantly lesser amounts. In cow milk, the concentration of zinc is reported to be in the range of 310–445 µg Zn100 g−1 (CitationScherz and Kirchhoff, 2006).
Zinc is essential to the human body when present in trace amounts. Higher concentrations above requirement level, may have detrimental effects such as increase in oxidative stress, disruption to the homeostasis of other essential elements and may be a risk factor in Alzheimer’s disease (Atwood et al., Citation1999; Dansher et al., Citation1997). Hence analytical methods for the determination of trace amounts of zinc are required. The commonly used analytical methods for the quantitative determination of zinc are graphite furnace atomic absorption spectrometry (GF-AAS) (CitationD'Haese et al., 1992), Flame-AAS (FAAS) (Li et al., Citation2007; Han et al., Citation2007), inductively coupled plasma mass spectrometry (ICPMS) (Batista et al., Citation2008; Nakatsuka et al., Citation2007), inductively coupled plasma atomic emission spectroscopy (ICP-AES) (Rao et al., Citation2002; Kara et al., Citation2005), Microwave induced plasma-atomic emission spectrometry (MIP-AES) (CitationOzbek and Akman, 2016), microprobe X-ray (CitationFlinn et al., 2005), spectrophotometry (CitationKilian and Pyrzyn’ska, 2003; CitationShpigun et al., 2006; CitationReddy et al., 2007; CitationPinto et al., 2012), colorimetric sensor (CitationSharma and Amlathe, 2012), and electroanalytical techniques (CitationKruusma et al., 2004), chemiluminescence (CitationLi et al., 2016).
An alternative method for the determination of zinc is molecular fluorescence spectroscopy, which is sensitive, simple, cost effective and provide ease of automation. It has been widely used for chemical analyses in a number of fields. Zinc like many other metals does not possess intrinsic fluorescence but it can form complexes with many fluorigenic ligands. There are many examples of ligands that have been used for complex formation with Zn2+ ions (Tabrizi, Citation2007; Ngwendson et al., Citation2006; Gan et al., Citation2005; Ressalan et al., Citation2005; Yu et al., Citation2007; Al-Kindy et al., Citation2008, Citation2011; Chattopadhyay et al., Citation2006; Kulatilleke et al., Citation2006; Kaur and Verma, Citation2016; Grand et al., Citation2016).
In our laboratory, we have developed a number of spectrofluorimetric methods for the sensitive and selective determination of metal ions in water (Al-Kindy et al., Citation2008, Citation2011, Citation2003, Citation2006, Citation2002, Citation2007). This work reports the use of a novel highly fluorescent ligand, 5-(4-flourophenyl)-quinolin-8-ol, for the determination of Zn2+ ions in water. A phenyl substituent at C5 of 8-hydroxyquinoline ligand leads to interesting emission properties. It is reported that the quantum yield of the complex of 5-(4-flourophenyl)-quinolin-8-ol with aluminum ions is much higher than the quantum yield obtained for aluminum ion complexes with parent 8-hydroxyquinoline (CitationSuliman et al., 2012).
To the best of our knowledge, the analytical application of 5-(4-flourophenyl)-quinolin-8-ol for the determination of Zn2+ ions has not been reported. Therefore, here we are utilizing the quantum efficiency of 5-(4-flourophenyl)-quinolin-8-ol to develop a highly sensitive method for the assay of zinc ions in water using 5-(4-flourophenyl)-quinolin-8-ol. In addition, matrix effects linked to intrinsic interferences from diverse metal ions associated with the determination of Zn2+ ions were investigated. Optimal conditions for the successful determination of zinc ions were ultimately attained and applied to the assay of milk samples, water sample and cream samples. Validation of the method was achieved using the well-established atomic absorption technique.
2 Experimental
2.1 Apparatus
Fluorescence measurements were conducted on a Perkin–Elmer LS55B luminescence spectrometer equipped with a xenon lamp with all instrumental parameters controlled by FL Winlab Software. Measurements of pH were carried out with WTW (pH 3015 Jenway) pH meter after calibrating the instrument daily with buffers pH 4, 7 and 10. Method validation for the determination of zinc in cream and tap water samples were carried out on Spectra Alt 220FS Atomic Absorption spectrometer from Varian.
2.2 Reagents
Sodium acetate and zinc acetate were provided by Fluka (Buchs, Switzerland). Acetic acid and sodium chloride were purchased from Arcos (New Jersey, USA). Magnesium sulfate, calcium chloride, potassium chloride, iron (III) chloride, anhydrous aluminum chloride, copper chloride, sodium dihydrogen orthophosphate, 2-amino-2-(hydroxymethyl)-propane-1,3-diol (TRIS) and sodium carbonate were obtained from BDH (UK). Sodium hydroxide and hydrochloric acid were obtained from Riedel-de Haën (Germany). Methanol HPLC grade (≥99.9%) was obtained from Sigma Aldrich (Germany).
All reagents used were of analytical grade and high-purity water was used from a Milli-Q system (Millipore, Bedford, MA, USA).
2.3 Preparation of buffers
A 0.2 M each of acetate, carbonate, phosphate and TRIS buffer were individually prepared in 500 mL using appropriate amounts of sodium acetate, sodium carbonate, sodium dihydrogen orthophosphate and 2-amino-2-(hydroxymethyl)-propane-1,3-diol respectively. Various pH buffers (2–12) were prepared by adding appropriate amounts of sodium hydroxide or hydrochloric acid to 0.2 M sodium acetate buffer.
2.4 Preparation of zinc acetate standard
A 2.5 × 10−4 M stock solution of zinc acetate dihydrate was prepared by dissolving 5.5 mg of the analytical reagent grade in deionized water to make a final volume of 100.0 mL in a volumetric flask. Working standards were prepared in buffers by appropriate dilution.
2.5 Synthesis
The synthesis pathway leading to the title compound 5-(4-flourophenyl)-quinolin-8-ol are presented in .
2.5.1 Synthesis of 5-bromo-8-benzyloxquinoline (1)
A mixture of 5-bromo-8-hyroxyquinoline (3.00 g, 13.40 mmol), potassium carbonate (1.90 g), benzyl chloride (1.66 mL, 13.90 mmol) and potassium iodide (0.075 g) in (22 mL) of acetone was refluxed under N2 for about 10 h. The reaction mixture was poured into (50 g) ice, filtered and washed with water. The resultant product was recrystallized from solution of ethanol–water and dried under vacuum to yield white to green solid (2.98 g, 71%). m.p 92.5–93.3 °C. υmax (KBr)/cm−1 1589, 1498. δH (400 MHz, CDCl3): 5.43 (2H, s, C(1′)CH2), 6.96 (1H, d, J 8.41, C(7)1H), 7.25 (1H, d, J 8.00, C(5′) H), 7.36 (2H,dd, J 7.11 and 17.78,C(4′) H), 7.49 (2H,d, J 7.35,C(3′)H), 7.53 (1H, dd, J 4.11 and 8.52,C(4)H), 7.61 (1H, d, J 8.43,C(6)H), 8.47 (1H, dd, J 1.24 and 8.53, C(4)H), 8.98 (1H, dd, J 1.17 and 4.01, C(2)H). δC (100.4 MHz, CDCl3): 71.3 C(1′), 110.9 C(7), 112.6 C(5), 123.1 C(3), 127.5 C(3′), 128.4 C(10), 128.8 C(5′), 129.1 C(4′), 130.3 C(6), 135.9 C(4), 136.9 C(2′), 141.7 C(9), 150.2 C(2), 154 C(8).
2.5.2 Synthesis of 8-(benzyloxy)-5-(4-fluorophenyl) quinolone (2)
5-bromo-8-benzyloxquinoline (1.00 g, 3.17 mmol), 4-fluorophenyl boronic acid (0.444 g, 3.17 mmol), tetrabutylammonium chloride (0.0956 g, 0.345 mmol), tetrakis (triphenylphosphine) palladium (0.172 g, 0.149 mmol) were added to air-free two phases mixture of toluene (30 mL) and an aqueous 1 M K2CO3 solution (30 mL). The resulting mixture was stirred under nitrogen at 90 °C for 24 h. The organic layer was separated and the aqueous phase was extracted with toluene (3 × 30 mL). After that, the organic layers were combined, washed with water (2 × 50 mL) and dried over anhydrous Na2SO4. Next, the solvent was evaporated; the residue was dissolved in dichloromethane and filtered over silica-gel using 25% methanol-dichloromethane as the mobile phase. Finally the solvent was evaporated and the product was recrystallized from ethanol to produce a yellow solid (0.600 g, 58%). m.p 140.9–142 °C. υmax (KBr)/cm−1 2223, 1600, 1510. δH (400 MHz, CDCl3): 5.50 (2H, s, C(1′)CH2), 7.07 (1H, d, J 8.25,C(7)H), 7.20–7.14 (2H, m, C(5′)H), 7.28–7.33 (2H, m, C(4′)H), 7.35–7.42 (6H, m, C(3, 3′, 5′′ and 2′′)H), 7.55(1H, d, J 7.25, C(6)H), 8.15 (1H, dd, J 8.57 and 1.64, C(4)H), 9.00 (1H, dd, J 1.63 and 5.77, C(2)H). δC (100.4 MHz, CDCl3): 71.19 C(1′), 109.78 C(7), 122.03 C(3), 127.5 C(3′), 127.64 C(10), 128.21 C(5′), 129.1 C(4′), 131.8 C(6), 134.44 C(4), 137.35 C(2′), 140.94 C(9), 149.64 C(2), 154.27 C(8), 128.27 C(5), 132.07 C(1′′), 131.99 C(2′′),115.67 and 115.88 C(3′′), 161.46 C(4′′).
2.5.3 Synthesis of 5-(4-flourophenyl)-quinolin-8-ol (3)
To a 50-mL round bottom flask equipped with septum and magnetic bar, a solution of Pd-C catalyst (1.20 g) and methanol (10 mL) was added by syringe and stirred for one hour under hydrogen gas. Then a solution of 8-(benzyloxy)-5-(4-fluorophenyl) quinoline (0.500 g 1.52 mmol) in methanol (10 mL) was added by injection. The TLC plate was used to monitor the reaction. When all the starting material was consumed, the black solution was filtered to separate the Pd-C catalyst from the product and the solvent was evaporated by rotary evaporator. Finally the yellow solid was recrystallized form isopropanol to yield (0.100 g, 31%). υmax (KBr)/cm−1 3345, 1601, 1502. δH (400 MHz, CDCl3): 7.18 (1H, d→t, J 8.64,C(7)H), 7.38–7.45 (4H, m, C(2′′, 3 and 6)H), 8.23 (1H, d, J 8.23, C(4)H), 8.81 (1H, d, J8.81, C(2)H). δC (100.4 MHz, CDCl3): 108.24 C(7), 120.31 C(3), 125.31 C(10), 126.98 C(6), 128.25 C(5), 130.03 C(2′′), 133.29 C(4), 115.95C(9), 150.10 C(2), 159.52 C(8), 161.98 C(4′′), 113.81 C(3′′), 130.12 C(1′′).
2.6 Preparation of 5-(4-flourophenyl)-quinolin-8-ol solution
A 1.0 × 10−3 M stock solution of 5-(4-flourophenyl)-quinolin-8-ol, was prepared by dissolving 11.9 mg of the ligand in methanol to make a final volume of 50.0 mL in a volumetric flask. Working solutions were prepared by appropriate dilutions.
2.7 Sample preparations
1 mL of each of the fresh milk samples purchased from the local market was transferred into a 100 mL volumetric flask and diluted to the mark with deionized water. Then appropriate volumes were pipetted in 10 mL volumetric flask containing 1 mL of the fluorescence reagents 5-(4-flourophenyl)-quinolin-8-ol, mixed thoroughly and filled to the mark with carbonate buffer of pH 8.0.
On the other hand, the cream sample was prepared by boiling 1 g of the cream in two mL hydrochloric acid and de-ionized water. The contents were then accurately transferred into a100 mL volumetric flask and filled to the mark with de-ionized water. 1 mL was pipetted in 25 mL volumetric flask and diluted with de-ionized water. Then 2 mL was taken in 10 mL volumetric flask and 1 mL of 5-(4-flourophenyl)-quinolin-8-ol was added, and filled to the mark by carbonate buffer of pH 8.0.
Standard particulate matter was removed from Tap water samples obtained from Sultan Qaboos University campus by filtering through a Whatman filter. The samples were then spiked with appropriate volumes of a 10-ppm Zn2+ solution. For the determination of zinc in the sample appropriate aliquots of the diluted solutions were mixed with the ligand and the fluorescence intensity was measured at pH 8.0 carbonate buffer.
Mineral water samples were spiked with 10 ppm Zn2+ standard. The determination of Zn2+ ions was carried out in the same manner as for tap water samples described above.
2.8 Preparation of Zn-5-(4-flourophenyl)-quinolin-8-ol complex
A hot solution of 5-(4-flourophenyl)-quinolin-8-ol (0.2 g, 0.836 mmol) in methanol (20 mL) was added dropwise to a stirred solution containing zinc chloride (0.057 g, 0.408 mmol) and ammonium acetate (0.329 g, 4.281 mmol), dissolved in 20 mL of deionized water. The mixture was refluxed for four hours and the resulting yellowish-green solid was filtered off, washed with water and methanol, and dried in vacuo. This compound was characterized by IR, H NMR spectroscopy and ESI-MS spectrometry.
3 Results and discussion
The complex formation between Zn2+ ions and 5-(4-flourophenyl)-quinolin-8-ol ligand was initially studied in methanol system in order to determine spectra characteristics, optimum ratio of Zn2+ ions to ligand and effect of varying the concentration of ligand. Using UV–visible spectrophotometry it was observed that the 5-(4-flourophenyl)-quinolin-8-ol absorbs strongly at 330 nm. Upon addition of Zn2+ ions to the ligand another strong absorption at 400 nm starts to emerge indicating formation of the complex and the absorption spectra is shown in .
Fig. 1 UV-visible spectra of FPHQ (dashed brown line) and FPHQ-Zn complex. [FPHQ] = 0.1 mM, [Zn2+] = 0.1 mM.
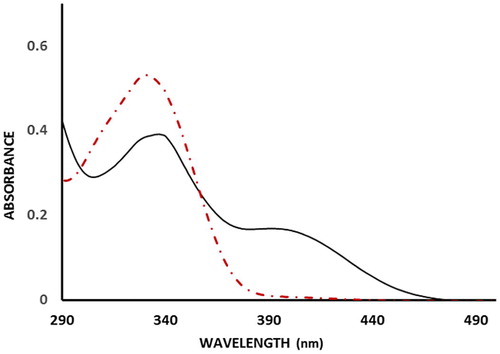
The fluorescence spectra of 5-(4-flourophenyl)-quinolin-8-ol (1.0 × 10−5 M) in methanol and its Zn2+ complex is shown in . 5-(4-flourophenyl)-quinolin-8-ol exhibited a fluorescence signal at λem 540 nm when excited at 390 nm. It is clear from that when Zn2+ ions were added to the solution of 5-(4-flourophenyl)-quinolin-8-ol the fluorescence intensity increased considerably compared to that of the ligand alone. This significant enhancement of the emission was accompanied by a red shift in excitation wavelength and emission wavelength from 390 nm to 394 nm and from 540 to 550 nm respectively. The shift in wavelength and the enhanced fluorescence emission implies complex formation between zinc and hydroxyl-pyridyl nitrogen of the 5-(4-flourophenyl)-quinolin-8-ol moiety (). This behavior of the 5-(4-flourophenyl)-quinolin-8-ol molecule was anticipated from previous reports that the hydroxy quinoline functional group is capable of forming complexes with many metal ions (CitationDangleterre et al., 2008).
Fig. 2 Excitation and emission spectra of 5-(4-flourophenyl)-quinolin-8-ol and its Zn complex (1) 5-(4-flourophenyl)-quinolin-8-ol (2) with 1.0 × 10−5 M of Zinc ions, [5-(4-flourophenyl)-quinolin-8-ol] = 1.0 × 10−5 M, [Carbonate buffer] = 0.2 M, pH = 8.0 FPHQ λex = 390 nm. λem = 540 nm, For Zn- FPHQ, λex = 394 nm, λem = 550 nm.
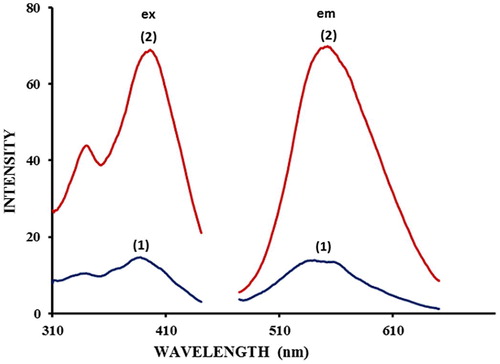
Scheme 2 A schematic representation of the complexation reaction of 5-(4-flourophenyl)-quinolin-8-ol with zinc ions.
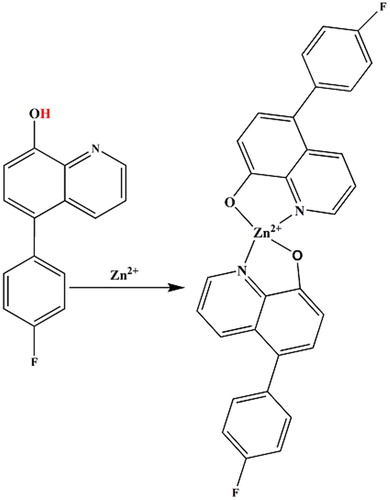
The presence of hydroxyl quinoline functional group in 5-(4-flourophenyl)-quinolin-8-ol, rendered the ligand less fluorescent when compared to its zinc complex. This may is due to intramolecular proton-transfer processes from hydroxyl group to the pyridyl nitrogen atom to form a low emission photo-tautomer. When complexation occurs, the intramolecular proton transfer described above is inhibited hence resulting in the enhancement of the fluorescence intensity. Furthermore, the effective rigidity of the molecule is increased during coordination of the ligand to the metal ion which minimizes non-radiative dissipation processes. The introduction of a fluoro-phenyl moiety at C5 of the 8-hydroxyquinoline has significantly enhanced the fluorescence of the complex due to the extension of the π-system. It is well known that for systems associates with ππ∗ transitions absorption and fluorescence involve the same electronic states; therefore both absorption and emission rate constants are expected to be larger for molecular systems that possess large molar absorptivity (CitationIngle and Crouch, 1988).
3.1 Complex stoichiometry
The optimum ratio of metal/ligand was studied by varying the concentration of zinc ions from 1.0 × 10−6 to 2.0 × 10−5 M while maintaining the molarity of 5-(4-flourophenyl)-quinolin-8-ol a constant at 1.0 × 10−5 M in order to ascertain the maximum complexation of the metal. The fluorescence intensity of the complex increases with increasing the ratio of Zn2+: 5-(4-flourophenyl)-quinolin-8-ol (). The stoichiometry of the complex was found to involve one Zn2+ ion and two ligand anions (5-(4-flourophenyl)-quinolin-8-ol−) as determined by intersection of the graph at a ratio of 1:2, on extrapolation of the linear part of the curves. On studying the complexation further, using continuous variation method (), maximum fluorescence intensity was obtained at a mole fraction of Zn2+ of 0.35, supporting further, that the ratio of Zn2+: 5-(4-flourophenyl)-quinolin-8-ol− in the complex is 1: 2.
Fig. 3 The fluorescence intensity of Zn (5-(4-flourophenyl)-quinolin-8-ol) versus mole ratio of Zn ions: 5-(4-flourophenyl)-quinolin-8-ol ligand. λem = 550 nm, λex = 394 nm.
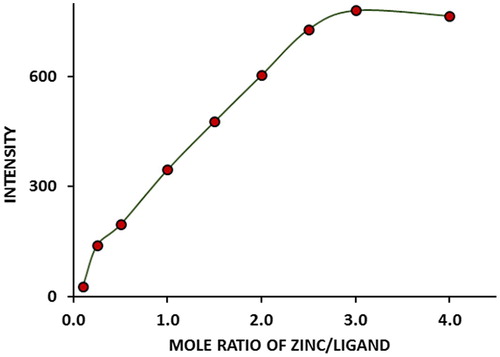
Fig. 4 Job's plot for the determination of stoichiometry of Zn (5-(4-flourophenyl)-quinolin-8-ol). [5-(4-flourophenyl)-quinolin-8-ol] = [Zn ions] = 1.0 × 10−4 M.
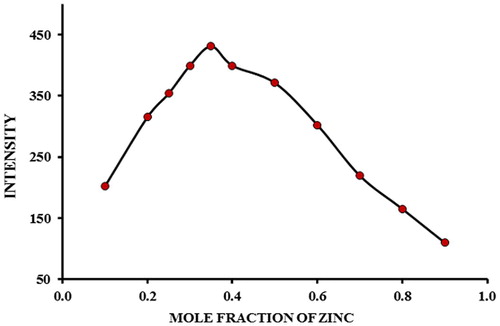
Furthermore, we isolated the zinc complex of 5-(4-flourophenyl)-quinolin-8-ol and characterized it using ESI-MS and 1H NMR spectra (Fig. S1 and S2). The results obtained confirmed the formation of 1:2 Zn2+: 5-(4-flourophenyl)-quinolin-8-ol− complex.
3.2 Effect of pH
The complexation of Zn2+ ions by the ligand is greatly influenced by the pH of the reaction media. Therefore, it was necessary to determine the pH of the system that produced maximum complexation formation between the metal and the ligand. The fluorescence intensity of the complex was measured in buffer solutions of pH 2–12. For the pH range from 2.0 to 4.0, the fluorescence intensity of the ligand was higher than that of the complex suggesting that no complexation occurs between Zn2+ ions and the ligand. On increasing the pH between 5.0 and 9.0 the fluorescence intensity increases sharply from pH 5.0 to 6.0 and remained almost a constant in the pH range 7.0–9.0. When the pH is increased further, a decrease in fluorescence intensity was observed (). Clearly, in acidic medium dissociation of the complex occurs accompanied by re-protonation of the hydroxyl group and pyridyl nitrogen in the oxine ring, hence the reduction in the fluorescence intensity. With increasing concentration of OH− at higher pH, 5-(4-flourophenyl)-quinolin-8-ol− compete with OH− for the Zn2+ ion, which decreases the amount of 5-(4-flourophenyl)-quinolin-8-ol-Zn complex formed thereby lowering the fluorescence intensity. Similar results were previously reported for other zinc complexes (CitationTabrizi, 2007, and CitationAl-Kindy et al., 2008). The optimum pH in this study was therefore maintained at 8.0 using carbonate buffer.
3.3 Effect of varying type of buffer
The type of buffer is known to affect the complex formation between metal ions and ligand. Hence the influence of type of buffer was investigated by measuring the fluorescence intensity of the complex at a pH of 8.0 in various types of buffers. Maximum fluorescence intensity was observed in the presence of carbonate buffer followed by TRIS, acetate and finally phosphate buffer (). These results indicate that carbonate ions exert less competition with the ligand for Zn2+ and hence resulting in higher complexation. On the other hand, phosphate ions may exert more competition with 5-(4-flourophenyl)-quinolin-8-ol for complex formation with Zn2+ and hence less Zn-5-(4-flourophenyl)-quinolin-8-ol complex is formed.
Fig. 6 Effect of type of buffer on fluorescence intensity of Zn (5-(4-flourophenyl)-quinolin-8-ol) complex.
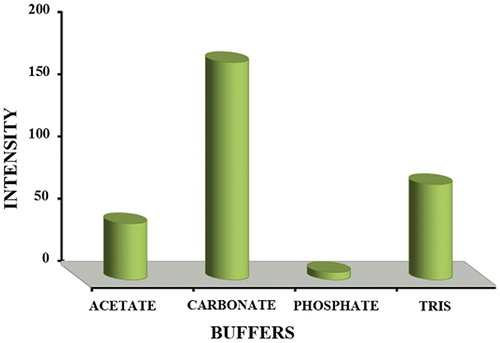
The fluorescence of 5-(4-flourophenyl)-quinolin-8-ol in aqueous system revealed a band at 535 nm emission with an excitation at 394 nm. These results exhibited a blue shift of about 15 nm when compared to the spectra in methanol where an emission wavelength of 550 nm was observed (). The excitation wavelength in aqueous system on the other hand, was similar to that observed in methanol. These results suggest that the complex maybe destabilized in aqueous system. Similarly, addition of Zn2+ ions resulted in the fluorescence enhancement ().
3.4 Analytical figures of merit
Using the optimum conditions discussed above, a plot of luminescence intensity I, versus Zn2+ ions concentration, C, was found to be linear over the concentration range of 50–1600 ppb. The calibration equation: I = 429.9 C + 1.78, with a correlation coefficient R2 of 0.998 was obtained, while the minimum detection limit (3Sb/m) where, Sb is the standard deviation of the blank (n = 5) and m is the slope, is 3.0 ppb. The reproducibility of the method was obtained for standard solutions of zinc and was found to be less than 1.0% (n = 5) indicating excellent precision.
3.5 Effect of interference
The effect of various species usually present in water samples on the fluorescence intensity of the Zn-5-(4-flourophenyl)-quinolin-8-ol complex was studied. A solution containing 65 ppb of Zn2+ and 1.0 × 10−5 M ligand was prepared in the presence of various concentrations of Ca2+, Mg2+, Na+, K+, Fe3+ and Cu2+. The results shown in and indicate that little or no interference is observed in presence of Ca2+, Mg2+, Na+ and K+. However, Fe3+ and Cu2+ gave a lower recovery due to the quenching effect of these species on the fluorescence intensity of Zn-5-(4-flourophenyl)-quinolin-8-ol complex as observed in previous fluorimetric studies involving Zn2+ ions (Compaino et al., Citation1991; Garcia de Torres et al., Citation1989). This renders the method not directly applicable to samples with high contents of Fe3+ and Cu2+ ions such as industrial wastewater. However, in presence of high concentrations of these ions masking agents can be used to eliminate their negative effects.
Fig. 8 Effect of interference on fluorescence intensity of Zn (5-(4-flourophenyl)-quinolin-8-ol) complex. . [Zn2+] ions = 65 ppb, [Ca2+] = [Mg2+] = [Na+] = 50 ppm, [K2+] = [Fe3+] = 80 ppm, [Cu2+] = 60 ppm.
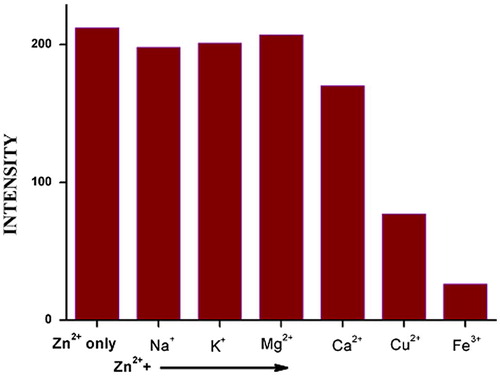
Table 1 Analysis of zinc in various water samples by the proposed method.
3.6 Application
The applicability of the method was evaluated to the determination of Zn2+ ions in potable water samples, such as commercial bottled water, and tap water, where satisfactory recoveries for Zn2+ ions were obtained with values ranging from 97 to 103% indicating that there was no evidence of interference from the matrix as is shown in .
The proposed fluorimetric method was validated by comparing the proposed method with flame-AAS procedure as a reference method for the analysis of tap water samples, cream samples and milk samples with five independent determinations. Both methods reported reasonable recoveries and relative standard deviations and is suitable for the determination of Zn2+ ions in natural water, milk and cream samples without fear of interferences from the matrix as is shown in . Comparable accuracy and precision of the two methods was inferred from the Student’s t-test and the F-test (). These results suggest that the proposed method may be applied for routine analysis as an alternative to flame- AAS method. Also it is noticeable that, trace metals quantification by flame-AAS requires preconcentration step to enhance the sensitivity and selectivity levels due to their extremely low concentrations and matrix interferences. When compared to flame-AAS method, the proposed method is simple, time saving and more laborious as it required the addition of reagents however this step is only a small part of the overall procedure.
Table 2 Analysis of Zn by the proposed method and by the standard FAAS method.
4 Conclusion
A sensitive and selective fluorimetric method for the determination of Zn2+ ions based on the complexation with 5-(4-flourophenyl)-quinolin-8-ol in carbonate buffer pH 8.0 is described. It was then applied to the analysis of Zn2+ ions in water, cream and milk samples. Overall results confirmed the applicability of the proposed procedure for the trace determination of zinc ions even in real samples with good detection limit and precision with time saving economical procedure that does not require any pre-treatment of the analyte samples.
Acknowledgements
The authors wish to express their gratitude to Sultan Qaboos University for providing financial support required for carrying out this research work.
Notes
Peer review under responsibility of University of Bahrain.
References
- S.M.Z.Al-KindyR.BadiaM.E.Diaz-GarciaFluorimetric monitoring of molecular imprinted polymerAnal. Lett.35200217631774
- S.M.Z.Al-KindyF.O.SulimanS.B.SalamaA sequential injection method for the fluorimetric determination of aluminum in drinking water using 8-hydroxy-7-(4-sulfo-1naphthylazo)-5-quinoline sulfonic acidMicrochem. J.742003173179
- S.M.Z.Al-KindyF.O.SulimanA.V.PillayFluorimetric determination of aluminium using sequential injection analysis (SIA): state of our art and future developmentsInstrum. Sci. Technol.342006611615
- S.M.Z.Al-KindyS.S.Al-GhamariF.O.SulimanA sequential injection method for the fluorimetric determination of aluminum in drinking water using 8-hydroxy-7-(4sulfo-1-naphthylazo)-5-quinoline sulfonic acidSpectrochim. Acta Part A68200711741179
- S.M.Z.Al-KindyS.T.Al-BulushiF.O.SulimanSpectrofluorimetric determination of zinc using 8-hydroxy-7-(4-sulfo-1-naphthylazo)-5-quinoline sulfonic acidSpectrochim. Acta, Part A712008676681
- S.M.Z.Al-KindyK.H.Al-HinaiF.O.SulimanH.J.Al-LawatiA.V.PillayDevelopment of a selective fluorimetric technique for rapid trace determination of zinc using 3 hydroxy flavonesArabian J. Chem.42011147152
- C.S.AtwoodX.HuangR.D.MoirR.E.TanziA.I.BushRole of free radicals and metal ions in the pathogenesis of Alzheimers diseaseMet. Ions Biol. Syst.361999310320
- B.L.BatistaJ.A.RodriguesL.TormenA.J.CurtiusJ.F.BarbosaSimultaneous determination of Cd, Cu, Mn, Ni, Pb and Zn in nail samples by inductively coupled plasma mass spectrometry (ICP-MS) after tetramethylammonium hydroxide solubilization at room temperature: comparison with ETAASTalanta762008576579
- N.ChattopadhyayC.A.MallickS.SenguptaPhotophysical studies of 7-hydroxy-4-methyl-8-(4’-methylpiperazin-1’-yl) methylcoumarin: a new fluorescent chemosensor for zinc and nickel ions in waterJ. Photochem. Photobiol., A17720065560
- R.CompainoS.Hemiindez-CassouM.D.PratFlow-injection determination of zinc by fluorescence spectrometryAnal. Chim. Acta2551991325328
- L.DangleterreC.P.CornardC.LapougeSpectroscopic and theoretical investigation of the solvent effects on Al(III)–hydroxyflavone complexesPolyhedron27200815811590
- G.DansherK.B.JensenC.J.FredericksonK.KempA.AndreasenS.JuhlIncreased amount of zinc in the hippocampus and amygdala of of Alzheimer's disease brains: a proton-induced X-ray emission spectroscopic analysis of cryostat sections from autopsy materialJ. NeuroSci. Methods7619975359
- J.DeZuaneHandbook of Drinking Water Quality Standards and Controls1990Van Nostrand Rein-holdNew York
- P.C.D'HaeseL.V.LambertsA.O.VanheuleM.E.D.BroeDirect determination of zinc in serum by Zee-man atomic absorption spectrometry with a graphite furnaceClin. Chem.38199224392443
- R.L.DutraH.F.MaltezE.CarasekDevelopment of an on-line pre-concentration system for zinc determination in biological samplesTalanta692006488493
- J.M.FlinnD.HunterD.H.LinkousA.LanzirottiL.N.SmithJ.B.F.BrightwelEnhanced zinc consumption causes memory deficits and increased brain levels of zincPhysiol. Behav.832005793803
- W.GanS.B.JonesJ.H.ReibenspiesR.D.HancockA fluorescent ligand rationally designed to be selective for zinc (II) over larger metal ions. The structures of the zinc (II) and cadmium (II) complexes of N,N-bis(2 methylquinoline)-2-(2-aminoethyl) pyridineInorg. Chim. Acta358200539583966
- A.Garcia de TorresA.K.ChakrabartiP.E.UreñaJ.M.PavonSensitive spectrofluorimetric determination of zinc at ultra-trace levelsAnal. Chim. Acta2171989363369
- M.M.GrandP.ChocholousJ.RůžičkaP.SolichC.I.MeasuresDetermination of trace zinc in seawater by coupling solid phase extraction and fluorescence detection in the Lab-On-Valve formatAnal. Chim. Acta92320164554
- S.HanW.GanQ.SuOn-line sample digestion using an electromagnetic heating column for the determination of zinc and manganese in tea leaf by flame atomic absorption spectrometryTalanta72200714811486
- J.D.IngleJr.S.R.CrouchSpectrochemical Analysis2nd ed.1988Prentice HallNew Jersey
- P.JiangZ.GuoFluorescent detection of zinc in biological systems: recent development on the design of chemosensors and biosensorsCoord. Chem. Rev.2482004205229
- D.KaraA.Fisherl.S.J.HilPreconcentration and determination of trace elements with 2,6-diacetylpyridine functionalized Amberlite XAD-4 by flow injection and atomic spectroscopyAnalyst130200515181523
- G.KaurN.VermaZinc finger peptide based optic sensor for detection of zinc ionsBiosens. Bioelectron.862016466471
- K.KilianK.Pyrzyn’skaSpectrophotometric study of Cd(II), Pb(II), Hg(II) and Zn(II) complexes with 5,10,15,20-tetrakis(4-carboxylphenyl) porphyrinTalanta602003669678
- J.KruusmaC.E.BanksL.NeiR.G.ComptonElectroanalytical detection of zinc in whole bloodAnal. Chim. Acta51020048590
- C.P.KulatillekeS.A.de SilvaY.EliavA coumarin based fluorescent photoinduced electron transfer cation sensorPolyhedron25200625932596
- Q.LiX.ZhaoQ.LvG.LiuThe determination of zinc in water by flame atomic absorption spectrometry after its separation and preconcentration by malachite green loaded microcrystalline triphenylmethaneSep. Purif. Technol.5520077681
- J.LiC.YinF.HuoDevelopment of fluorescent zinc chemosensors based on various fluorophores and their applications in zinc recognitionDyes Pigm.1312016100133
- S.NakatsukaK.OkamuraK.NorisuyeY.SohrinSimultaneous determination of suspended suspended particulate trace metals (Co, Ni, Cu, Zn, Cd and Pb) in seawater with small volume filtration assisted by microwave digestion and flow injection inductively coupled plasma mass spectrometerAnal. Chim. Acta59420075260
- J.N.NgwendsonC.L.AmiotD.K.SrivastavaA.BanerjeeDesign of a zinc(II) ion specific fluorescence sensorTetrahedron Lett.47200623272330
- N.OzbekS.AkmanMethod development for the determination of calcium, copper, magnesium, manganese, iron, potassium, phosphorus and zinc in different types of breads by microwave induced plasma-atomic emission spectrometryFood Chem.2002016245248
- J.PintoM.Garcia-VargasC.MorenoEnhanced spectrophotometric methods for trace metal determination in waters: zinc as an exampleAnal. Methods42012147152
- K.S.RaoT.BalajT.P.RaoY.BabuG.R.K.NaiduDetermination of iron, cobalt, nickel, manganese, zinc, copper, cadmium and lead in human hair by inductively coupled plasma-atomic emission spectrometrySpectrochim. Acta Part B57200213331338
- K.J.ReddyR.KumarA.V.ReddySpectrophotometric determination of zinc in foods using N-ethyl-3-thiosemicarbazone: evaluation of a new analytical reagentFood Chem.1012007585591
- S.RessalanC.S.P.IyerAbsorption and fluorescence spectroscopy of 3-hydroxy-3-phenyl-1-o-o-carboxyphenyltriazene and its copper (II), nickel(II) and zinc(II) complexes: a novel fluorescence sensorJ. Lumin.1112005121129
- H.ScherzE.KirchhoffTrace elements in foods: zinc contents of raw foods–a comparison of data originating from different geographical regions of the worldJ. Food Comp. Anal192006420433
- R.D.SharmaS.AmlatheQuantiatative determination and removal of zinc using disposable colorimetric sensors: an appropriate alternative to optodesJ. Chem. Pharm. Res.4201210971105
- L.K.ShpigunY.V.ShushenachevP.M.KamilovaKinetic separation in flow injection spectrophotometry: simultaneous determination of copper and zinc in a single runAnal. Chim. Acta3602006573574
- F.O.SulimanS.N.Al-BusafiM.Al-RisiK.N.Al-BadiSynthesis, characterization and DFT investigation of aluminium complexes of ary-substituted-8-hydroxyquinolineDyes Pigm.92201211531159
- A.B.TabriziCloud point extraction and spectrofluorimetric determination of aluminium and zinc in foodstuffs and water samplesFood Chem.100200716981703
- B.L.ValleeK.H.FalchukThe biochemical basis of zinc physiologyPhysiol. Rev.73199379118
- T.YuW.SuW.LiZ.HongR.HueB.LiA Schiff base zinc complex and its electroluminescent propertiesThin Solid Films515200740804084
Appendix A
Supplementary data
Supplementary data associated with this article can be found, in the online version, at http://dx.doi.org/10.1016/j.jaubas.2017.01.004.