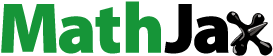
Abstract
We tested alkaline-activated montmorillonite as a low-cost adsorbent for simultaneous removal of Ni(II) and Mn(II) ions from solution. The experiment was performed by batch adsorption to evaluate the effects of pH, initial metal ion concentration, particle size, adsorbent dose, contact time and temperature on adsorption. Alkaline modification of montmorillonite increased the specific surface area from 23.2 to 30.7 m2/g and the cation exchange capacity from 90.78 to 94.32 mEq/100 g. The adsorption capacity of the montmorillonite for Ni(II) and Mn(II) ions increased with alkaline modification. The adsorbent was characterized by Fourier transform infrared, X-ray diffraction and scanning electron microscopy. When four isotherms, the Langmuir, Freundlich, Temkin and Dubinin–Radushkevich models, were applied to the experimental data, the best fit was obtained with the Freundlich model. The kinetic data were analysed with pseudo-first order, pseudo-second order, Elovich and intraparticle diffusion rate equations; greater conformity was found with the Elovich equation. Thermodynamic studies revealed a spontaneous, endothermic physical adsorption process.
1 Introduction
Pollution of water with toxic substances in industrial effluents is a major concern for human health and environmental quality, heavy metals being the most dangerous components. It has been reported that the toxicity due to metallic discharges into the environment far exceeds the combined total toxicity of all radioactive and organic waste [Citation1]. Heavy metals are commonly found in wastewater from many industries, such as paints, batteries, ceramic glazes, pigments, dyes, glass, ink, matches, fireworks and metal products [Citation2]. Although small amounts of many heavy metals are necessary in normal biological cycles, most become toxic at high concentrations. Heavy metals are toxic to living organisms because they tend to persist in the environment, as they are non-biodegradable and accumulate, becoming concentrated in the food chain [Citation3]. Metals such as lead, nickel, cadmium, manganese, chromium, copper, mercury and zinc are known to be significantly toxic [Citation4]. Manganese in particular is considered a pollutant mainly because of its organoleptic properties; in high concentrations, it causes neurological disorders [Citation5]. High concentrations of nickel cause serious health effects, including liver and heart damage, skin irritation, nasal cancer, headache and dermatitis [Citation6]. Therefore, removal of these metals from industrial wastewater is important.
Several techniques have been used to remove heavy metals from effluents, such as oxidation, coagulation–flocculation, membrane filtration, sedimentation, solvent extraction, ion exchange, reverse osmosis, evaporation, reduction and adsorption [Citation7]. Most of these processes, however, are expensive, inefficient and inapplicable to a wide range of pollutants and toxic wastes that are difficult to treat [Citation8]. Adsorption is the generally preferred method for removing heavy metal ions in terms of initial cost, flexibility and simplicity of design, ease of operation, insensitivity to toxic pollutants and the availability of different adsorbents [Citation9]. Furthermore, it does not result in the formation of harmful substances, a problem with most other methods. Although activated carbon has been the substance of choice for treating wastewater containing heavy metals, because of its good adsorption [Citation4,Citation10], it is expensive, which limits its widespread use. As a result, many scientists have sought cheaper alternatives.
Low-cost adsorbents used to treat wastewater containing heavy metals include biomass and agricultural waste, algae, clay, zeolites, soil and sawdust [Citation11–Citation18], and use of natural and modified forms of montmorillonite has been reported [Citation19–Citation22]. Montmorillonite has a high specific surface area and is an effective adsorbent. It adsorbs mainly by two mechanisms: cation exchange in the interlayers resulting from interactions between ions and the negative permanent charge and also formation of inner sphere complexes through Si–O− and Al–O− groups at the clay particle edges [Citation19,Citation21]. Modification of various clay minerals by alkaline treatment has been found to improve their adsorptive capacity [Citation23–Citation25].
This study follows the same direction, with use of a low-cost, eco-friendly, readily accessible montmorillonite obtained from Ugwoba, Enugu State, Nigeria, as an alternative adsorbent for the removal of Ni(II) and Mn(II) ions from aqueous solutions. The effect of alkaline treatment on the adsorption potential of the clay was measured under various experimental conditions: pH, initial metal ion concentration, adsorbent particle size, adsorbent dose, contact time and temperature. Isotherms and kinetic and thermodynamic models were used to further understand the mechanism of the adsorption process.
2 Experimental
2.1 Adsorbent preparation
The montmorillonite was collected from Ugwoba in the Oji River local government area, Enugu State, Nigeria, and then dissolved in excess distilled water in a pretreated plastic container with appropriate stirring; the mixture was then filtered through a 500-μm mesh sieve, and the filtrate was kept for 24 h to settle. Excess water was decanted from the top of the filtrate, and the residue obtained was sun dried for several days, oven dried at 105 °C for 4 h, then pulverized to obtain unmodified montmorillonite clay.
2.2 Alkaline modification of adsorbent
A portion of 200 g of unmodified montmorillonite clay was added to 500 mL of 1 mol/L NaOH solution, stirred for 30 min and left for 24 h at a room temperature of 27 °C. The aqueous phase was decanted, and the process was repeated with 500 mL of 0.5 mol/L KOH; the aqueous phase was again decanted, and the residue washed with excess distilled de-ionized water to neutral pH. The clay was oven-dried at 100 °C for 16 h then pulverized and passed through mesh sieves of sizes 100–500 μm to obtain alkaline-modified montmorillonite clay. The adsorbent was stored in a glass bottle for further use.
2.3 Characterization of adsorbents
The chemical composition of the clays was characterized by atomic absorption spectrophotometry (Buck Scientific model 210VGP) after digestion with nitric and hydrofluoric acid. The methylene blue absorption test method was used to determine specific surface area [Citation26]. Cation exchange capacity was determined by the ammonium acetate method [Citation27]. The pH point-of-zero charge (pHpzc) was obtained as described [Citation28], while the slurry pH was determined by soaking 1 g of the adsorbent in 50 mL of distilled water with stirring for 24 h and then filtering. The X-ray diffraction (XRD) of the adsorbents was determined in a Randicon MD 10 model, and a Fourier-transform infrared spectrophotometer (FTIR; Shimadzu 8400s) was used. The pore properties and Brunauer, Emmett and Teller (BET) surface area of the adsorbents were determined from nitrogen adsorption–desorption isotherms in a micromeritics accelerated surface area and porosimetry system 2010 model analyser, while scanning electron microscopy (SEM; Hitachi S4800) was used to assess the morphology of the adsorbents.
2.4 Batch adsorption
All the reagents used in the study were of analytical grade obtained from Sigma–Aldrich and were used without further purification. A binary stock solution of 1000 mg/L of Ni(II) and Mn(II) ions was prepared by dissolving appropriate amounts of NiSO4·6H2O and MnSO4·H2O in 1 L of double-distilled water. Working solutions of 100–500 mg/L were prepared from the stock solution by serial dilution. The pH of each solution was adjusted to 2.0–8.0 by drop-wise addition of 0.1 mol/L HCl or NaOH.
Batch adsorption was performed by addition of 0.1 g of the adsorbent to 50 mL of a solution of metal ions in a 100-mL pretreated plastic bottle at a room temperature of 300 K. To determine the effect of temperature, the bottles were placed in a thermo-stated water bath. The effects of the operating conditions pH (2.0–8.0), adsorbent dose (0.1–0.5 g), particle size (100–500 μm), contact time (20–300 min), initial metal ion concentration (100–500 mg/L) and temperature (300–323 K) were investigated. In each experiment, one parameter was varied while the others were kept constant. The solution was filtered at the end of a given contact time, and the concentration of metal ions remaining in the filtrate was determined by atomic absorption spectrophotometry. Each experiment was performed twice, and the mean value was computed to minimize error. The amount of metal ions adsorbed was calculated from the following equations:(1)
(1)
(2)
(2) where qe (mg/g) is the adsorption capacity of the adsorbent for the adsorbate at equilibrium, Co (mg/L) and Ce (mg/L) are the initial and equilibrium concentrations of metal ions in solution, respectively, m (g) is the mass of adsorbent, and V (L) is the volume of solution used.
3 Results and discussion
3.1 Adsorbent characterization
The physicochemical characteristics of the adsorbents are shown in . Silica and alumina were the major components of montmorillonite. Alkaline modification of unmodified clay led to a slight decrease in silica, from 47.32% to 42.98%, and an increase in alumina, from 25.91% to 26.25%, resulting in a decrease in the silica to alumina ratio from 1.83 to 1.64. The decrease in the concentration of silica with alkaline treatment is due to an etching reaction between silica and the strong base used. A similar result was reported previously [Citation23]. A feature of our study was use of both NaOH and KOH in the modification, as other researchers have used either one or the other but not both; we added KOH to further increase the adsorption capacity. NaOH helps to remove surface impurities, thereby increasing the porosity and surface area for metal adsorption [Citation29], while addition of KOH replaces Na+ ions by K+ ions, which may further enhance the surface porosity and adsorption potential of the adsorbent. At an initial metal concentration of 500 mg/L, pH 6.0, a contact time of 180 min, an adsorbent dose 0.1 g and a particle size of 100 μm, the adsorption potential of the clay increased from 117.45 to 125.95 mg/g for Ni(II) ions and from 102.76 to 111.95 mg/g for Mn(II) ions after KOH treatment. Further increases in the concentration of KOH or use of NaOH decreased the adsorption potential, perhaps due to excessive alkaline leaching of the clay structure, which may affect the porous nature of the adsorbent. KOH treatment has been reported to be effective for removing silica and increasing the pore volume and surface area of adsorbents [Citation30].
Table 1 Physicochemical characterization of the adsorbents.
The specific surface area of an adsorbent is important in determining its adsorption capacity, and a high value is desirable for optimum adsorption. In general, the higher the specific surface area of a material the higher its adsorption potential and vice versa, although not in all cases. Alkaline modification increased the specific surface area of the montmorillonite, as determined by the methylene blue absorption method, from 23.2 to 30.7 m2/g, and the BET (SBET) from 55.76 to 87.24 m2/g, which is desirable. It has been reported that the specific surface area of an adsorbent can differ significantly with the method of determination [Citation31,Citation32]. The SBET of unmodified clay (55.76 m2/g) was close to the value (61 ± 2 m2/g) determined by Macht et al. [Citation32] for montmorillonites, whereas a low SBET of 26.33 m2/g was reported by Guo et al. [Citation33]. The factors that contribute to variation in the SBET values of montmorillonites include the type and purity of the clay, the saturating cation, the out-gassing temperature and the general preparation of the sample. The increase in specific surface area and SBET is attributed to an increase in pore property due to the etching reaction between silica and a strong base [Citation34]. An increase in the total pore volume from 0.0688 to 0.0981 cm3/g and a decrease in the average pore diameter from 49.35 to 44.98 Ǻ was obtained after alkaline modification. The cation exchange capacity, the maximum quantity of total cations a clay can hold at a given pH that is available for exchange in a given solution, increased from 90.78 to 94.32 mEq/100 g after alkaline modification. The negative charges introduced by an OH group in the presence of fewer H+ ions under alkaline condition leads to a slight increase in cation exchange capacity [Citation35]. The high capacity of the alkaline-modified clay indicates that removal of Ni(II) and Mn(II) ions from solution also involves an ion exchange mechanism.
The slurry pH and the pHpzc of adsorbents usually give useful information about surface oxygen complexes and the electronic surface charge of an adsorbent arising from the interaction between the adsorbent surface and the aqueous solution. The complexes on adsorbent surfaces are generally classified as acidic, basic or neutral. The slurry pH indicated that the adsorbents (unmodified and alkaline-modified montmorillonite clay) were slightly acidic (pH 4.2 and 5.8, respectively). There is a relation between the pHpzc and the adsorption capacity of an adsorbent: when the solution pH is lower than the pHpzc, the surface of the adsorbent is positively charged, thereby favouring the adsorption of anionic species, while for pH values greater than the pHpzc, the surface is negatively charged thereby favouring the adsorption of cationic species [Citation36]. Alkaline modification resulted in an increase in pHpzc from 3.7 to 5.2. This increase does not imply that alkaline-modified clay has a lower potential or affinity to adsorb metal ions from a solution but that optimum adsorption of metal ions is most likely to be achieved at pH values higher than 5.2, when the surface of the adsorbent is negatively charged.
3.2 FTIR analysis
The FTIR spectra of unmodified and alkaline-modified montmorillonite clay were determined to identify the surface functional groups responsible for binding of Ni(II) and Mn(II) ions and the effect of alkaline modification (). The unmodified clay showed absorption at 3697.66 and 3620.51 cm−1, perhaps corresponding to the inner surface –OH stretching vibration of kaolinite, indicating the presence of kaolinite in the clay. The presence of outer surface –OH stretching was indicated by bands at 3441.12 and 3410.26 cm−1. An absorption band at 1627.97 cm−1 represents the OH bending vibration and can also be assigned to the –COO– symmetric stretching vibration [Citation37]. The outer OH stretching and the symmetric –COO– stretching vibrations indicate the presence of smectite [Citation38]. The Si–O bending vibration was represented by bands at 1114.89–1006.88 cm−1, while the stretching vibrations were observed at 796.63 and 694.4 cm−1. Absorption bands at 912.36 cm−1 correspond to the Al–O bending vibration, while bands at 536.23, 470.65 and 430.14 cm−1 represent Al–O–Si skeletal vibrations [Citation39,Citation40]. Alkaline modification of the adsorbent was indicated by the shift in absorption bands from 3441.12 and 3410.26 cm−1 to 3427.62 and 3389.04 cm−1, respectively, for the outer –OH stretching. Shifts in –OH bending from 1627.97 to 1629.9 cm−1, Si–O bending from 114.89, 1097.53 and 1006.88 cm−1 to 1112.96, 1101.39 and 1008.8 cm−1, respectively, and the Al–O–Si vibration from 470.65 to 472.58 cm−1 were observed. The FTIR spectra showed that the aluminol and silanol groups are major sites for binding of positively charged ions onto alkaline-modified montmorillonite clay.
3.3 XRD and SEM analysis
The XRD spectra of unmodified and alkaline-modified clay are shown in . The d-spacing values of unmodified clay indicate that montmorillonite is the major constituent, with quartz, kaolinite and gibbsite. The sharp increase in the intensity of the montmorillonite peak in alkaline-modified clay suggests that the leached silica came from the silica phase and not the montmorillonite phase. XRD gave useful information about crystalline and amorphous changes of an adsorbent. The presence of sharp and weak peaks suggests an amorphous adsorbent [Citation41], and the amorphous nature of alkaline-modified clay indicates that Ni(II) and Mn(II) ions in solution can easily penetrate its surface for efficient adsorption [Citation42].
SEM analysis of unmodified and alkaline-modified clay is shown in , which revealed the porous nature of the adsorbents and an increase in surface porosity with alkaline modification. The more porous nature of alkaline-modified clay signifies enhanced sorption potential for metal ions, such that physical adsorption would play a major role in the overall sorption process [Citation43].
3.4 Effect of initial metal ion concentration
The amount of metal ions adsorbed is a function of the initial concentration of the adsorbate (metal ion), making it an important factor in effective adsorption. The effect of initial metal ion concentration on the adsorption of Ni(II) and Mn(II) ions onto unmodified and alkaline-modified montmorillonite clay is shown in . The adsorption capacity of both adsorbents for metal ions increased with the metal concentration, as the increasing concentration gradient overcomes the resistance to mass transfer of metal ions between the aqueous phase and the adsorbent [Citation17]. A higher concentration in a solution implies a higher concentration of metal ion to be fixed on the surface of the adsorbent [Citation4]. Alkaline-modified clay had a higher adsorption capacity for both metal ions than unmodified clay, indicating the effectiveness of alkaline modification. At a concentration of 500 mg/L, 125.95 mg/g of Ni(II) and 111.95 mg/g of Mn(II) ions were adsorbed by alkaline-modified clay, while unmodified clay adsorbed 110.45 mg/g Ni(II) and 89.4 mg/g Mn(II). The higher adsorption by alkaline-modified clay may be due to its increased surface area and better cation exchange capacity as compared with unmodified clay. Both unmodified and alkaline-modified clay showed greater adsorption of Ni(II) than Mn(II) ions, perhaps due to the smaller ionic radius of Ni(II) (0.72 Ǻ), which makes its sorption easier than that the larger Mn(II) ions, with an atomic radius of 0.80 Ǻ.
3.5 Effect of pH
The initial pH of a solution is another important parameter in adsorption, as metal ion removal usually increases with increased pH. The pH of a solution affects the degree of ionization of the adsorbate and the surface properties of the adsorbent [Citation17]. The effect of initial pH of the solution on the percentage removal of Ni(II) and Mn(II) ions onto alkaline-modified clay is shown in . Adsorption of both metal ions increased with increasing pH, with significant adsorption at pH 6–8, which is higher than the pHpzc of 5.2 of alkaline-modified clay, when the surface becomes negative. An optimum pH of 6.0 was selected and used for all experiments in order to avoid the formation of metal hydroxide precipitates at higher pH. At lower pH, higher concentrations of H+ ions are present in solution, which creates a competition between the protons and metal ions for the active sites of the adsorbent. The low adsorption at low pH was thus due to saturation of the active sites of alkaline-modified clay with hydrogen ions. The increased removal of Ni(II) and Mn(II) ions with increasing pH is also due to less competition between protons and metal ions for active sites and a decrease in positive surface charge, which results in less electrostatic repulsion between the surface and metal ions before ion exchange [Citation5].
3.6 Effect of adsorbent dose
Adsorbent dosage is a useful parameter for determining the capacity of an adsorbent for a given initial adsorbate concentration. The effect of alkaline-modified clay dose on adsorption of Ni(II) and Mn(II) ions from solution is shown in ; the percentage removal of both metal ions increased with increasing dosage due mainly to an increase in the number of available active sites [Citation14]. The equilibrium adsorption capacity per unit mass of alkaline-modified clay decreased considerably with increase in sorbent dose for both metal ions: increasing the dose from 0.1 to 0.5 g decreased the equilibrium adsorption capacity from 34.85 to 8.51 mg/g for Ni(II) and from 32.35 to 8.05 mg/g for Mn(II). This may be due to a decrease in the total adsorption surface area available, resulting from overlapping or aggregation of adsorption sites [Citation37], or a higher adsorbent dose may provide more active adsorption sites, so that adsorption sites remain unsaturated during adsorption [Citation44].
3.7 Effect of particle size
The effect of adsorbent particle size on percentage removal of Ni(II) and Mn(II) from solution onto alkaline-modified clay is shown in . A slight decrease in the removal of both metal ions was seen with increasing particle size. Thus, increasing the particle size from 100 to 500 μm decreased the removal from 69.7% to 55.8% for Ni(II) and from 64.7% to 50.6% for Mn(II). This decrease is attributable to a decrease in the surface area of adsorbent available for metal ions. The breaking up of larger particles into smaller ones tends to open tiny cracks and channels on the particle surface of the adsorbent, resulting in greater accessibility and better diffusion [Citation45]. Similar results have been reported previously [Citation4,Citation46].
3.8 Equilibrium isotherm modelling
Equilibrium adsorption isotherms are used to relate the adsorbate concentration in solution and the amount on the adsorbent at equilibrium [Citation47]. These parameters often provide fundamental information on the sorption mechanism, surface properties and the affinity of adsorbents, which helps to determine the applicability of sorption as a unit operation. Therefore, it is important to establish the most suitable correlation of equilibrium curves in order to optimize the conditions for designing adsorption systems. The most frequently used isotherms, the Langmuir, Freundlich, Temkin and Dubinin–Radushkevich models, were therefore used to analyse the data ().
Table 2 Equilibrium isotherm parameters.
The Langmuir isotherm is used to describe monolayer adsorption onto the surface of an adsorbent with a finite number of identical adsorption sites and no interaction between sites. The model is expressed as [Citation48]:(3)
(3) where qL (mg/g) is the monolayer adsorption capacity of the adsorbent, and KL (L/mg) is the adsorption constant, which reflects the affinity between the adsorbent and adsorbate. qL and KL were determined from the slope and intercept of the plots of Ce/qe versus Ce. An essential feature of the Langmuir isotherm can be expressed in terms of a dimensionless separation factor (RL), defined as:
(4)
(4)
Adsorption is said to be favourable if 0 < RL < 1 and unfavourable if RL > 1. shows low correlation coefficients (r2) for Ni(II) and Mn(II) (<0.95), indicating that the adsorption does not follow the Langmuir isotherm closely. A favourable adsorption process was, however, indicated by the RL values, which lay within the range of 0.25–0.65 for Ni(II) and 0.33–0.71 for Mn(II) at all concentrations studied.
The Freundlich isotherm [Citation49] is based on the assumption that sorption takes place on a homogeneous adsorbent surface, where the sorption energy distribution decreases exponentially and can be expressed as:(5)
(5) where KF (mg/g)(mg/L)1/n and n are the constants for adsorption capacity and intensity, respectively. A plot of log qe versus log Ce gives a straight line of slope 1/n and intercepts log KF. As can be seen in , the r2 values for both Ni(II) and Mn(II) ions were high (>0.95), indicating a good fit of the Freundlich model to the adsorption process. This suggests that the surface of alkaline-modified montmorillonite clay is heterogeneous. Furthermore, the values of n obtained for both metal ions lie between 1 and 10, indicating favourable adsorption [Citation50].
The Temkin isotherm is based on the assumption that the free energy of adsorption is a function of the surface coverage; it is expressed as [Citation51]:(6)
(6) where B = RT/b (mg/g) is the isotherm constant related to the heat of adsorption, and A (L/g) is the equilibrium binding constant corresponding to the maximum binding energy, R is the gas constant and T (K) is absolute temperature. The constants A and B were determined from the plot of qe versus ln Ce. The r2 values obtained () for both metal ions showed a poor fit for the data.
The Dubinin–Radushkevich isotherm does not assume a homogeneous surface or a constant adsorption potential and is expressed as [Citation52]:(7)
(7) where qm (mg/g) is the theoretical saturation capacity, β (mol2/J2) is a coefficient related to the mean free energy of adsorption, and ɛ is the Polanyi expressed as:
(8)
(8)
The constants qm and β were calculated from the intercept and slope of ln qe versus ɛ2. The isotherm did not provide a good fit for either metal ion, as indicated by the r2 values. Therefore, the adsorption of Ni(II) and Mn(II) ions onto alkaline-modified montmorillonite clay conformed best to the Freundlich adsorption model.
The differences in the adsorbed amounts deduced from the four models are simply a result of the different equations for analysing the experimental data. Similar results have been reported by many researchers [Citation4,Citation5,Citation8,Citation13,Citation14,Citation20,Citation29].
3.9 Effect of contact time
The effect of contact time on the adsorption of Ni(II) and Mn(II) ions onto alkaline-modified montmorillonite clay is shown in . An initial increase in percentage removal with increased contact time was observed, and adsorption became fairly stable over time. Equilibrium removal was achieved around 120 min for Ni(II) and 160 min for Mn(II), after which further increases in contact time did not result in significant adsorption. Maximum adsorption was achieved at 180 min for both metal ions, and this time was used in all experiments to ensure maximum removal. The faster removal rate with Ni(II) than with Mn(II) may be due to the smaller ionic radius of Ni(II) (0.72 Ǻ) than Mn(II) (0.80 Ǻ), which makes for easier, more rapid diffusion to the surface of alkaline-modified clay. The rapid adsorption during the initial stages might be due to the presence of abundant active sites on the surface of alkaline-modified clay, which become saturated with time. Initially, sorption is controlled mainly by diffusion from the bulk to the surface of the clay, whereas, later, sorption is probably an attachment-controlled process due to the presence of fewer active sites [Citation17].
3.10 Kinetic modelling
The mechanism controlling adsorption was investigated with pseudo-first order, pseudo-second order, Elovich and intraparticle diffusion rate equations. The kinetic parameters are shown in . The pseudo-first order or Lagergern equation is based on the assumption that the rate of adsorption site occupation is proportional to the number of unoccupied sites [Citation53]. The linear form of the Lagergern equation is:(9)
(9) where KI (min−1) is the rate constant, and qt and qe are the amounts of metal ion adsorbed at time t and equilibrium, respectively. The constant KI and qecal were obtained from the slope and intercept of the plot of log(qe − qt) versus t. The r2 values () obtained for the two metal ions showed a poor fit.
Table 3 Kinetic parameters for the adsorption of Ni(II) and Mn(II) unto KMUC.
The pseudo-second order model assumes that the rate of occupation of adsorption sites is proportional to the square of the number of unoccupied sites [Citation54]. The linear form of the pseudo-second order equation is:(10)
(10) where K2 (g/mg min) is the constant. The initial sorption rate h was calculated from the equation:
(11)
(11)
If the pseudo-second order equation is applicable, the plot of t/qt versus t yields a straight line. K2 and qe were calculated from the slope and intercept of the plot. This model provided a good fit to the experimental data for both metal ions, as seen from the r2 values (); however, the calculated qe values showed wide discrepancy from experimental values, indicating that adsorption does not follow the pseudo-second order model closely.
The kinetic data were analysed further with the Elovich equation, which is expressed in its linear form as [Citation8]:(12)
(12) where α (mg/g min) is the initial sorption rate constant, and β (g/mg) is a constant corresponding to the surface coverage and activation energy for chemisorption. This equation was applied by a linear plot of qt versus ln t, which showed conformity of the data to this model for both ions (), with good r2 values of 0.979 and 0.980 for Ni(II) and Mn(II) ions, respectively.
The diffusion mechanism was further analysed with the intraparticle diffusion rate equation, expressed as [Citation55]:(13)
(13) where Kd (mg/g min1/2) is the intraparticle diffusion rate constant and C is the intercept. A linear plot of qt versus t1/2 was used to obtain the constants Kd and C. Intraparticle diffusion is the sole rate-controlling step if the plot is linear and passes through the origin (C = 0). shows the r2 values obtained for the two metal ions, indicating the existence of an intraparticle diffusion mechanism, although it is not the sole rate-controlling step (C ≠ 0). Occurrence of the intercept C shows the existence of a boundary layer effect, indicating a surface phenomenon such as mass transfer or liquid film diffusion in the sorption process.
3.11 Thermodynamic studies
The influence of solution temperature on the adsorption of Ni(II) and Mn(II) ions from solution by alkaline-modified clay is shown in . A slight increase in the adsorption of both ions was seen with an increase in temperature from 300 K to 323 K. The percentage adsorbed increased from 69.7% to 77.9% for Ni(II) and from 64.7% to 74.2% for Mn(II), due perhaps to acquisition of more kinetic energy to overcome the energy barrier and the creation of more active sites on the adsorbent due to dissociation of surface components on the alkaline-modified clay [Citation37].
Fig. 9 Effect of temperature on percentage removal of metal ions from solution onto alkaline-modified montmorillonite clay (concentration 100 mg/L, pH 6.0, adsorbent dose 0.1 g, contact time 180 min, particle size 100 μm).
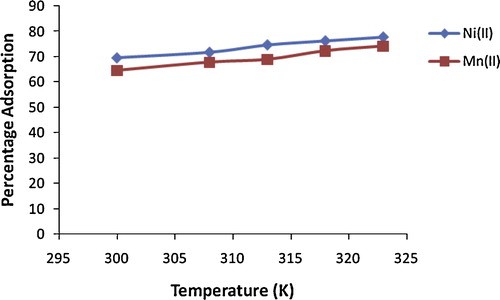
Thermodynamic parameters such as the standard free energy change (ΔG°), enthalpy change (ΔH°) and entropy change (ΔS°) were calculated to evaluate the feasibility of the adsorption process from the following equations [Citation22]:(14)
(14)
(15)
(15)
(16)
(16) where Kc is the thermodynamic equilibrium constant, Ca (mg/L) is the concentration of metal ions adsorbed at equilibrium, Ce (mg/L) is the concentration of metal ions remaining in solution at equilibrium, R is the ideal gas constant (8.314 J/mol K), and T (K) is the absolute temperature. The values of ΔH° and ΔS° were estimated from the slope and intercept of the plot of ln Kc versus 1/T. The thermodynamic parameters obtained are presented in .
Table 4 Adsorption thermodynamic parameters.
Negative values of ΔG° were obtained at all temperatures for both metal ions, indicating spontaneous adsorption. The positive values of ΔH° obtained reveal an endothermic adsorption process, which is supported by the increased adsorption with increasing temperature. The positive values of ΔS° reveal increasing randomness at the solid–solution interface [Citation56], and the low ΔS° values suggest no remarkable change in entropy. The magnitude of ΔH° provides information about the type of adsorption. The heat evolved during physical adsorption lies within the range 2.1–20.9 kJ/mol, while that of chemisorption is in the range 80–200 kJ/mol [Citation57]. The magnitude of ΔH° obtained indicates physical adsorption between the metal ions and the adsorbent ().
4 Conclusion
The results show that alkaline modification of montmorillonite enhances its adsorption capacity for Ni(II) and Mn(II) ions. The FTIR spectra showed that certain functional groups are responsible for binding the metal ions from solution. SEM indicated an increase in the porosity of the alkaline-modified adsorbent as compared with the unmodified form, which enhances metal ion adsorption on alkaline-modified montmorillonite clay. The heterogeneous nature of the alkaline-modified clay was revealed by the good fit of the data to the Freundlich model, while thermodynamic studies revealed endothermic, spontaneous physical adsorption. These results indicate that alkaline-modified montmorillonite clay is potentially a low-cost adsorbent for the removal of Ni(II) and Mn(II) ions from solution.
Notes
Peer review under responsibility of Taibah University
References
- M.A.O.BadmusT.O.K.AuduB.U.AnyataRemoval of lead ions from industrial wastewaters by activated carbon prepared from periwinkle shellsTurkish J. Eng. Environ. Sci.312007251263
- A.K.MeenaG.K.MishraP.K.RaiC.RajagopalP.N.NagarRemoval of heavy metal ions from aqueous solution using carbon aerogel as an adsorbentJ. Hazard. Mater.1222005161170
- R.SinghN.GautamA.MishraR.GuptaHeavy metals and living systems: an overviewIndian J. Pharmacol.432011246253
- N.BarkaM.AbdennouriM.E.MakhfoukS.QourzalBiosorption characteristics of cadmium and lead onto eco-friendly dried cactus cladodesJ. Environ. Chem. Eng.12013144149
- S.R.TaffarelJ.RubioOn the removal of Mn(II) ions by adsorption onto natural and activated Chilean zeolitesMiner. Eng.222009336343
- E.DenkhauseK.SalnikovNickel essentiality, toxicity and carcinogenicityCrit. Rev. Oncol. Hematol.4220023556
- F.GodeE.PehlivanRemoval of chromium(III) from aqueous solution using lewatit S 100: the effect of pH, time, metal concentration and temperatureJ. Hazard. Mater.1362006330337
- S.LiangX.GuoN.FengQ.TianIsotherm, kinetics and thermodynamic studies of adsorption of Cu(II) from aqueous solution by Mg/K type orange peel adsorbentsJ. Hazard. Mater.1742010756762
- S.WangH.LiKinetic modeling and mechanism of dye adsorption on unburned carbonDyes Pigments722007308314
- M.J.IqbalM.N.AshiqAdsorption of dyes from aqueous solution on activated charcoalJ. Hazard. Mater.13920075766
- L.TofanC.PaduraruI.VolfMaize bran as a low-cost resource for Cu(II) ions removalCellulose Chem. Technol.452011275280
- H.BarreraF.Urena-NunezB.BilyeuC.Barrera-DiazRemoval of chromium and toxic ions present in mine drainage by Ectodermis opuntiaJ. Hazard. Mater. B1362006846853
- G.H.PinoL.M.S.MesquitaM.L.ToremG.A.S.PintoBiosorption of cadmium by green coconut shell powderMiner. Eng.192006380387
- U.A.GulerM.SariogluSingle and binary biosorption of Cu(II), Ni(II) and methylene blue by raw and pretreated Spirogyria sp: equilibrium and kinetic modelingJ. Environ. Chem. Eng.12013369377
- S.WangZ.NanY.LiZ.ZhaoThe chemical bonding of copper ions on kaolin from Suzhou, ChinaDesalination2492009991995
- F.A.DawoduG.K.AkpomieI.C.OgbuApplication of kinetic rate equations on the removal of copper(II) ions by adsorption unto aloji kaolinite clay mineralInt. J. Multidisc. Sci. Eng.320122126
- B.DasN.K.MondalCalcareous soil as a new adsorbent to remove lead from aqueous solution: equilibrium, kinetic and thermodynamic studyUniv. J. Environ. Res. Technol.12011515530
- S.LarousA.H.MeniaiM.Bencheikh LehocineExperimental study of the removal of copper from aqueous solution by adsorption using sawdustDesalination1852005483490
- O.AbollinoM.AcetoM.MalandrinoC.SaizaniniE.MentastiAdsorption of heavy metals on Na-montmorillonite: effect of pH and organic substancesWater Res.37200316191627
- F.BarbierG.DucM.Petit-RamelAdsorption of lead and cadmium ions from aqueous solution to the montmorillonite/water interfaceColloid Surf. A1662000153159
- A.M.L.KraepielK.KellerF.M.M.MorelA model for metal adsorption on montmorilloniteJ. Colloid Interface Sci.21019994354
- K.G.BhattacharyyaS.S.GuptaKaolinite, montmorillonite and their modified derivatives as adsorbents for removal of Cu(II) from aqueous solutionSep. Purif. Technol.502006388397
- G.JozefaciukG.BowankoEffect of acid and alkali treatment on surface area and adsorption energies of selected mineralsClay Miner.502002771783
- F.KooliY.LiuS.X.TanJ.ZhengOrganoclays from alkaline-treated acid-activated claysJ. Therm. Anal. Calorimetry115201414651475
- S.KumarA.K.PandaR.K.SingPreparation and characterization of acids and alkali treated kaolin clayBull. Chem. React. Eng. Catal.820136169
- J.C.SantamarinaK.A.KleinY.K.WangE.PrenckeSpecific surface: determination and relevanceCan. Geotech. J.39200211611171
- J.D.RhoadesCation exchange capacityMethods of Soil Analysis, Part 2. Chemical and Microbial Properties2nd ed.1982American Society of Agronomy/Soil Science of AmericaHadison, Wisconsin
- M.S.OnyangoY.KojimaO.AoyiE.C.BernardoH.MatsudaAdsorption equilibrium modeling and solution chemistry dependence of fluoride removal from water by trivalent cation-exchange zeoliteJ. Colloid Interface Sci.2792004341350
- X.YangX.CuiAdsorption characteristics of Pb(II) on alkali treated tea residueWater Res.32013110
- R.K.GautamA.MudhooG.LofranoM.C.ChattopadhyayaBiomass derived biosorbents for metal ions sequestration: adsorbent modification and activation methods and adsorbent regenerationJ. Environ. Chem. Eng.22014239259
- Y.H.ShenEstimation of surface area of montmorillonite by ethylene oxide chain adsorptionChemosphere48200210751079
- F.MachtK.EusterhuesG.J.PronkK.U.TotscheSpecific surface area of clay minerals: comparison between atomic force microscopy measurement and bulk-gas (N2) and liquid (EGME) adsorption methodsAppl. Clay Sci.5320112026
- Z.GuoY.LiS.ZhangH.NiuZ.ChenJ.XuEnhanced sorption of radiocobalt from water by Bi(III) modified montmorillonite: a novel adsorbentJ. Hazard. Mater.1922011168175
- W.T.TsaiK.J.HsienC.W.LaiChemical activation of spent diatomaceous earth by alkaline etching in the preparation of mesoporous adsorbentsInd. Eng. Chem. Res.43200475137520
- S.A.CardenaT.G.VelazquezG.O.RevillaL.S.CortezStudy of the surface charge of a porous clay heterostructure and its adsorption capacity of alkaline metalsJ. Mex. Chem. Soc.5420109297
- S.M.NomanbhayK.PalanisamyRemoval of heavy metals from industrial wastewaters using chitosan coated oil palm shell charcoalElect. J. Biotechnol.820054353
- Y.LiB.XiaQ.ZhaoF.LiuP.ZhangQ.J.Duet al.Removal of copper ions from aqueous solution by calcium alginate immobilized kaolinJ. Environ. Sci.232011404411
- G.E.EkosseFourier transform infrared spectrophotometery and X-ray powder diffractometry as complementary techniques in characterizing clay size fraction of kaolinJ. Appl. Sci. Environ. Manage.920054348
- R.K.VempatiM.Y.A.MollahG.R.ReddyD.L.CockeIntercalation of kaolinite under hydrothermal conditionsJ. Mater. Sci.31199612551259
- A.NjoyaC.NkoumbouC.GrosboisD.NjopwouoD.NjoyaA.Courtin-Nomadeet al.Genesis of mayouom kaolin deposit (western Cameroon)Appl. Clay Sci.322006125140
- M.KobyaE.DemirabisE.SenturkM.InceAdsorption of heavy metal ions from aqueous solution by activated carbon prepared from apricot stoneBiores. Technol.96200515181521
- V.T.P.VinodR.B.SashidharA.A.SukumarCompetitive adsorption of toxic heavy metal contaminants by gum kondagodu: a natural hydrocolloidColloid Surf. B752010490495
- S.VafakhahM.E.BahrololoomR.BazarganlariM.SaeedikhaniRemoval of copper ions from electroplating effluents solution with native corn cob and corn stalk and chemically modified corn stalkJ. Environ. Chem. Eng.22014356361
- C.RajiT.S.AnirudhanKinetics of Pb(II) adsorption by polyacrylamide grafted sawdustIndian J. Chem. Technol.41997157162
- G.KarthikeyanK.AnbalaganN.A.MuthulashimiAdsorption dynamics and equilibrium studies of Zn(II) onto chitosanJ. Chem. Sci.1162004119127
- S.Y.QuekD.A.J.WaseC.F.ForsterThe use of sago waste for the sorption of lead and copperWater SA241998251256
- E.AyranciO.DumanAdsorption behaviors of some phenolic compounds onto high specific area activated carbon clothJ. Hazard. Mater.1242005125132
- I.LangmuirThe adsorption of gases on plane surfaces of glass, mica and platinumJ. Am. Chem. Soc.40191813611368
- H.M.F.FreundlichOver the adsorption in solutionJ. Phys. Chem.571906385471
- F.SlejkoAdsorption Technology: A Step by Step Approach Process1985Marcel DekkerNew York
- M.J.TemkinV.PyzhevKinetics of ammonia synthesis on promoted iron catalystActa Physicochim. URSS121940217256
- M.M.DubininE.D.ZaverinaL.V.RadushkevichSorption and structure of active carbons: adsorption of organic vaporsJ. Phys. Chem.21194713511362
- S.LagergrenAbout the theory of so-called adsorption of soluble substancesK. Sven. Ventenskapsakad. Handl.Band 241898139
- Y.S.HoReview of second order models for adsorption systemsJ. Hazard. Mater. B1362006681689
- W.J.WeberJ.C.MorrisKinetics of adsorption on carbon from solutionJ. Saint. Eng. Div. Am. Soc. Civ. Eng.8919633160
- Y.NuhogluE.MalkocThermodynamic and kinetic studies for environmentally friendly Ni(II) biosorption using waste pumice of olive oil factoryBiores. Technol.100200923752380
- Y.LiuY.J.LiuBiosorption isotherms, kinetics and thermodynamicsSep. Purif. Technol.612008229242