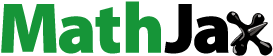
Abstract
Borate glasses with the composition xK2O-(25-x)Na2O-12.5MgO-12.5BaO-50B2O3 (x = 0, 5, 10, 15, 20 and 25 mol%) were prepared by the melt-quenching technique and were characterized using X-ray diffraction at room temperature. From the optical absorption spectra, the direct forbidden, direct allowed, indirect allowed, and indirect forbidden energy gaps; Urbach energy; refractive index (n); dielectric constant (ɛ); reflection loss (R); molar refraction (Rm); and molar polarizability (αm) values of all of the glass samples were evaluated. Rm, αm and Λth increase with the increase of the K2O content. The values of R, ɛ and n increase up to x = 10 mol% and then decrease above x = 10 mol%, which may be due to the mixed alkali effect. The density and molar volume values show the opposite behaviour and vary nonlinearly with the K2O content, which manifests the mixed alkali effect in the present glass system. The infrared (IR) spectra of the borate glasses reveal the existence of 3- and 4-coordinated boron atoms. The specific vibrations of the Na-O, K-O, Mg-O, and Ba-O bonds were observed in an IR study.
1 Introduction
In alkali oxide glasses, if the content of one alkali oxide is gradually replaced by another alkali oxide, keeping the total alkali oxide content constant, a nonlinear variation of some of the physical and optical properties is observed, which is called the mixed alkali effect (MAE) [Citation1]. MAE represents the nonlinear variation in density, molar volume, glass transition temperature, conductivity, band gap energy and dielectric constant properties, which are associated with the alkali ion movement. The addition of alkali oxides to pure borate glasses changes the boron coordination number from 3 to 4 and leads to the formation of non-bridging oxygens. The 4-fold coordinated boron atoms enhance the network rigidity by the crosslinking of the borate framework. In contrast, the non-bridging oxygens destroy the borate network. The negative charge of the network sites is compensated by the positive charge of alkali/alkaline earth oxide ions (M+/M2+). The alkali ions do not directly participate in the network formation and occupy interstitial voids. As a consequence, the structures of modified borate glasses are essentially built from the covalently bonded structural units. The ionic bonds are formed between M+, M2+ and B-O anionic units, and their bond strength depends on the specific alkali and alkaline cations. Infrared spectroscopy is used to investigate the structure of alkali borate glasses over the wide range and composition [Citation2–Citation4] of alkali oxides. The aim of this present work is to study the mixed alkali effect in various physical and optical properties of the glasses under investigation.
2 Experimental methods
The oxide borate glasses of xK2O-(25-x)Na2O-12.5MgO-12.5BaO-50B2O3 (x = 0, 5, 10, 15, 20 and 25 mol%) were prepared by the melt-quenching technique. The starting materials were Merck (GR grade) boric acid (H3BO3), potassium carbonate (K2CO3), sodium oxide (Na2O), magnesium oxide (MgO), and barium oxide (BaO). The calculated amounts by mol% of these compounds were thoroughly mixed and ground in an Agate mortar with a pestle. The ingredients were placed into a porcelain crucible and melted in an electrically heated furnace maintained at 1150 °C for 40 min. To obtain homogeneity, the melt was shaken frequently. The homogeneous melt was then quickly poured onto a stainless steel plate and pressed with another stainless steel plate, both maintained at 200 °C. The resulting glass samples were subsequently annealed at 300 °C for 6 h to relieve the strains. The glass samples thus obtained were clear, transparent and bubble free. All of the prepared glasses were used for further experimental studies. presents the chemical composition of the glasses studied. In the glass system studied, the alkaline earth oxide content was kept constant, while the alkali oxide content was varied.
Table 1 Composition (mole%) of the glass samples studied in the present work.
The X-ray diffractograms were recorded at room temperature with a 0.020/s scanning rate in the range (100–900) using the Philips X-ray diffractometer with copper Kα tube target and nickel filter operated at 40 kV, 30 mA. The optical absorption spectra of all of the glass samples were recorded with a (2092PIUV/VIS-Analytical Technologies Limited) UV–vis spectrometer in the wavelength region of 200–1000 nm. The Infrared Transmission spectra of all of the glass samples were recorded at room temperature by using a Perkin Elmer Frontier FTIR in the mid-IR range, i.e., 400–4000 cm−1. The KBr pallet technique was used to record the IR spectra. The sample was fine powdered and mixed with 0.3 g KBr in the ratio 2:100 and put into a 13 mm dye and pressed with a pressure of 7–8 tonnes using a hydraulic press to obtain transparent pallets with an approximate thickness of 1 mm. The spectra of these pallets were then recorded by using a universal sample holder with the resolution of 4 cm−1 and 16 scans per sample. The background removal and baseline correction were performed with the help of Spectrum 10 software.
3 Results and discussion
3.1 Physical properties of the mixed alkali borate glasses are as follows
The densities of all of the glass samples were estimated from the formula(1)
(1) where W1 and W2 are the weights of the glass sample in air and xylene, respectively, and 0.86 g/cm3 is the density of the xylene at room temperature. The molar volume of the glass composition was calculated using the formula,
(2)
(2) where xi is the molar fraction, Mi is the molecular weight of the ith component of the glass and ρ is the density of the glass. The molar volume relates directly to the spatial distribution of the oxygen in the glass network. The density and molar volume values are given in . shows the room temperature density (ρ) and molar volume (Vm) variation as a function of K2O content. It is found that the density and molar volume show opposite behaviour and vary nonlinearly with the K2O content, which manifests the mixed alkali effect in the present glass system.
Table 2 Physical and optical parameters KNMBB glass system.
The theoretical optical basicity (Λth) of all of the glass sample values were evaluated by using the following equation [Citation5](3)
(3) where xK2O, xNa2O, xMgO, xBaO and xB2O3 are the equivalent fractions of different oxides in mol%, i.e., the proportion of oxide atoms they contribute to the stoichiometry of the glass. The values of optical basicity for the individual oxides are
,
, ΛMgO = 0.078, ΛBaO = 1.15 and
[Citation6]. The theoretical optical basicity (Λth) values are given in . The Λth values increase with increasing K2O content. The variations in the refractive index and optical basicity parameter as a function of K2O content are shown in . It was observed that the variation with K2O content is nonlinear, which may be attributed to the mixed alkali effect.
3.2 Optical absorption spectra
The optical absorption spectra for all of the samples of KNMBB glasses were recorded at room temperature and are shown in . The disorder in the optical absorption (OA) intensities is observed. In the glasses studied, the network modifiers are the alkaline earth oxides (MgO, BaO) and the alkali oxides (K2O, Na2O). The content of MgO and BaO was kept constant, while the content of K2O and Na2O was varied. In B2O3 glasses, the addition of network modifiers leads to the modification (breakage) of the glass network, creating non-bridging oxygens (NBOs). As the content of the alkali oxides is changed, there will be variation in the NBO concentrations. Therefore, it may be concluded that the disorder in OA intensities may be attributed to the variation of NBO concentrations. From the optical absorption spectra, the cut-off wavelength, direct forbidden, direct allowed, indirect allowed, and indirect forbidden energy gaps, and Urbach energy values are evaluated, and the results are presented in . The optical absorption coefficient α(ϑ) near the fundamental absorption edge of the curve was determined from the relation(4)
(4) where d is the thickness of each sample, and the factor
corresponds to the absorbance (A). I0 and It are the intensities of the incident and transmitted beams, respectively. For amorphous materials, the relation between α(ϑ) and the phonon energy of incident radiation hϑ follows the Davis and Mott [Citation7] and Tauc and Menth [Citation8] relation
(5)
(5) where ‘n’ is the index, which can have the values 1/3, 1/2, 2 and 3, corresponding to direct forbidden, direct allowed, indirect allowed and indirect forbidden transitions, respectively. Here, B is a constant called the band tailing parameter, Eopt is the optical band gap energy, and hϑ is the incident photon energy. The Tauc's plots for different glass samples were obtained by plotting (αhϑ)1/2 and (αhϑ)1/3 as a function of the photon energy hϑ for n = 2 and n = 3, which correspond to the indirect allowed and indirect forbidden transitions and are shown in and , respectively. The values of Eopt were calculated from the linear regions of these curves, which were extrapolated to meet the hϑ axis at (αhϑ)1/2 = 0 for indirect allowed and (αhϑ)1/3 = 0 for indirect forbidden band gaps energies, and are listed in for all of the compositions. The variation of energy Eopt for n = 2 and n = 3 with K2O content is shown in . The variation is nonlinear, which may due to the mixed alkali effect. For amorphous materials, the value of the absorption coefficient α(ϑ) is given by
(6)
(6) where C is a constant and ΔE is the Urbach's energy [Citation9]. The exponential tail is due to phonon-assisted indirect electronic transitions [Citation6]. shows the variation of ln(α) as a function of photon energy hϑ, while the inset shows the nonlinear variation in ΔE with respect to the mol% of K2O content. This is an indication of the presence of the mixed alkali effect in the glass matrix. The Urbach energy values are presented in .
3.2.1 Refractive index (n), dielectric constant (ɛ)
The refractive index (n) of all the glass samples values were evaluated from the optical band gap energy (Eopt) values using the relation proposed by Dimitrov and Sakka [Citation6](7)
(7)
The optical dielectric constants of the present glass system values were calculated from the refractive index value of each glass using the following equation [Citation10].(8)
(8)
The calculated refractive index (n) and dielectric constant (ɛ) values are listed in . shows the nonlinear variation in dielectric constant as a function of K2O content, which may be due to the mixed alkali effect.
3.2.2 Reflection loss (R), molar refraction (Rm) and molar polarizability (αm)
The reflection loss (R) values were evaluated from the refractive index of the glasses using Fresnel's formula [Citation11].(9)
(9)
Molar refraction is the one of the parameters related to the glass structure and can be calculated by the Lorentz–Lorenz equation as in [Citation12–Citation15](10)
(10)
The ratio is called the polarizability per unit volume. According to the Herzfeld theory of metallization [Citation11], prediction of the present glasses as metallic or insulator is based on the metallization criterion (M), which is given as
If
, the material exhibits a metallic nature, and if
, the material is treated as having an insulating nature. The metallization parameter values of the present glass system are given in . From these values, we concluded that all of the present glass samples behave as non-metals. The molar refraction for each glass was evaluated as a function of molar polarizability (αm) via
(11)
(11)
Using the values of N (Avogadro's number), this equation becomes(12)
(12)
From Eq. Equation(12)(12)
(12) , the molar polarizability values can be evaluated, the results of which are presented in . The variation in molar refraction as a function of the theoretical optical basicity is shown in . shows the variation of the reflection loss and molar polarizability as a function of K2O content, which exhibits the mixed alkali effect (MAE). The mixed alkali effect was also observed by other workers [Citation16,Citation17] in the physical properties and spectroscopic studies.
3.3 FT-IR spectra
The FT-IR transition spectra gives significant information about the molecular vibrations as well as rotation associated with a covalent bond. The broad bands are exhibited in the oxide spectra, most probably due to the combination of the high degeneracy of vibrational states and the thermal broadening of the lattice dispersion bands. Alkali or alkaline earth cations act as network modifiers, breaking bridging oxygen bonds to form non-bridging oxygen atoms (NBOs) and residing in sites interstitial to the tetrahedral network in the vicinity of the negatively charged NBOs. shows the infrared spectra of the present glass samples.
The assignments of the infrared spectra of all of the glasses arise largely from the modified borate networks and are mainly active in the spectral range of 400–1600 cm−1. From the IR spectra, the peaks can be categorized as sharp, medium and broad. In the infrared spectrum, (i) the profile in the wavenumber range from 400 to 800 cm−1 is assigned to the bending vibrations of various borate segments and vibrations of alkali and alkaline earth cations through the glass network, (ii) bands in the range 800–1200 cm−1 are due to B-O asymmetric stretching of tetrahedral BO4 units, and (iii) peaks approximately 1200–1600 cm−1 are the strong IR bands, which are generated by the stretching vibrations of borate units in which boron atoms are connected to three oxygen atoms in BO3 units [Citation18–Citation21]. Therefore, systematic changes in the infrared spectra of the glasses under study showed the presence of three principal broad bands at approximately 700, 1000 and 1390 cm−1, and these have shown a sharp deep band approximately 446 cm−1, 413 and 435 cm−1, 430 and 455 cm−1, 417 and 436 cm−1, 417, 437 and 453 cm−1 and 454 cm−1, corresponding to the KMBB6, KNMBB5, KNMBB4, KNMBB3, KNMBB2 and NMBB1 glass systems, which indicate the presence of different specific vibrations of K-O, Na-O, Mg-O and Ba-O as the alkali and alkaline cations at localized sites [Citation2,Citation22–Citation24]. Also observed are deep broad bands approximately 998 cm−1, 954 cm−1, 932 cm−1, 949 cm−1 and 988 cm−1, corresponding to KMBB6, KMBB5, KMBB3, KMBB2 and KMBB1 in glass systems only. The assignments of the peaks are listed in and are due to the B-O stretching vibration of tetrahedral BO4 units. We observed from the IR spectra that the peak positions appeared at the high-energy side for NMBB1 and low-energy side for KMBB6 glasses except approximately 700 cm−1, as sodium oxide (Na2O) has a lower atomic radius compared to potassium oxide (K2O). From these data, it can be observed that the IR band at ∼806 cm−1 is absent; this is an indication of the absence of boroxol rings in the present glass system, and hence the structure of the glasses consists of only BO3 and BO4 groups. shows the weak and broad bands and band assignments of all of the glass samples. It was observed that the intensity of the broad band at ∼710 cm−1 increases and shifts towards the higher wave number side as the concentration of K2O increases from 0 to 25 mol%, and the remaining weak and broad bands change very little with K2O concentration for all of the glass samples except x = 0 mol%, which is clearly depicted in the inset of and may be due to the change in concentration of non-bridging oxygens and expansion of the B-O network with x mol%.
Table 3 Observed IR bands and band assignments for the KNMBB glass system.
4 Conclusions
• | The density and molar volume values show opposite behaviour and vary nonlinearly with the K2O content, which manifests the mixed alkali effect in the present glass system. |
• | The theoretical optical basicity slightly increases with the increase of the K2O content. |
• | The indirect allowed and indirect forbidden energy gap values were evaluated corresponding to n = 2 and n = 3 in the present glass system. |
• | The values of Rm, αm and Λth increase with the increase of the K2O content. The values of R, ɛ and n increase up to x = 10 mol% and then decrease thereafter, which may be due to the mixed alkali effect. |
• | The FT-IR spectra of the present glass samples exhibited the three characteristic active vibrational modes of a borate network: (i) the profile in the wavenumber range from 400 to 800 cm−1 is assigned to the bending vibrations of various borate segments and vibrations of Na-O, K-O, Mg-O, and Ba-O cations through the glass network; (ii) bands in the range of 800–1200 cm−1 are due to B-O asymmetric stretching of tetrahedral BO4 units; and (iii) peaks at approximately 1200–1600 cm−1 are the strong IR bands, which are generated by the stretching vibrations of borate units in which boron atoms are connected to the three oxygen atoms in BO3 units. |
• | In the IR spectra, the intensity of the broad band at ∼710 cm−1 increases and shifts towards the higher wave number side as the concentration of K2O increases from 0 to 25 mol%, and the remaining weak and broad bands change very little with the K2O concentration, except x = 0 mol%, which may be due to the change in the concentration of non-bridging oxygens of the glass matrix. |
Acknowledgements
One of the authors, G. Srinivas, thanks the DST-PURSE program and UGC-New Delhi for financial assistance through the RFSMS (Junior Research Fellow) program and also thanks UGC DAE CSR, Indore center and CFRD, Osmania University, Hyderabad, for providing research facilities.
Notes
Peer review under responsibility of Taibah University.
References
- J.O.IsardMixed alkali effects in glassesJ. Non-Cryst. Solids11969235
- E.I.KamitsosA.P.PatsisM.A.KarakassidesG.D.ChryssikosJ. Non-Cryst. Solids126199052
- E.I.KamitsosM.A.KarakassidesG.D.ChryssikosPhys. Chem. Glasses281987203
- J.Krogh-MoePhys. Chem. Glasses6196546
- J.A.DuffyPhys. Chem. Glasses3019891
- V.DimitrovS.SakkaJ. Appl. Phys.7919961736
- E.A.DavisN.F.MottPhil. Mag.221970903
- J.TaucA.MenthJ. Non-Cryst. Solids81972569
- K.L.ChopraS.K.BahlThin Solid Films111972377
- J.E.ShelbyJ.RullerPhys. Chem. Glasses281987262
- K.PradeshC.J.OtonV.K.AgotiyaM.RaghavendraG.Vijaya PrakashOpt. Mater.312008155160
- H.A.LorentzAnn. Phys.91880641
- L.LorenzWied. Ann.11188170
- J.A.DuffyJ. Solid State Chem.621986145
- K.HerzfeldPhys. Rev.291927701
- M.A.SameeA.M.AwasthiT.ShripathiS.BaleCh.SrinivasuS.RahmanJ. Alloys Compd.509201131833189
- R.S.E.S.DawaudS.HashimY.S.M.AlajeramiM.H.A.MharebTamchek N.J. Mol. Struct.10752014113117
- A.A.Abou ShamaF.H.El-BatalEgypt. J. Solids291200649
- G.A.B.R.MohamedK.Abdel-Ati AliA.G.E.-D.MostafaTurk. J. Phys.31200731
- A.A.AlemiH.A.SedghiR.MirmohseniGolsanamluBull. Mater. Sci.291200655
- P.PascutaL.PopS.RadaM.BoscaE.CuleaJ. Mater. Sci. Mater. Electron.192008424
- G.PadmajaP.KistaiahJ. Phys. Chem.113200923972404
- R.A.CondrateVibrational spectra of structural units in glassJ. Non-Cryst. Solids8419862633
- Y.TetsujiK.NoboruS.ShuichiY.MasayukiJ. Non-Cryst. Solids3212003147156