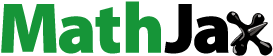
Abstract
A facile and economical hydrothermal method was used to synthesise Zn2SnO4 nanoparticles with cubic spinel structure. The crystallography and optical properties of the as-synthesised nanoparticles were studied using X-ray diffraction (XRD) and UV–visible spectroscopy (UV–vis). The morphology of the nanoparticles was observed using field emission scanning electron microscopy (FESEM). The synergistic antibacterial effect of Zn2SnO4 nanoparticles against Gram-positive and Gram-negative pathogenic bacteria was investigated. These results indicate that the Zn2SnO4 nanoparticles have potent antibacterial activity against both Gram-positive and Gram-negative bacteria and can be used as a bactericidal agent to prevent and control the spread and persistence of infectious diseases.
1 Introduction
Microbes and humans have forged a unique and largely beneficial relationship, but emerging infectious diseases continue to be one of the daunting challenges worldwide, leading to the upsurge in the investigation of nanomaterials. Given that the bacteria have developed resistance against many common antibacterial agents, there is a need for concrete advances in developing effective antibacterial therapeutic agents. Significant efforts have been directed towards the development of metal oxide nanoparticles, which are demonstrated to be effective for treating infectious diseases to combat antibiotic-resistant bacteria [Citation1,Citation2]. Recently, zinc-based stannate materials have attracted considerable attention due to their versatile applications in diverse fields, including photoelectrochemical cells, transparent conductive electrodes, Li-ion batteries, photocatalysts, and sensors [Citation3–Citation10]. Researchers have developed different techniques to synthesise zinc stannate nanostructures, which include solvothermal [Citation11], high-temperature calcination [Citation12], solution combustion [Citation13], precipitation method [Citation14], sol–gel [Citation15], and hydrothermal reaction [Citation5,Citation7,Citation16–Citation19]. The hydrothermal synthesis has been demonstrated beyond doubt to be the most efficient method for growing zinc stannate nanostructures with various morphologies, such as cubes, spheres, and rods, by varying the chemicophysical parameters. Although the antibacterial behaviour of many metal oxides has been investigated, the studies on the antibacterial potential of zinc stannate nanoparticles are still at a nascent stage. Considering the potential toxicity of metal oxide nanoparticles to pathogenic bacteria, we report the antibacterial efficacy of Zn2SnO4 nanoparticles prepared via hydrothermal method. In this study, we investigated their structural and optical properties and their antibacterial activity against Gram-positive (Staphylococcus aureus, Bacillus subtilis) and Gram-negative (Klebsiella pneumonia, Escherichia coli) bacteria. To that end, this report describes the first systematic study on the antibacterial properties of Zn2SnO4 nanoparticles.
2 Materials and methods
2.1 Materials
Zinc chloride, tin chloride pentahydrate and sodium hydroxide (NaOH) were purchased from Sigma Aldrich. Double distilled water is used throughout the experiment.
2.2 Preparation of Zn2SnO4 nanoparticles
Zn2SnO4 nanoparticles were synthesised via hydrothermal method using NaOH as a mineraliser. In a typical procedure, the ratio of Zn:Sn:NaOH is 2:1:8. Zinc chloride and tin chloride pentahydrate were completely dissolved in double-distilled water to form a transparent solution under magnetic stirring for 3 h. NaOH was subsequently added drop-wise into the mixture to form a white slurry. The precursor solutions were maintained at a pH of 8. After stirring for 30 min, the final mixture was transferred into a Teflon line stainless steel autoclave with a filling capacity of 80%. The autoclave was maintained at 200 °C for 24 h and cooled naturally to room temperature. The resulting nanoparticles were separated from the reaction solution by centrifugation and washed repeatedly with deionized water and ethanol. Finally, the washed particles were dried at 80 °C for 20 h to obtain the powdered sample.
2.3 Analysis of Zn2SnO4 nanoparticles
The optical absorption spectra of Zn2SnO4 nanoparticles were obtained using a Perkin Elmer Lambda 25 UV-Vis spectrometer in the wavelength range of 200–800 nm. The as-synthesised NPs were pressed into a pellet, and FT-IR spectrum of the pellet was recorded in the region 4000–400 cm−1. The X-ray diffraction patterns was recorded using a Rich Seifert diffractometer with monochromatic CuKα (λ = 1.5406 Å) radiation in the 2θ range of 10–80°. The morphology of the ZnO and Zn2SnO4 nanoparticles was observed using a FESEM (Field emission scanning electron microscopy).
2.4 Antibacterial assay
The antibacterial activities of the as-synthesised Zn2SnO4 nanoparticles were evaluated using the quantitative well diffusion assay. All glassware, media, and reagents used for this test were sterilised in an autoclave at 121 °C for 15 min. The Mueller Hinton agar was used as a test medium for antibacterial susceptibility testing. Gram-positive (S. aureus, B. subtilis) and Gram-negative (K. pneumonia, E. coli) bacteria were used as the model test strains. Microbes were streaked on the plate surface using a sterile cotton swab. A sterile cork borer (8 mm diameter) was used to make five wells. The four different concentrations (250, 500, 750, and 1000 μg) of Zn2SnO4 nanoparticle suspension were filled in the wells. The well plates were incubated at 37 °C for 24 h, and they were examined for the effectiveness of Zn2SnO4 nanoparticles by measuring the zone of inhibition.
3 Results and discussion
The powder XRD pattern of the as-synthesised sample is shown in . The diffraction pattern matches notably well with the standard JCPDS powder diffraction file no. 24-1470. The pattern reveals the formation of face-centered cubic spinel structure with the lattice constant a = 8.657 Å. In a hydrothermal process, the alkaline concentration is a key factor that influences the crystallinity, morphology, and size of the as-synthesised sample [Citation19]. The XRD pattern indicates that the reaction is complete and the as-synthesised nanoparticles are close to the expected stoichiometric ratio. The mean crystallite size was calculated using the Scherrer equation Equation(1)(1)
(1) , given as
(1)
(1) where D is the average grain size in nm, k (0.89) is a constant, λ is the X-ray wavelength, and θ and β are the diffraction angle and full-width at half-maximum of the observed peaks, respectively. From , the mean crystallite size is found to be 20.95 nm. Compared to other synthesis methods [Citation20], highly crystalline powders with narrow size distribution and high purity can be obtained via hydrothermal synthesis route without any post treatment at high temperature, which makes it an excellent method to synthesise Zn2SnO4 nanoparticles.
Table 1 Parameters calculated from XRD pattern of Zn2SnO4 nanoparticles.
UV–vis absorption spectrum was used to study the energy structures and optical properties of Zn2SnO4 NPs. The diffuse reflectance spectrum of Zn2SnO4 NPs shows a sharp increase in the reflectance at 300 nm, as seen in . The band gap energy was determined using the Kubelka–Munk function:(2)
(2) where h is the Planck's constant, ν is the frequency of vibration, Eg is the energy gap, A is the proportionality constant, and F(R∞) is the function of reflectance:
(3)
(3) where (R) is the absolute value of reflectance and F(R) is equivalent to the absorption coefficient. The direct band gap of Zn2SnO4 was estimated by plotting (F(R) · hν)2 vs hν (eV) as shown in the inset of . The optical band gap of zinc stannate nanoparticles is found to be 3.9 eV, which is apparently larger than that of bulk zinc stannate (3.6 eV). This blue shift in the optical band gap is due to the quantum confinement effect arising from the small size. The fundamental band gap of zinc stannate with a direct-forbidden transition is proposed to be 3.6–3.7 eV, but upon heating the band gap narrows down to 3.25 eV due to the incorporation of excess Zn into the Zn2SnO4 crystal.
FT-IR spectrum was recorded for zinc stannate in the range 400–4000 cm−1 and is shown in . A broad absorption peak observed at 530–591 cm−1 is due to the symmetric stretching vibration of ZnO and SnO2 groups, and this band could be assigned to the Sn–O–Zn bonding in the Zn2SnO4. The absorption peaks at ∼1600 and 3280 cm−1 indicate the presence of hydrogen bonds and the absorption peak at ∼1429 cm−1 is assigned to the C–H vibration mode attributed to the organic residuals. The peak at ∼2243 cm−1 is assigned to the possible bonding of Zn in ZnO and the peak ∼1170 cm−1 is attributed to the Sn–O stretching vibrations in Zn2SnO4. These observed peaks (at 530–591, ∼1170, and ∼2243 cm−1) are attributed to the formation of Zn2SnO4.
The FESEM analysis was used to identify the morphology of the synthesised Zn2SnO4NPs. The SEM image of Zn2SnO4 nanoparticles shown in reveals spherical morphology of the as-synthesised particles and the formation of network of aggregates. The growth of zinc stannate nanoparticles is initiated by the reaction between zinc ions and tin ions during hydrothermal synthesis. Apart from the reaction time and temperature, the concentration of sodium hydroxide in the precursor solution plays an important role in determining the size and shape of zinc stannate nanoparticles. Zinc hydroxystannate is transformed into zinc stannate at a medium concentration of sodium hydroxide.
3.1 Evaluation of antibacterial activity
In this study, the relative antibacterial activity of Zn2SnO4 nanoparticles with a mean crystallite size of 20.95 nm was investigated quantitatively by the well diffusion method. shows the four different concentrations of Zn2SnO4 nanoparticle suspension tested against Gram-positive (S. aureus, B. subtilis) and Gram-negative (K. pneumonia, E. coli) bacteria. Based on the observations (), the presence of an inhibition zone clearly indicates the biocidal action of Zn2SnO4 nanoparticles. The size of the inhibition zone increases significantly with the increasing concentration of NPs. Zn2SnO4 NPs have the maximum antibacterial efficacy against Gram-positive B. subtilis with the highest zone of inhibition of 19 mm followed by a zone of inhibition of 18 mm against S. aureus. Moderate antibacterial activity against Gram-negative K. pneumonia and E. coli with lower zone of inhibition of 12 mm is observed. The toxicity of Zn2SnO4 NPs depends on their concentration and these NPs are mildly toxic at low concentration. The mechanism of nanoparticle toxicity depends on composition, size, surface modification, intrinsic properties, and bacterial species. NPs attach to the bacterial cell membrane by electrostatic interaction and disrupt the integrity of bacterial cell, which in turn increases its permeability leading to cell death. The toxicity induced due to dissolved Zn ions and Sn ions from Zn2SnO4 nanoparticles is negligible and the toxicity strength of NPs depends on intrinsic toxic properties of heavy metals [Citation21].
Fig. 5 Antibacterial efficacy of Zn2SnO4 nanoparticles against Gram-positive (S. aureus, Bacillus subtilis) and Gram-negative (Klebsiella pneumonia, Escherichia coli) bacteria.
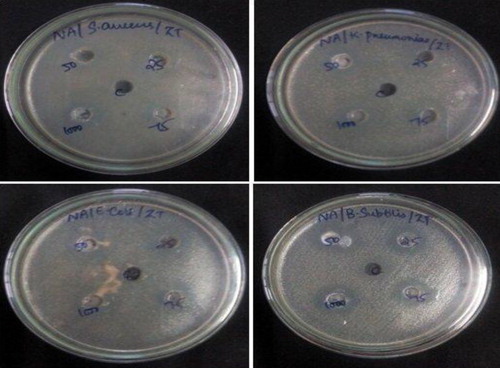
Table 2 Antibacterial efficacy of as-synthesised Zn2SnO4 nanoparticles against human pathogenic bacteria (NZ – no zone).
4 Conclusions
Spherical Zn2SnO4 nanoparticles were successfully synthesized by hydrothermal method under mild reaction condition. The lattice parameters calculated from X-ray diffraction pattern revealed the formation of face-centered cubic spinel structured Zn2SnO4 with a mean crystallite size of 20.95 nm. The band gap energy calculated from the diffuse reflectance spectrum is 3.9 eV for Zn2SnO4 nanoparticles, which is slightly higher than the bulk value of 3.6 eV for Zn2SnO4. FT-IR studies revealed the different functional groups present in the as-synthesised Zn2SnO4 and further confirmed the formation of zinc stannate nanoparticles. Based on the test results, we can conclude that Zn2SnO4 nanoparticles exhibit efficient antibacterial activity, which makes them a promising candidate for biomedical and pharmaceutical applications.
Competing interests
The authors declare that they have no competing interests.
Authors’ contributions
Emima and Allwin carried out experiments and wrote the manuscript. Emima, Allwin, Annie, Mary Jaculine, and Jerome Das discussed the experimental results and commented on the manuscript. All authors read and approved the final manuscript.
Acknowledgements
The authors are thankful to the management of Loyola College, Chennai for providing the necessary facilities to carry out this research work and also acknowledge Armats BioteK Lab for helping out in the antibacterial test.
Notes
Peer review under responsibility of Taibah University
References
- M.MiyauchiZ.LiuZ.-G.ZhaoS.AnandanK.HaraSingle crystalline zinc stannate nanoparticles for efficient photo electrochemical devicesChem. Commun.46201015291531
- P.K.StoimenovR.L.KlingerG.L.MarchinK.J.KlabundeMetal oxide nanoparticles as bactericidal agentsLangmuir18200266796686
- T.Lana-VillarrealG.BoschlooA.HagfeldtNanostructured zinc stannate as semiconductor working electrodes for dye-sensitized solar cellsJ. Phys. Chem. C111200755495556
- B.TanE.TomanY.LiY.WuZinc stannate (Zn2SnO4) dye-sensitized solar cellsJ. Am. Chem. Soc.129200741624163
- M.A.Alpuche-AvilesY.WuPhotoelectrochemical study of the band structure of Zn2SnO4 prepared by the hydrothermal methodJ. Am. Chem. Soc.131200932163224
- G.MaR.ZouL.JiangZ.ZhangY.XueL.YuG.SongW.LiaJ.HuPhase-controlled synthesis and gas-sensing properties of zinc stannate (ZnSnO3 and Zn2SnO4) faceted solid and hollow microcrystalsCrystEngComm14201221722179
- A.RongX.P.GaoG.R.LiT.Y.YanH.Y.ZhuJ.Q.QuD.Y.SongHydrothermal synthesis of Zn2SnO4 as anode materials for Li-ion batteryJ. Phys. Chem. B11020061475414760
- A.SivapunniyamN.WiromratM.T.Z.MyintJ.DuttaHigh-performance liquefied petroleum gas sensing based on nanostructures of zinc oxide and zinc stannateSens. Actuators B: Chem.1572011232239
- Z.LiY.ZhouJ.ZhangW.TuQ.LiuT.YuZ.ZouHexagonal nanoplate-textured micro-octahedron Zn2SnO4: combined effects toward enhanced efficiencies of dye-sensitized solar cell and photoreduction of CO2 into hydrocarbon fuelsCryst. Growth Des.12201214761481
- S.-H.ChoiD.HwangD.-Y.KimY.KervellaP.MaldiviS.-Y.JangR.DemadrilleI.-D.KimAmorphous zinc stannate (Zn2SnO4) nanofibers networks as photoelectrodes for organic dye-sensitized solar cellsAdv. Funct. Mater.23201331463155
- G.SunS.ZhangY.LiSolvothermal synthesis of Zn2SnO4 nanocrystals and their photocatalytic propertiesInt. J. Photoenergy2014 7 pp.
- J.-B.ShiP.-F.WuH.-S.LinY.-T.LinH.-W.LeeC.-T.KaoW.-H.LiaoS.-L.YoungSynthesis and characterization of single-crystalline zinc tin oxide nanowiresNanoscale Res. Lett.92014210
- P.JayabalaV.SasirekhaJ.MayandiV.RamakrishnanMicrowave assisted synthesis of zinc stannate nanocubes for dye sensitized solar cell applicationSuperlattices Microstruct.752014775784
- A.R.BabarS.B.KumbharS.S.ShindeA.V.MoholkarJ.H.KimK.Y.RajpureStructural, compositional and electrical properties of co-precipitated zinc stannateJ. Alloys Compd.509201175087514
- Y.ZhaoL.HuH.LiuM.LiaoX.FangL.WuaBand gap tunable Zn2SnO4 nanocubes through thermal effect and their outstanding ultraviolet light photoresponseSci. Rep.420146847
- X.LouX.JiaJ.XuS.LiuQ.GaoHydrothermal synthesis, characterization and photocatalytic properties of Zn2SnO4 nanocrystalMater. Sci. Eng. A4322006221225
- E.L.FolettoJ.M.SimõesM.A.MazuttiS.L.JahnE.I.MullerL.S.F.PereiraE.M.de Moraes FloresApplication of Zn2SnO4 photocatalyst prepared by microwave-assisted hydrothermal route in the degradation of organic pollutant under sunlightCeram. Int.39201345694574
- Z.ChenM.CaoC.HuNovel Zn2SnO4 hierarchical nanostructures and their gas sensing properties toward ethanolJ. Phys. Chem. C115201155225529
- V.ŠepelákS.M.BeckerI.BergmannS.IndrisM.ScheuermannA.FeldhoffC.KübelM.BrunsN.StürzlA.S.UlrichM.GhafariH.HahnC.P.GreyK.D.BeckerbkP.HeitjansNonequilibrium structure of Zn2SnO4 spinel nanoparticlesJ. Mater. Chem.22201231173126
- N.MuseeM.ThwalaN.NotaThe antibacterial effects of engineered nanomaterials: implications for wastewater treatment plantsJ. Environ. Monit.13201111641183
- G.ApplerotJ.LelloucheN.PerkasY.NitzanA.GedankenE.BaninZnO nanoparticle-coated surfaces inhibit bacterial biofilm formation and increase antibiotic susceptibilityRSC Adv.2201223142321