Abstract
Objective
The antimicrobial peptide hCAP18/LL-37 is detected in desquamated epithelial cells of human whole saliva, but the functional importance of this pool of hCAP18/LL-37 is not understood. Here, we assess the impact of homogenates of desquamated oral epithelial cells and exogenous, synthetic LL-37 on two oral bacteria: S. mutans and S. gordonii.
Material and methods
Desquamated epithelial cells of unstimulated whole saliva were isolated and cellular and extracellular levels of hCAP18/LL-37 analyzed by ELISA. Bacterial viability was determined by BacLight Live/Dead staining and confocal laser scanning microscopy.
Results
Desquamated oral epithelial cells harboured hCAP18/LL-37, and they spontaneously released/leaked the peptide to their medium. Exogenous, synthetic LL-37 showed cytotoxic activity against S. mutans but not S gordonii, suggesting that LL-37 acts differentially on these two types of oral bacteria. Homogenates of desquamated oral epithelial cells had no effect on S. mutans viability. Treatment with exogenous, synthetic LL-37 (8 and 10 μM) reduced S. mutans viability, whereas lower concentrations (0.1 and 1 µM) of the peptide lacked effect.
Conclusions
Desquamated oral epithelial cells contain hCAP18/LL-37, but their cellular levels of hCAP18/LL-37 are too low to affect S. mutans viability, whereas exogenous, synthetic LL-37 has a strong effect on these bacteria.
Introduction
The human antimicrobial peptide LL-37 and its proform hCAP18 are produced by white blood cells and epithelial cells [Citation1,Citation2]. The hCAP18 protein is encoded by the CAMP gene and cleaved extracellularly to LL-37 by serine protease 3 and kallikrein 5 [Citation3,Citation4]. LL-37 exerts its antibacterial activity via formation of pores in the bacterial cell wall and through binding and neutralization of bacterial endotoxins [Citation5–8]. Besides its antibacterial properties, LL-37 can also act as a chemoattractant for inflammatory cells and modulate NF-κB activity [Citation2,Citation9]. Interestingly, LL-37 has been shown to be active against many species of oral bacteria, both Gram-positive and Gram-negative, and the peptide is suggested to represent an important defense system against oral pathogens [Citation10,Citation11]. However, it has not been clarified if endogenous LL-37, found in the desquamated oral epithelial cells of whole saliva, can affect oral bacteria.
Whole saliva collected from both adults and children contains LL-37 [Citation12–15]. LL-37 and hCAP18 have been demonstrated in isolated human parotid and submandibular/sublingual saliva, implying that salivary LL-37, at least partly, originates from glandular tissue of the major salivary glands [Citation16]. Interestingly, vascular neutrophils of both parotid and submandibular glands express strong hCAP18/LL-37 immunoreactivity, suggesting that salivary LL-37 originates from these cells [Citation16]. Saliva has been shown to contain serine protease 3, indicating that hCAP18 can be cleaved and processed to active LL-37 in the saliva [Citation17]. Besides salivary gland neutrophils, oral epithelial cells and desquamated oral epithelial cells may also contribute to salivary LL-37 content. Indeed, hCAP18/LL-37 has been convincingly demonstrated in desquamated oral epithelial cells using immunocytochemistry, Western blot and ELISA, arguing that these cells may act as a reservoir for hCAP18/LL-37 [Citation18]. However, the functional importance of endogenous desquamated oral epithelial cell hCAP18/LL-37 has not been clarified.
Here, we determine hCAP18/LL-37 levels in human desquamated epithelial cells isolated from whole saliva and assess the anti-bacterial effects of LL-37 on two oral colonizers: S. mutans and S. gordonii. We show that desquamated oral epithelial cells spontaneously release/leak LL-37. Furthermore, we demonstrate that homogenates of desquamated oral epithelial cells possess no anti-bacterial activity, suggesting that they contain concentrations of hCAP18/LL-37 which are too low to have an impact on bacterial viability. In contrast, exogenous, synthetic LL-37 reduces viability of S. mutans but not S. gordonii, showing that LL-37 acts differentially on these two types of Streptococci.
Materials and methods
Isolation of desquamated epithelial cells of human saliva
Desquamated epithelial cells were isolated from unstimulated whole saliva collected from four healthy volunteers, three female and one male donor (26–59 years of age) using the method previously described by Aidoukovitch et al. [Citation18]. All donors provided informed consent and the procedure was approved by the Regional Ethical Review Board, Lund and performed in accordance with the World Medical Association Declaration of Helsinki. Briefly, saliva was diluted in 0.1% dithiothreitol (DTT; Sigma-Aldrich, St Louis, MO, USA) in phosphate-buffered saline (PBS), incubated for 15 min on a bench roller at 4 °C, and filtered through a nylon 60 µm net (Merck Millipore, Burlington, MA, USA). The samples were then centrifuged at 82 g for 5 min at room temperature to isolate a cellular and a cell-free fraction of saliva. The cell pellet was rinsed once in PBS, centrifuged, and then re-suspended in either PBS or EpiGRO™ human epidermal keratinocyte medium (Millipore, Temecula, CA, USA). Cell counting was performed using Bürker chambers and cell morphology assessed by phase-contrast microscopy using an Olympus CKX41 microscope equipped with a digital camera (Olympus Europe, Hamburg, Germany). The collected saliva contained about 100,000 desquamated epithelial cells per ml. For both quantitative real-time RT-PCR and ELISA analysis, samples from each volunteer were analyzed separately.
To assess whether desquamated oral epithelial cells can bind/internalize exogenous LL-37, cells were seeded in 6-well plates and incubated with or without synthetic LL-37 in a water-jacket cell incubator at 37 °C under 5% CO2 in air for 4 h in EpiGRO™ human epidermal keratinocyte medium. At the end of the incubation, cell suspensions were collected and centrifuged at 82 g for 5 min in room temperature. After centrifugation, the supernatant was discarded and the pellet was washed with PBS, followed by counting and re-suspension in PBS. Cell-homogenates were prepared by sonication for 2 × 10 s on ice.
For assessment of cellular leakage of hCAP18/LL-37, cells were suspended in PBS in 12-well plates at 37 °C under 5% CO2 in air in a cell incubator for 20, 70 and 130 min. After incubation, cells were removed through gentle centrifugation (82 g for 5 min at room temperature) and supernatants collected.
Cell line and cell culture
Human skin keratinocyte cell line HaCaT cells (CLS Cell Line Service GmbH, Eppelheim, Germany) were cultured in DMEM/Ham’s F12 (1:1) medium (Thermo Fisher Scientific, Waltham, MA, USA) supplemented with antibiotics (penicillin 50 units/ml and streptomycin 50 µg/ml, both from Biochrom GmbH, Berlin, Germany) and 10% foetal bovine serum (Biochrom). The HaCaT cells were kept in a water-jacketed cell incubator at 37 °C under 5% CO2 in air. The cells were trypsinized and re-seeded upon reaching confluence. For the HaCaT cells, each culture well was regarded as one biological replicate (n = 1).
Quantitative real-time RT-PCR
The cells were collected and lysed in QIAzol (Qiagen, Venlo, The Netherlands). Extraction and purification of total RNA was carried out using a miRNeasy Mini Kit (Qiagen) and a QIAcube machine (Qiagen), according to the manufacturer’s instructions. RNA concentration and quality were determined using a Nano Drop 2000 C spectrophotometer (Thermo Fisher Scientific, Waltham, MA, USA). Transcript levels were analyzed by a one-step quantitative real-time RT-PCR using a thermal cycler (Applied Biosystems, Walthamn, MA, USA), QuantiFast SYBR Green RT-PCR kit (Qiagen) and QuantiTect primer assay (Qiagen). The delta CT method was used to calculate target gene expression, with glyceraldehyde 3-phosphate dehydrogenase (GAPDH) as reference gene. The CT values (Cycle Threshold, number of cycles required for the PCR product to span the limit of detection) were used to assess CAMP gene expression in desquamated oral epithelial cells and HaCaT cells. Each sample was analyzed in duplicate. Primers for LL-37 (HS_CAMP_1_SG) and GAPDH (HS_GAPDH_2_SG) were purchased from Qiagen.
Enzyme-linked immunosorbent assay (ELISA)
The concentration of hCAP18/LL-37 protein in cell homogenates and supernatants was determined using an ELISA assay (The HK321 LL-37 ELISA, Hycult Biotech, Uden, The Netherlands) according to the manufacturer’s instructions. Samples were incubated in wells coated with LL-37 antibody, and a biotinylated tracer antibody was then added to bind to the captured LL-37. A streptavidin-peroxidase conjugate which binds to the biotinylated tracer antibody and reacts with its substrate tetramethylbenzidine was then included. This reaction was stopped by oxalic acid. The absorbance at 450 nm was read using a Multiscan Go Microplate Spectophotometer (Thermo Fisher Scientific). Each sample was analyzed in duplicate or triplicate. The HK321 LL-37 ELISA that we use here cannot discriminate between human LL-37 and hCAP18.
Culture of S. mutans and S. gordonii and assessment of bacterial viability
Type strains of S. mutans (UA159) and S. gordonii DL1 (Challis) were grown to mid-exponential growth phase (OD600 = 0.4) in Todd-Hewitt broth at 37 °C, 5% CO2 in air. Aliquots were centrifuged (2000 g, 4 °C, 5 min) and the bacterial pellets incubated for 24 h in a cell incubator at 37 °C under 5% CO2 in air with an equal volume of homogenates of desquamated oral epithelial cells, synthetic LL-37 or vehicle control as appropriate. At the end of the incubation, aliquots were added into mini-flow cells (µ-slide VI Ibi-treat flow-cells (Ibidi GmbH, Martinsried, Germany) and centrifuged at 2000 g, 20 °C for 5 min prior to the assessment of bacterial viability by staining with the LIVE/DEAD® BacLight™ (Molecular Probes, Eugene, Oregon, USA) kit according to the manufacturer’s instructions. The slides were then viewed using a laser scanning confocal microscope with an excitation wavelength of 488 nm (Nikon Eclipse TE2000, Nikon Corp., Tokyo, Japan). Ten randomly selected images were taken for each sample and analysis of live (green) and dead (red) cells performed using the BioImage_L 2.1 software. All experiments were performed in duplicate or triplicate using independent biological replicates.
Agents
Synthetic LL-37 (Bachem, Bubendorf, Switzerland) was dissolved in DMSO (Sigma-Aldrich). Controls received vehicle as appropriate. The final concentration of DMSO was 0.1%.
Statistics
Summarized data are presented as mean ± standard error of the mean. Statistical significance was calculated using the Student’s two-tailed t-test for paired comparisons (control cells vs. cells treated with LL-37 for each individual) or one-way ANOVA for multiple comparisons with Bonferroni’s multiple comparison’s test for post-hoc analysis as appropriate. p-Values less than .05 were considered statistically significant. Each experiment was repeated at least two times. Data analysis was performed using the GraphPad Prism 8 (GraphPad Software, San Diego, CA, USA).
Results
Desquamated epithelial cells of human whole saliva express the CAMP gene and contain hCAP18/LL-37 protein
First, we assessed morphology of cells isolated from human whole saliva. The cells are large and flat, and they also show small nuclei and a large cytoplasmic fraction as demonstrated by phase-contrast microscopy (data not shown). These morphological characteristics are representative for aged epidermal keratinocytes and desquamated oral epithelial cells [Citation18,Citation19]. No or very few cells with different morphology compared to that of desquamated oral epithelial cells were detected. Next, we assessed CAMP gene activity in the desquamated oral epithelial cells using quantitative real-time RT-PCR. Human skin keratinocyte cell line HaCaT cells were included as positive control, since epithelial cells are well-known producers of hCAP18/LL-37 [Citation1,Citation2]. Desquamated oral epithelial cells isolated from each of the four volunteers included in the study expressed transcript for CAMP (CT value for CAMP was 32.3 ± 1.0, n = 4). For HaCaT cells, the corresponding value was 30.8 ± 0.8 (n = 5). In the next experiments, we determined hCAP18/LL-37 cellular protein concentration in isolated desquamated oral epithelial cells treated with or without synthetic LL-37 using ELISA. The endogenous concentration of hCAP18/LL-37 in 25,000 untreated control cells was around 10 ng (). Pre-treatment of the cells with 1 µM LL-37 for 4 h increased cellular hCAP18/LL-37 levels by around 30 times (). Cells spiked with exogenous LL-37 can represent desquamated oral epithelial cells loaded with LL-37 processed from hCAP18 released by glandular cells of the major salivary glands, similar to what may occur in whole saliva in-vivo [Citation16,Citation17]. We conclude that desquamated oral epithelial cells express hCAP18/LL-37 and, furthermore, we propose that these cells can bind and/or internalize exogenous LL-37.
Figure 1. Desquamated epithelial cells of human whole saliva contain hCAP18/LL-37 protein. Desquamated oral epithelial cells treated with exogenous LL-37 (1 µM) for 4 h (+) contain about 30 times more hCAP18/LL-37 than non-treated control cells (−). Desquamated oral epithelial cells were isolated from all four volunteers enrolled in the study, and hCAP18/LL-37 protein levels were analyzed in samples from each of the four individuals. Values are presented as mean ± standard error of the mean of four individuals in each group. ***represents p < .001.
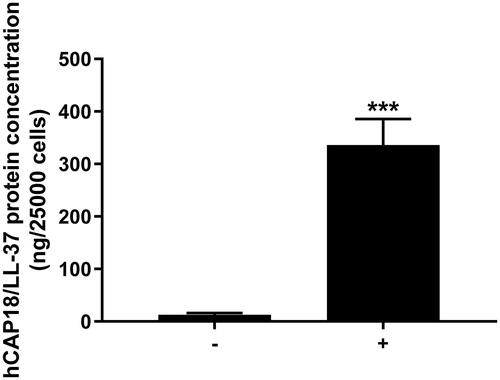
Desquamated oral epithelial cells spontaneously release/leak hCAP18/LL-37
Next, we investigated if hCAP18/LL-37 is spontaneously released from the desquamated oral epithelial cells. Cells were isolated from three of the four volunteers included in the study. ELISA analysis showed that the hCAP18/LL-37 peptide was spontaneously released within 20 min, but then hCAP18/LL-37 levels in the medium gradually decreased at 70 and 130 min, suggesting that the peptide is metabolized and processed extracellularly after its release (). Non-conditioned PBS contained no hCAP18/LL-37 as predicted ().
Figure 2. Desquamated oral epithelial cells spontaneously release/leak hCAP18/LL-37. Desquamated oral epithelial cells were isolated from three of the four volunteers included in the study and incubated in PBS for 20, 70 and 130 min, and at the end of incubation cells were removed and the supernatant collected for analysis. The levels of hCAP18/LL-37 in the medium were determined for each subject and time point. No hCAP18/LL-37 was observed in non-conditioned PBS, whereas PBS which had been in contact with cells for 20, 70 and 130 min contained the peptide. Data are presented as mean ± standard error of the mean of three individuals. * and ** represent p < .05 and p < .01, respectively, vs. data for the 20 min time point.
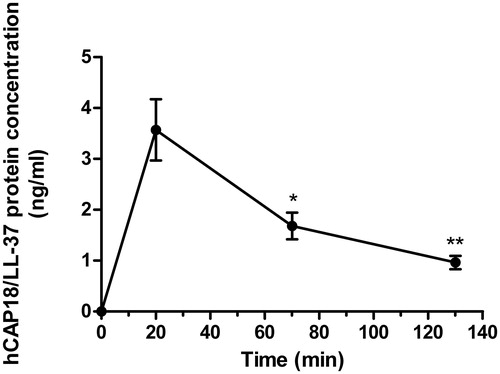
Exogenous, synthetic LL-37 but not homogenate of desquamated oral epithelial cells reduces S. mutans viability
In the next experiments, we assessed the activity of exogenous, synthetic LL-37 against S. mutans and S. gordonii using BacLight staining, and analysis of live (green) and dead (red) cells by laser scanning confocal microscopy. Treatment with 8 µM LL-37 for 24 h strongly reduced S. mutans viability, whereas it had no effect on S. gordonii (). Incubation with a lower concentration of LL-37 (1 µM) had no effect on the viability of either S. mutans or S. gordonii ().
Figure 3. Incubation with synthetic LL-37 for 24 h reduces S. mutans viability. S. mutans (A-C) or S. gordonii (D-F) were treated with 1 (B, E) or 8 (C, F) µM LL-37 and the proportion of live and dead bacteria determined. Controls (A, D) received vehicle as appropriate. Experiments were performed in duplicate, and images are representative for each group. Scale bar in panel F represents 25 µm (for all images).
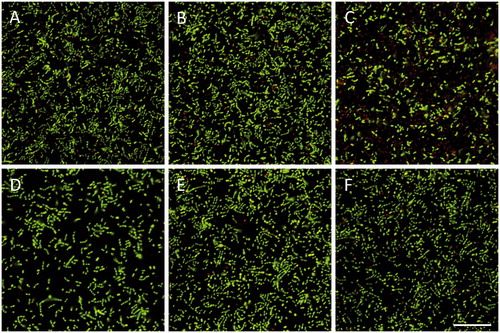
Next, we assessed the effects of homogenates of desquamated oral epithelial cells on S. mutans viability in order to investigate if the cells contain high enough endogenous LL-37 concentrations to influence bacterial viability. We only investigated the effects of cellular homogenate on S. mutans viability, since S. mutans, but not S. gordonii, is sensitive to synthetic LL-37. The bacteria (2 × 106 per ml) were incubated in cell homogenates prepared from 100,000 or 500,000 cells per ml PBS for 24 h, before the proportion of live (green) and dead (red) cells was assessed. In one experimental group, 500,000 cells were pre-incubated with 1 µM LL-37 for 4 h to spike the cells with the peptide. Synthetic LL-37 (8 µM) was included as positive control. The cell homogenates prepared from 100,000 or 500,000 cells had no effect on S. mutans viability, whereas synthetic LL-37 (8 µM) reduced viability by around 40% (). Hence, the endogenous cellular levels of LL-37 seem to be too low to have any impact on bacterial viability. For cellular homogenates of 100,000 and 500,000 cells, the concentration of LL-37 corresponds to 8 and 40 nM LL-37, respectively, based on the ELISA analysis (). For the spiked cells, the concentration of LL-37 is estimated to be 1.2 µM considering that these cells contain about 30 times higher levels of LL-37 compared to untreated control cells (). However, homogenates of the spiked cells also had no effect on S. mutans viability, suggesting that 1.2 µM LL-37 is too low a concentration of the peptide to be able to neutralize these bacteria. Notably, the ELISA cannot discriminate between hCAP18 and LL-37, and thus we may predict that the concentrations of LL-37 in the cellular homogenates can be even lower than the calculated ones presented above.
Figure 4. Incubation with synthetic LL-37 but not homogenates of desquamated oral epithelial cells for 24 h attenuates S. mutans viability. Neither cellular homogenates prepared from 100,000 cells per ml PBS (B) nor homogenates from 500,000 cells per ml PBS (C) had any impact on number of live and dead bacteria compared to control (A). Summarized data are shown in panel F. Also, incubation with homogenate of cells (500,000 cells per ml PBS) pre-treated with 1 µM LL-37 for 4 h to spike them with LL-37 had no effect on S. mutans viability (D, F), whereas synthetic LL-37 (8 µM), included as positive control, reduced the number of live bacteria by about 40% (E, F). Cells were isolated from one of the volunteers included in the study, and experiments were performed as independent experiments in duplicate. All experiments were performed with cells isolated from the same individual. For each sample, 10 images were analyzed, and the number of live bacteria presented as percent of total number. Values are presented as mean ± standard error of the mean of 9–10 observations in each group. *** represents p < .001 vs. control. Scale bar in panel E represents 25 µm (for all images).
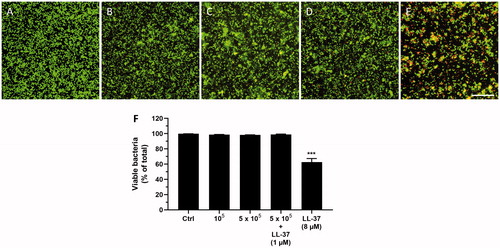
Concentration-response relationship for synthetic LL-37-induced attenuation of S. mutans viability
In order to establish the LL-37 concentration-response relationship for its activity against S. mutans, we treated bacteria with four different concentrations of synthetic LL-37 (0.1, 1, 8 and 10 µM) for 24 h. Treatment with 8 and 10 µM LL-37 reduced S. mutans viability by about 30%, whereas lower concentrations of the peptide (0.1 and 1 µM) had no effect (). Notably, the concentration-response relationship for LL-37-induced attenuation of S. mutans viability showed a prominent reduction of bacterial viability in the rather narrow concentration range of 1-8 µM.
Figure 5. Treatment with 8 and 10 µM of synthetic LL-37 reduces S. mutans viability. Bacteria were treated with or without LL-37 (0.1, 1, 8 and 10 µM) for 24 h and the proportion of live and dead bacteria assessed. Experiments were performed in triplicate. Values are presented as mean ± standard error of the mean of 29–30 observations in each group. *** represents p < .001 vs. control.
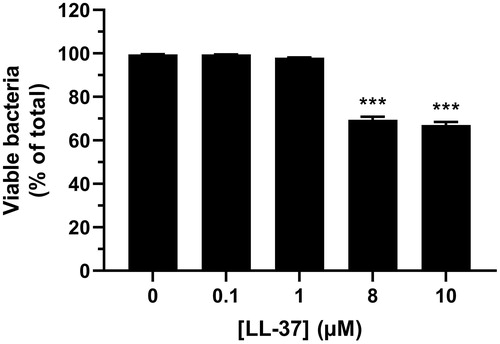
Discussion
In the present study, we demonstrate that desquamated epithelial cells of human whole saliva contain antimicrobial hCAP18/LL-37, but their endogenous cellular levels of the peptide are too low to have an impact on S. mutans viability based on ELISA analysis of cellular contents of hCAP18/LL-37 and the concentration-response relationship for synthetic LL-37-induced S. mutans cytotoxicity. High concentrations of LL-37 (>1 µM) have been observed in gingival crevicular fluid of patients with periodontitis and in psoriatic skin lesions of patients with psoriasis [Citation20,Citation21]. These reports indicate that very high concentrations of LL-37 can occur locally in association with inflammatory conditions. Here, we show that synthetic LL-37 neutralizes S. mutans in the 8-10 µM concentration range, i.e. at a similar concentration of the peptide to that observed in the gingival crevicular fluid of diseased sites in periodontitis patients [Citation20]. Importantly, salivary concentration of LL-37 in humans has been reported to be in the range 0.15-6.1 μM, and thus the LL-37-evoked neutralization of S. mutans observed in the present study indeed seems to be relevant for the in-vivo situation [Citation22].
Interestingly, we show that synthetic LL-37 affects the viability of S. mutans, but the peptide seems to lack effect against S. gordonii, suggesting that LL-37-induced anti-bacterial activity depends on differeing bacterial properties amongst streptococcal species. We assessed viability of S. mutans and S. gordonii treated with a high concentration of LL-37 (8 μM) in parallel experiments observing a prominent reduction of S. mutans viability but no effect on S. gordonii. Of course, we cannot rule out that higher concentration of LL-37 (>8 µM) show cytotoxic effects also against S. gordonii, but importantly these concentrations of LL-37 are presumably too high to be relevant for the in-vivo situation. The differential sensitivity of S. mutans and S. gordonii for LL-37-induced cytotoxicity may reflect structural and or functional differences of these two types of Streptococci. The antimicrobial activity of the positively charged LL-37 molecule is thought to depend on high-affinity binding of the peptide to the negatively charged bacterial cell wall [Citation8]. Hence, the cell wall of S. mutans may carry more negatively charged sub-units than S. gordonii. However, the differential sensitivity of S. mutans and S. gordonii to LL-37 may also reflect differences in their physiological/pathophysiological properties. S. mutans has been associated with dental caries, and thus our present results are probably of pathophysiological relevance for initiation and/or development of caries [Citation23]. LL-37-induced neutralization of S. mutans has been shown before, and interestingly S. mutans from caries-active individuals seemed to be less sensitive to LL-37-induced cytotoxicity than S. mutans strains from caries-free subjects, indicating that S. mutans from caries-active patients may develop resistance to the peptide [Citation24]. Besides its antibacterial activity, LL-37 also shows cytotoxic effects in human host cells. The peptide has been shown to reduce human host cell viability in a rapid process involving plasma-membrane permeabilization [Citation25]. Therefore, we assess in the present study direct effects of LL-37 on bacterial cell viability using LIVE/DEAD® BacLight™ kit rather than CFU analysis.
Here, we show that desquamated oral epithelial cells spontaneously release/leak hCAP18/LL-37 to their extracellular environment, suggesting that these cells may contribute to salivary levels of free hCAP18/LL-37 through this mechanism. Our data further demonstrate that hCAP18/LL-37 is rapidly released from the desquamated oral epithelial cells with a peak in extracellular concentration at 20 min, and that the concentration of hCAP18/LL-37 in the medium then decreases at 70 and 130 min. A possible explanation to the reduction of the peptide at 70 and 130 min is that hCAP18/LL-37 released by the desquamated oral epithelial cells may bind to negatively charged moieties of the plasma-membrane and/or to negatively charged macromolecules such as DNA, and that this cell-bound pool of hCAP18/LL-37 is spun down by centrifugation and therefore not detected in the supernatant. Immunoreactivity for hCAP18/LL-37 is mainly observed in the periphery of desquamated oral epithelial cells, and this may represent a readily releasable pool of hCAP18/LL-37 which is released when the cells go into lysis [Citation18]. Taken together, our findings suggest that spontaneous release/leakage of hCAP18/LL-37 from desquamated oral epithelial cells may contribute to the amounts of biologically active LL-37 in whole saliva.
In summary, we show that desquamated oral epithelial cells contain hCAP18/LL-37, and that these cells spontaneously release/leak the peptide to their extracellular environment. Moreover, we demonstrate that exogenous, synthetic LL-37 shows activity against S. mutans but not S. gordonii, and that the endogenous levels of LL-37 in desquamated oral epithelial cells are too low to have an impact on S. mutans viability.
Acknowledgements
The authors thank Katarzyna Kawka for excellent technical assistance.
Disclosure statement
The authors report no conflicts of interest.
Additional information
Funding
References
- Zanetti M. The role of cathelicidins in the innate host defenses of mammals. Curr Issues Mol Biol. 2005;7(2):179–196.
- Hancock REW, Haney EF, Gill EE. The immunology of host defence peptides: beyond antimicrobial activity. Nat Rev Immunol. 2016;16(5):321–334.
- Sørensen OE, Follin P, Johnsen AH, et al. Human cathelicidin, hCAP-18, is processed to the antimicrobial peptide LL-37 by extracellular cleavage with proteinase 3. Blood. 2001;97(12):3951–3959.
- Yamasaki K, Schauber J, Coda A, et al. Kallikrein-mediated proteolysis regulates the antimicrobial effects of cathelicidins in skin. Faseb J. 2006;20(12):2068–2080.
- Larrick JW, Hirata M, Balint RF, et al. Human CAP18: a novel antimicrobial lipopolysaccharide-binding protein. Infect Immun. 1995;63(4):1291–1297.
- Turner J, Cho Y, Dinh NN, et al. Activities of LL-37, a cathelicidin-associated antimicrobial peptide of human neutrophils. Antimicrob Agents Chemother. 1998;42(9):2206–2214.
- Nizet V, Ohtake T, Lauth X, et al. Innate antimicrobial peptide protects the skin from invasive bacterial infection. Nature. 2001;414(6862):454–457.
- Burton MF, Steel PG. The chemistry and biology of LL-37. Nat Prod Rep. 2009;26(12):1572–1584.
- Cederlund A, Gudmundsson GH, Agerberth B. Antimicrobial peptides important in innate immunity. Febs J. 2011;278(20):3942–3951.
- Ji S, Hyun J, Park E, et al. Susceptibility of various oral bacteria to antimicrobial peptides and to phagocytosis by neutrophils. J Periodontal Res. 2007;42(5):410–419.
- Bechinger B, Gorr SU. Antimicrobial peptides: mechanisms of action and resistance. J Dent Res. 2017;96(3):254–260.
- Murakami M, Ohtake T, Dorschner RA, et al. Cathelicidin antimicrobial peptides are expressed in salivary glands and saliva. J Dent Res. 2002;81(12):845–850.
- Tao R, Jurevic RJ, Coulton KK, et al. Salivary antimicrobial peptide expression and dental caries experience in children. Antimicrob Agents Chemother. 2005;49(9):3883–3888.
- Davidopoulou S, Diza E, Sakellari D, et al. Salivary concentration of free LL-37 in edentulism, chronic periodontitis and healthy periodontium. Arch Oral Biol. 2013;58(8):930–934.
- Colombo NH, Ribas LF, Pereira JA, et al. Antimicrobial peptides in saliva of children with severe early childhood caries. Arch Oral Biol. 2016;69:40–46.
- Svensson D, Aidoukovitch A, Anders E, et al. The host defense peptide LL-37 is detected in human parotid and submandibular/sublingual saliva and expressed in glandular neutrophils. Eur J Oral Sci. 2018;126(2):93–100.
- Yang TY, Zhou WJ, Du Y, et al. Role of saliva proteinase 3 in dental caries. Int J Oral Sci. 2015;7(3):174–178.
- Aidoukovitch A, Dahl S, Fält F, et al. Antimicrobial peptide LL-37 and its pro-form, hCAP18, in desquamated epithelial cells of human whole saliva. Eur J Oral Sci. 2020;128(1):1–6.
- Soroka Y, Ma'or Z, Leshem Y, et al. Aged keratinocyte phenotyping: morphology, biochemical markers and effects of dead sea minerals. Exp Gerontol. 2008;43(10):947–957.
- Turkoglu O, Emingil G, Eren G, et al. Gingival crevicular fluid and serum hCAP18/LL-37 levels in generalized aggressive periodontitis. Clin Oral Invest. 2017;21(3):763–769.
- Ong PY, Ohtake T, Brandt C, et al. Endogenous antimicrobial peptides and skin infections in atopic dermatitis. N Engl J Med. 2002;347(15):1151–1160.
- Byfield FJ, Wen Q, Leszczynska K, et al. Cathelicidin LL-37 peptide regulates endothelial cell stiffness and endothelial barrier permeability. Am J Physiol Cell Physiol. 2011;300(1):C105–C112.
- Tanzer JM, Livingston J, Thompson AM. The microbiology of primary dental caries in humans. J Dent Educ. 2001;65(10):1028–1037.
- Phattarataratip E, Olson B, Broffitt B, et al. Streptococcus mutans strains recovered from caries-active or caries-free individuals differ in sensitivity to host antimicrobial peptides. Mol Oral Microbiol. 2011;26(3):187–199.
- Svensson D, Wilk L, Mörgelin M, et al. LL-37-induced host cell cytotoxicity depends on cellular expression of the globular C1q receptor (p33). Biochem J. 2016;473(1):87–98.