Abstract
Background
Allergic rhinitis (AR), a common condition in the westernized world, is suggested to be more immunologically complex than the archetypical ‘Th2’ inflammation. New approaches are needed to decode this complexity.
Aims/objectives
In this study, we explored a novel histology-based analysis for circulating blood leukocyte profiling in 16 patients with seasonal AR outside and during the pollen season.
Material and Methods
Leukocytes were purified with minimal ex-vivo artefacts, embedded into agarose-paraffin pellets for immunohistochemistry-based immune cell profiling. Blood leukocyte mapping was performed.
Results
Samples collected during the pollen season had statistically increased eosinophils, neutrophils, monocytes, and CD8+ T-lymphocytes compared to the off-season baseline. In contrast, no change was observed for CD20+ B-lymphocytes and CD3+ T-lymphocytes. Subclassification of CD4+ T-helper cells demonstrated a parallel and significant expansion of Th2 and Th17-cells during the pollen season, while Th1-cells remained unchanged. Whereas absolute basophils numbers were unaltered, the basophil markers GATA2 and CPA3 increased during the pollen season.
Conclusions and Significance
This study introduces a novel and applicable method for systemic immune cell screening and provides further evidence of complex and parallel Th2 and Th17-immune signatures in seasonal AR. It also forwards GATA2 and CPA3 as potential biomarkers for ongoing allergic inflammation.
Chinese Abstract
背景:过敏性鼻炎 (AR) 是西方化世界的一种常见病症, 被认为比典型的“Th2”炎症在免疫学上更为复杂, 需要新的方法来解释这种复杂性。
目的:在本项研究中, 我们探索了一种基于组织学的新型分析法, 用于分析 16 名在花粉季节之外和期间患有季节性 AR 的患者的循环血液白细胞特征。
材料和方法:用尽量少的离体人工制品纯化白细胞, 将其包埋于琼脂糖石蜡颗粒中, 用于基于免疫组织化学的免疫细胞分析, 并进行了血液白细胞制图。
结果:与淡季基线相比, 在花粉季节收集的样本具有统计学意义上的嗜酸性粒细胞、嗜中性粒细胞、单核细胞和 CD8+ 淋巴细胞的增加。 相反, CD20+ B 淋巴细胞和 CD3+ T 淋巴细胞没有观察到任何变化。 CD4+ T 辅助细胞的亚分类表明, 在花粉季节, Th2 和 Th17 细胞显示平行且显著的扩增, 而 Th1 细胞保持不变。 在花粉季节, 尽管嗜碱性粒细胞的绝对数量没有改变, 但嗜碱性粒细胞标记物 GATA2 和 CPA3有所增加。
结论和意义:本研究介绍了一种新的适用于系统免疫细胞筛选的方法, 并提供了季节性 AR 的复杂且平行的 Th2 和 Th17 免疫特征的进一步证据。 它还提出将 GATA2 和 CPA3 作为持续过敏性炎症的潜在生物标志物。
Introduction
Allergic rhinitis (AR) is largely considered to be an IgE-mediated disease with Th2-driven eosinophil-rich mucosal inflammation as main immunopathological feature [Citation1]. Its treatment comprises oral/topical antihistamines and nasal corticosteroids [Citation2]. Additional options are allergen-immunotherapy and, more recently, biologics targeting IgE [Citation3] or type-2 cytokines and their receptors [Citation4]. The use of costly biologics is predominantly reserved for AR-patients with concomitant type-2 and eosinophilic asthma and/or nasal polyposis. However, with long-term safety data and expected future price reductions they may become options for a larger group of patients. Indeed, improved measures are needed, because with current pharmacotherapy up to 20% of AR-patients remain highly symptomatic [Citation5].
An important factor to consider when optimizing the treatment for AR is introduction of improved biomarkers. In particular, in the emerging era of personalized medicine, such are needed to inform about the immunological/inflammatory nature underlying the symptoms of individual patients. Indeed, recent data has forwarded AR to be a more immunologically complex disease than previously thought [Citation2,Citation6]. Thus, although the archetype allergic Th2 and IgE-driven eosinophilic inflammation do play an important role [Citation1], other separate and/or parallel immune responses may also contribute to the immunopathology. For example, it has recently been observed that neutrophil responses may be a significant feature of AR [Citation7] and that Th17-immune responses may contribute [Citation8].
A range of biomarkers have been tested to monitor disease activity and inform about inflammatory status in AR [Citation6,Citation9]. Analyses of plasma proteins, reflecting plasma exudation, and individual cytokines in nasal lavages have been utilized [Citation10], but their use may be limited in clinical practice. Assessment of routine blood samples has a potential to overcome this, and has, despite only being a systemic measure, been used to monitor immune markers and circulating leukocytes in AR [Citation11,Citation12]. The assessment of peripheral blood leukocytes in AR has traditionally been performed by flow cytometry and fluorescence activated cell sorting (FACS) [Citation12]. While this is a powerful and valuable method, tentative limitations include artefactual cell changes during cell purification and that cell markers to be analysed must be determined beforehand.
The present study utilizes a novel histology-based analysis of blood leukocytes for profiling circulating immune cells present in routine blood samples. Specifically, we examine enriched blood leukocytes, which had been subjected to minimal ex vivo handling before being fixed and embedded in paraffin, for histological analysis. Comparisons are made between multiple leukocyte population markers, including neutrophils and Th17-lymphocytes, in samples collected during and after the natural pollen season in patients with seasonal AR.
Methods
Patients and clinical sampling
Sixteen patients with seasonal AR participated (mean age 26, range 20-39 years). Inclusion criteria were a history of seasonal AR for at least two years and a positive skin prick (Soluprick, ALK, Hørsholm, Denmark) test to birch pollen or other seasonal allergen (15 of the patients were allergic to birch pollen) (). Serum-specific IgE was not included in the protocol. Exclusion criteria was chronic nasal disease (i.e. chronic rhinosinusitis or perennial AR), moderate-to-severe asthma, and recent respiratory tract infections. Individuals with a positive skin prick test to house dust mite or animal dander were allowed to participate if they did not present any perennial symptoms and if they were not exposed to allergen-relevant animals. The study was approved by the Swedish Ethical Review Board (2016/166) and informed consent was obtained. Leukocyte profiles were explored in peripheral blood samples collected during the Swedish birch pollen season in the spring and off-season late in the autumn.
Table 1. Patient-characteristics.
Assessment of symptoms
Symptoms were recorded during the pollen season using diary cards [Citation13]. The recorded symptoms, i.e. rhinorrhoea, nasal blockage, and sneezing, were scored on a four-graded scale: grade 0, none; grade 1, mild; grade 2, moderate; and grade 3, severe symptoms. For each day, a total nasal symptom score (TNSS) was calculated.
Preparation of blood leukocyte pellets
To make blood leukocyte pellets we slightly modified a protocol that previously have been shown to result in minimal ex vivo handling artifacts or handling-evoked accidental cell activation [Citation14] (). Briefly, within minutes after the collection, 8 ml blood samples were immediately transferred into heparin tubes containing 16 ml of 4% buffered formalin (Histolab-REF:02176, pH7.4) and left for 1-hour at room temperature. To sediment red blood cells (RBC) from formalin-fixed whole blood, the blood sample was divided into two equal volumes of 12 ml and each portion was transferred into tubes containing 5 ml of dextran (5% dextran + 3 g glucose in PBS) and left for 1-hour at room temperature.
Figure 1. Schematic overview of blood leukocyte pellet preparation (A). After a brief pre-fixation, cells are separated by dextran sedimentation (1). Layers containing leukocytes are collected and white blood cells are spun down into pre-warmed melted agarose (2), which after being allowed to solidify and subjected to 4% formaldehyde fixation is embedded in paraffin (3) and cut into sections used for detection of multiple leukocyte markers by immunohistochemistry (4) (scale bar = 35 µm). Selection of optimal low pH heat-induced antigen retrieval conditions (B) and representative example of Htx-stained leukocytes amidst the few remaining erythrocytes (C) (scale bar = 10 µm). Example of detection and segmentation strategies for computerized quantitative image analysis (D,E) (D, scale bar = 25 µm; E, scale bar = 17 µm). In panel E, the brown RORγt-positive nuclei up to the left is touching CD4-immunoreactivity and is thus reclassified as a Th17 cell.
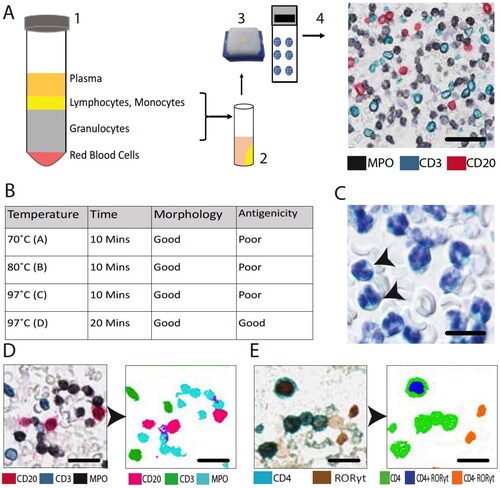
After 1-hour dextran-based RBC sedimentation, the leukocyte enriched plasma was carefully retrieved by a pipette and centrifuged at 1400 rpm (at 27 °C) for 10 min. The obtained leukocyte pellet was resuspended into 4% formalin and transferred into a tube containing pre-warmed melted agarose (low melting agarose, Sigma-Aldrich A4018-10G) and again centrifuged at 1400 rpm for 10 min (at 27 °C). The hardened agarose containing the leukocyte pellet was left in 4% formalin for approximately 12 h (overnight) at room temperature before being subjected to standard dehydration (SAKURA-Tissue-Tek VIP® 6 AI automated dehydration machine) and embedding into paraffin pellets.
Immunohistochemistry
Paraffin-embedded blood pellets were sectioned at 4 µm thickness, and at least five blood pellet sections were placed per slide to create blood pellet micro array slides. As part of the antigen retrieval process, slides were baked at 60 °C for 45 min and pretreated with pH-low (pH 6) in an antigen retrieval solution (Dako-DM829) in a Dako PT Link machine (97 °C for 20 min; PT-200, Dako/Agilent, Glostrup, Denmark).
A modified double and triple staining immunohistochemistry (IHC) protocol was applied for simultaneous visualization of multiple immune cells [Citation15]. Briefly, after antigen retrieval, endogenous peroxidase was blocked with peroxidase blocking reagent (Dako-DM821) for 10 min. Next, the slides were incubated with the primary antibody for 1 h, washed extensively, and thereafter incubated with an isotype-relevant secondary antibody coupled to an HRP-labeled polymer (Envision Flex-DM822 amplification kit) for 30 min before being subjected to a 10-minute incubation with HRP chromogen substrate. Excess substrate unbound antibodies in the sections were denatured using 1:3 diluted denaturing solution (Biocare Medical-DNS 001 L) for 5 min. After the denaturation step, a similar protocol round was repeated to achieve the double and triple staining. For double and triple staining, the following HRP chromogens were used; 3,3′-Diaminobenzidine (DAB-Dako-DM827, brown precipitate), vina green (Biocare-BRR807BC), and deep space black (Biocare-BRI4015 H, L). Finally, the slides were stained by hematoxylin (Htx) nucleus counterstaining and mounted in pertex (Pertex-Histolab). Information on the primary cell marker antibodies is presented in . Control experiments where the primary antibody was replaced with a non-relevant isotype control did not result in any staining. Importantly, for the comparison between off-season and season marker values, all sample preparations were identical and offseason and seasonal samples were IHC stained and analyzed simultaneously.
Table 2. Immune cell markers and primary antibodies.
Digitalization of immunostained leukocyte pellets and computerized image analysis
An automated Olympus VS-120 Virtual Slide Microscope was used for high resolution digitalization (20X lens magnification) of the entire section area from all multi-stained blood pellet sections. In each cell pellet, the immunoreactivity for each stained cell marker (i.e. the detection chromogens), as well as the background Htx-stained cell nuclei, were automatically detected by color and spectral-based segmentation by computerized image analysis (Visiomorph software, Visopharm, Denmark) (). Finally, for each cell marker, the total area (pixels) of the corresponding chromogen was calculated.
The areas of nuclei and immunostainings were combined to obtain the total area of blood pellet. Finally, the area of each marker was divided with the total cell pellet area and converted into a percentage of immunoreactivity. In order to automatically detect double stained Th1, Th2, and Th17 CD4+-lymphocytes, each nucleus with positive staining for the relevant nuclear transcription factor (T-bet, GATA3, and RORγt, respectively) were detected by color segmentation. Next, any transcription factor-positive nucleus that was in direct spatial contact with surrounding CD4-immunoreactivity was automatically reclassified as the corresponding Th-subset ().
Statistics
All data were presented as individual or mean values. The Wilcoxon signed-rank test was used to compare data from during and after the pollen season (paired data). The Spearman’s correlation coefficient analysis was used to investigate statistical relationships between parameters. The tests are non-parametric alternatives recommended when the data cannot be assumed to be normally distributed. All calculation were performed in Prism 9.
Results
Method optimization and validation
Initial tests revealed that the current protocol of generated paraffin blood leukocyte pellets for histological analysis resulted in a well-preserved morphology of the cells as inspected after routine Htx-staining. Heat-induced antigen retrieval (HIER) prior to IHC had a slightly negative effect on the morphology. Thus, initial studies, with different combinations of programmed time and temperature curves were tested in order to yield the combination that was best suited morphology preservation, robust IHC staining and computerized image analysis (). Our preliminary explorations also revealed that each cross sectioned pellet typically contained cross section planes of 5000-7000 individual leukocytes.
Using the optimized protocol with 97 °C HIER for 20 min, freshly cut sections from all season and off-season blood samples were simultaneously subjected to immunohistochemical staining and computerized image analysis. Importantly, despite that our type of quantification is conceptually different from conventional routine differential cell counts, the rough overall proportions of cell markers representing the major cell types was fairly similar to the expected overall leukocyte composition (). Furthermore, routine clinical eosinophil differential counts correlated with the histology-based enumeration of immunoreactivity for the eosinophil cationic protein marker EG2 ().
Figure 2. Correlation between clinical routine eosinophil cell count and computerized quantification of eosinophil granule marker EG2 in blood leukocyte pellets (A). The overall composition and relative proportion of immunoreactivity for common leukocyte identity markers (B). Quantitative data of pairwise measurements of immunoreactive area in blood leukocyte pellets collected off-season/autumn and during the spring pollen season (C–I). Data shown are for major leukocyte population markers. * and *** denote a significance level of p < .05 and p < .001, respectively. Correlation in A is analyzed by Spearman’s correlation coefficient analysis.
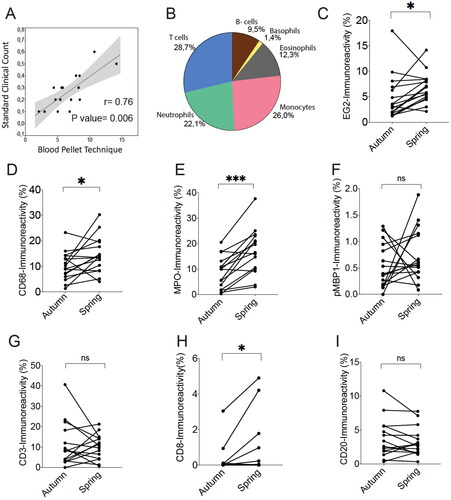
Altered blood cell marker immunoreactivity during the pollen season
We performed a paired analysis comparing off-season (autumn) and pollen season (spring) immunoreactivity values for major immune cell populations. Our data revealed statistically elevated levels of EG2 (eosinophils), CD68 (monocytes), and MPO (myeloperoxidase; neutrophils) during the pollen season compared to paired off-season autumn values (). No statistical change was observed for the basophil marker pro major basic protein 1 (pMBP1), the pan T-cell marker CD3, or the B-lymphocyte marker CD20 ().
Increased Th2 and Th17 blood signatures
Assessment of T-lymphocyte subpopulations, including transcription factor-defined CD4+ T-helper subsets, demonstrated a statistically elevated value for CD8 immunoreactivity (i.e. cytotoxic T-cells) compared to off-season values. Among the Th-cell types CD4+, GATA3+ double positive Th2 cells, and CD4+ RORγt+ Th17-cells increased during the pollen season, whereas no such change was observed for CD4+ T-bet+ Th1-cells ().
Figure 3. Quantitative T-helper subset data of pairwise autumn and spring measurements for each individual patient (A, C, F). Th1, Th2, and Th17-populations were identified by double staining for CD4 and their corresponding transcription factor; i.e. T-bet, GATA3, and RORγt (B, D, E, G) (scale bar = 5 µm). * and ** denote a significance level of p < .05 and p < .01, respectively.
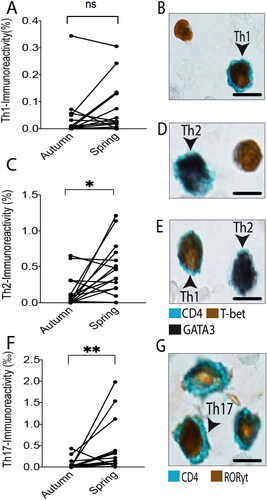
Basophil-related markers
Basophils are linked with allergic disorders. Interestingly, although no statistical seasonal increase was observed for the basophil markers pMBP1 (), a robust statistical increase was observed for the basophil transcription factor GATA2 (). A subset of the basophils has been described to also contain the granule proteases carboxypeptidase 3 (CPA3) and tryptase [Citation16,Citation17]. During the pollen season elevated blood leukocyte CPA3 but not tryptase could be detected together with a non-statistical trend towards increased surface bound IgE ().
Figure 4. Pairwise leukocyte pellet data for the basophil-associated markers IgE, GATA2, CPA3, and tryptase (A, C-E). ** and *** denote a significance level of p < .01 and p < .001, respectively. Representative example images of IgE-stained blood leukocytes (B) (scale bar = 50 µm (left) and 6 µm (right)). Triple immunofluorescence staining exemplifying a basophil, detected with the basophil marker basogranulin, which is double positive for the granule proteases CPA3 and tryptase (F) (scale bar = 10 µm).
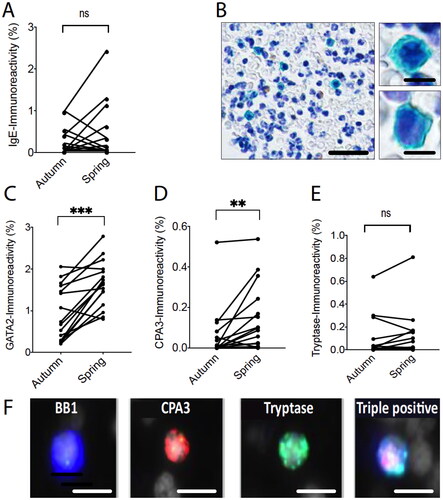
Cell marker correlations
During the pollen season many cell markers was expressed independently within individual patients. However, a statistical correlation was present between EG2/eosinophils and the basophil marker GATA2 (). Intriguingly, a strong correlation was also found also between Th2 and Th17-lymphocytes (). Although our study only included a limited number of patients it allowed for some insight into the differences between autumn and spring values among the various IHC markers. Some markers, such as blood leukocyte tryptase (i.e. basophil tryptase), pan CD3+ T cells, and the B-cell marker CD20, were of similar levels within patients examined during and after the pollen season. Despite that many of the cell markers were statistically increased during the spring pollen season, there was no clear correlations between leukocyte markers and the spring symptom scores.
Figure 5. Scattergrams demonstrating a correlation between blood leukocyte pellet levels of eosinophils (EG2 immunoreactivity) and the basophil-associated transcription factor GATA2 (A). Correlation between blood pellet Th2 and Th17-cells during the pollen season (B). The level of statistical correlation was tested with Spearman’s correlation coefficient analysis.
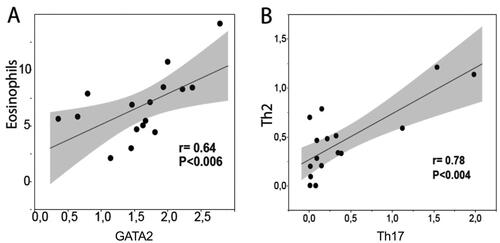
Discussion
The heterogeneous nature of inflammation in AR, and the emergence of new selective treatment options, call for improved ways to explore the immunological heterogeneity without invasive nasal sampling. This study presents such an approach and demonstrates that histological analysis of blood leukocyte pellets can be used for mapping circulating immune cell profiles. The fact that many immune cell parameters were increased during the pollen season show the capacity of the present approach to monitor clinically relevant changes in inflammation status. Of particular interest is our finding of increased parallel Th2, Th17, neutrophil, and eosinophil responses during the pollen season. Our data also reveal basophil-associated GATA2 and CPA3 as potential markers of ongoing allergic inflammation. The present approach may not be restricted to AR, but has bearings on most inflammatory conditions.
From a methodological perspective, it is important to stress that the present approach to monitor blood immune cell-markers is conceptually different from standard assessments of the leukocyte composition in blood, e.g. by flow cytometry and FACS, by differential cell counts of smears or cytospin preparations. Whereas FACS and blood differential cell counts measure numbers of whole cells, our analysis detects a normalized area of actual staining (i.e. immunoreactivity). In contrast to FACS and cytospin samples, the present paraffin-embedded pellets can be used to create large numbers of blood leukocyte sections (>100) for analysis. Furthermore, repeated sectioning and complementary analyses can be performed a long time after sampling (>10 years), which is a methodological advantage. Moreover, advanced computerized image analysis may be used to generate ‘FACS-like’ data of staining intensity for the thousands individual cells present in a leukocyte pellet section. Naturally, a stained marker area per marker-positive cell may differ, e.g. the nuclear transcription factors, cell membrane, and cytoplasmic granule markers analyzed in this study differ with respect to how much area of an entire cell that is stained. Together with differences in staining intensity, it should be expected that relative proportions between the present cell identification markers do not correspond to exact proportions of cell numbers. Despite this, a surprisingly good correlation was achieved in thus study between, e.g. eosinophil numbers determined by clinical routine and the present EG2-immunoreactivity (). Further methodological validation of the present histology-based approach using larger sample numbers is warranted.
The present study demonstrates that our methodological approach has the sensitivity to detect multiple allergen-evoked changes among circulating blood leukocytes. Among these, the significant eosinophil and Th2-responses were expected. Indeed, eosinophilia, which is a hallmark of AR, is thought to be driven by an underlying ‘Th2-response’ (more correctly labelled type 2 response, as type 2 cytokines may arise from cells other than CD4+ Th2-lymphocytes). Of immunopathological relevance, allergen-induced immune responses not only stimulate eosinophil infiltration into the nasal mucosa, but also their activation and cytotoxic mediator release through piecemeal degranulation and programmed cytolysis [Citation13]. Perhaps seemingly more surprising was our observation of very robust and parallel increases in Th17-cells and MPO immunoreactivity, suggesting a significant IL-17 driven neutrophil response. This is supported by the fact that IL17-cytokines like IL17A and IL17F stimulate immune cells and epithelial cells to release the neutrophil chemokines G-CSF and IL-8/CXCL8 [Citation18]. The observation of combined eosinophilic and coexisting neutrophilic inflammation during the pollen season under real life settings reinforces/supports the modern view that AR is more complex than the archetypical pure eosinophilic type 2 inflammation. Our findings thus agree with studies that suggest ‘mixed response’ as a significant feature of AR [Citation7,Citation11]. ‘Mixed type inflammation’ is described in other type 2 diseases, e.g. asthma and chronic rhinosinusitis with nasal polyps, and linked to more severe and therapy-resistant/refractory disease [Citation19,Citation20]. It is likely that the mechanisms behind co-emergence of eosinophilia and neutrophilia are multifactorial. Interestingly, among many alternatives, a recent report suggests that neutrophils in AR may not only be down-stream effector cells but may also contribute to the eosinophilia by priming T-cells to recruit eosinophils [Citation7]. Further research is needed to identify initial upstream molecular and environmental determinants behind the heterogeneous inflammation in seasonal AR.
Basophils are implicated in allergic diseases [Citation16] and basophil-associated biomarkers have an important role, e.g. to predict response to immunotherapy [Citation21]. Basophil activation status in the blood is commonly analyzed by FACS as a translocation of CD63, CD69, and CD203c to the cell surface [Citation22]. Unfortunately, the present type of histological analysis cannot reliably differentiate between cytosol and membrane immunoreactivity. Hence, we explored five alternative basophil-associated markers: pMBP1, GATA2, CPA3, tryptase, and surface bound IgE. While several of these may be expressed on other cells in inflamed tissues, they are more of less unique to basophils in circulating blood [Citation16]. For example, most mast cells express GATA2, tryptase, and surface bound IgE, and a significant proportion also expresses CPA3. However, mature mast cells are not present in the blood and mast cell progenitors are so exceedingly rare that they cannot have contributed to our overall blood analysis. The marker pMBP1 has been forwarded as a basophil pan marker but may also be present in eosinophil progenitors. Eosinophil progenitors, which also express GATA2, may increase during the pollen season and could therefore be a confounding factor in our assessment of GATA2 basophils. However, when staining for CD34+ progenitor cells these were present in exceedingly low numbers (data not shown). Hence, neither eosinophils nor mast cell progenitors could have contributed to our basophil marker outcome. Among the employed markers, CPA3 and GATA2 were significantly higher in-season than during off-season conditions. Although the biological background to this remain unknown, these markers may therefore be added to the list of potential blood biomarkers for assessing allergic inflammation.
In the present study, with the research aim to detect differences between patients presenting AR symptoms during a pollen season (cf. after the pollen season), TNSS was used. An alternative would have been symptom-recording via visual analogue scale, which was not chosen here. We acknowledge that the number of the patients in this study is relatively small for biomarker validation. However, the fact that the design allows for paired analyses, and that we did observe clear season – outside-season differences for several parameters, underscores a potential of this method. For example, with the present research aim, a paired design may be superior to a separate control group. However, for further work on the present histology-based blood leukocyte profiling, groups of healthy individuals (and other patient groups) may be beneficial. As complement to lager patient/sample numbers, a sandwich approach with double sampling may be considered.
Conclusions
Using a novel methodology for robust mapping of circulating leukocytes, our study provides evidence of complex and parallel Th2 and Th17 immune signatures in seasonal AR and a surprisingly robust upregulation of blood basophil markers GATA2 and CPA3. Further studies applying these and other markers to larger patient cohorts are suggested to provide valuable insight into the immunological complexity of AR and other inflammatory conditions.
Author contributions
LG, FN, and JE conceived and designed the clinical study design. LG and FN coordinated the patient selection and clinical sampling. PS, MM, and JE designed the histological studies. PJ, MM, PS, and JE validated the leukocyte preparation protocol. PS, GE, and MM performed the histological experiments and quantifications. PS, FN, and JE analysed and interpreted the data and wrote the manuscript. LG and LB contributed with data interpretation and manuscript editing. All authors read and approved the final manuscript.
Acknowledgements
The authors thank Karin Janser, Britt-Marie Nilsson, and Daisy Bornesund for coordinating sample preparations and for skilful technical assistance. The authors thank Charlotte Cervin‐Hoberg and Lena Glantz-Larsson for organizing patient recruitment and blood sampling.
Disclosure statement
No potential conflict of interest was reported by the author(s).
Data availability statement
The datasets used and/or analyzed during the current study are available from the corresponding author on reasonable request.
Additional information
Funding
References
- Bousquet J, Anto JM, Bachert C, et al. Allergic rhinitis. Nat Rev Dis Primers. 2020;6(1):95.
- Meng Y, Wang C, Zhang L. Recent developments and highlights in allergic rhinitis. Allergy. 2019;74(12):2320–2328.
- Zhang Y, Xi L, Gao Y, et al. Omalizumab is effective in the preseasonal treatment of seasonal allergic rhinitis. Clin Transl Allergy. 2022;12(1):e12094.
- Weinstein SF, Katial R, Jayawardena S, et al. Efficacy and safety of dupilumab in perennial allergic rhinitis and comorbid asthma. J Allergy Clin Immunol. 2018;142(1):171–177 e1.
- Bousquet PJ, Bachert C, Canonica GW, et al. Uncontrolled allergic rhinitis during treatment and its impact on quality of life: a cluster randomized trial. J Allergy Clin Immunol. 2010;126(3):666–668. e1–5.
- Segboer CL, Fokkens WJ, Terreehorst I, et al. Endotyping of non-allergic, allergic and mixed rhinitis patients using a broad panel of biomarkers in nasal secretions. PLoS One. 2018;13(7):e0200366.
- Arebro J, Ekstedt S, Hjalmarsson E, et al. A possible role for neutrophils in allergic rhinitis revealed after cellular subclassification. Sci Rep. 2017;7:43568.
- Gu ZW, Wang YX, Cao ZW. Neutralization of interleukin-17 suppresses allergic rhinitis symptoms by downregulating Th2 and Th17 responses and upregulating the Treg response. Oncotarget. 2017; Apr 48(14):22361–22369.
- Wang WY, Boot JD, Mascelli MA, et al. Comparison of biomarkers between allergic rhinitis only and allergic rhinitis with concomitant asthma. Allergy. 2009;64(7):1102–1103.
- Greiff L, Andersson M, Erjefalt JS, et al. Airway microvascular extravasation and luminal entry of plasma. Clin Physiol Funct Imaging. 2003;23(6):301–306.
- Jordakieva G, Jensen-Jarolim E. The impact of allergen exposure and specific immunotherapy on circulating blood cells in allergic rhinitis. World Allergy Organ J. 2018;11(1):19.
- Zhang H, Cardell LO, Bjorkander J, et al. Comprehensive profiling of peripheral immune cells and subsets in patients with intermittent allergic rhinitis compared to healthy controls and after treatment with glucocorticoids. Inflammation. 2013;36(4):821–829.
- Ahlstrom-Emanuelsson CA, Greiff L, Andersson M, et al. Eosinophil degranulation status in allergic rhinitis: observations before and during seasonal allergen exposure. Eur Respir J. 2004;24(5):750–757.
- Malm-Erjefalt M, Greiff L, Ankerst J, et al. Circulating eosinophils in asthma, allergic rhinitis, and atopic dermatitis lack morphological signs of degranulation. Clin Exp Allergy. 2005;35(10):1334–1340.
- Pretolani M, Bergqvist A, Thabut G, et al. Effectiveness of bronchial thermoplasty in patients with severe refractory asthma: clinical and histopathologic correlations. J Allergy Clin Immunol. 2017;139(4):1176–1185.
- Korosec P, Turner PJ, Silar M, et al. Basophils, high-affinity IgE receptors, and CCL2 in human anaphylaxis. J Allergy Clin Immunol. 2017;140(3):750–758 e15.
- Samorapoompichit P, Kiener HP, Schernthaner GH, et al. Detection of tryptase in cytoplasmic granules of basophils in patients with chronic myeloid leukemia and other myeloid neoplasms. Blood. 2001;98(8):2580–2583.
- Bullens DM, Truyen E, Coteur L, et al. IL-17 mRNA in sputum of asthmatic patients: linking T cell driven inflammation and granulocytic influx? Respir Res. 2006;7(1):135.
- Ray A, Kolls JK. Neutrophilic inflammation in asthma and association with disease severity. Trends Immunol. 2017;38(12):942–954.
- Delemarre T, Holtappels G, De Ruyck N, et al. A substantial neutrophilic inflammation as regular part of severe type 2 chronic rhinosinusitis with nasal polyps. J Allergy Clin Immunol. 2021;147(1):179–188 e2.
- Caruso M, Cibella F, Emma R, et al. Basophil biomarkers as useful predictors for sublingual immunotherapy in allergic rhinitis. Int Immunopharmacol. 2018;60:50–58.
- Salter BM, Nusca G, Tworek D, et al. Expression of activation markers in circulating basophils and the relationship to allergen-induced bronchoconstriction in subjects with mild allergic asthma. J Allergy Clin Immunol. 2016; Mar137(3):936–938 e7.