ABSTRACT
A method is reported for the determination of diphenylamine and butylated hydroxytoluene in mineral and synthetic oil. The procedure used differential pulse voltammetry with a glassy carbon electrode. This method was then used for determining these antioxidants in supporting electrolyte consisting of dilute sulfuric acid and sodium dodecyl sulfonate in ethanol. Anodic peaks were obtained for both analytes. Oxidation peaks at 250 mV were observed from a mixture of butylated hydroxytoluene and dinonyl diphenylamine, allowing their simultaneous determination. This approach was successfully used for the determination of dinonyl diphenylamine and butylated hydroxytoluene in fortified mineral and synthetic oils with good accuracy and precision.
Introduction
The role of lubricating oil in the operation of mechanical components is analogous to blood in the human body. Hence, lubricating oil may be considered to be the blood of mechanical operation. Lubricating oils in the presence of oxygen, high temperatures, and pressures may thus be susceptible to degradation (Lansdown Citation2004). The decomposition of lubricants is primarily due to their oxidation to form acids and insoluble resins like varnishes, gums, and gels. The production of acids first increases the hydrogen ion concentration of oil, which leads to corrosion. The subsequent formation of insoluble resins increases the viscosity and inhibits lubrication (Bakunin and Parenago Citation1992). Hence, the improvement of the stability of lubricating oils to suppress their breakdown is usually performed by additives. Previous studies (Jun-bing, Aguilar, and Donnelly Citation2009; Rudnick Citation2009; Wu et al. Citation2013) have shown that the presence of natural or synthetic antioxidants in lubricants results in the enhancement of stability and extension of the lifetime of lubricants. However, the effectiveness of antioxidants decreases with operating time. These phenomena reduce the stability of lubricants and decrease the effectiveness. Hence, the lubricating oil is ineffective and jeopardizes the service life of equipment. The process of decreasing the effectiveness of antioxidants and lowering the lubricity is usually accompanied by changes in physical and chemical properties of the lubricants. Hence, the determination of antioxidant concentration allows effective estimation of the lifetime of the lubricants, preventing damage to machinery and unnecessary waste of lubricating oils from premature renewal (Kauffman Citation1994).
There are many techniques available for determining these antioxidants. These include: infrared spectroscopy (de Lira et al. Citation2010; Gracia et al. Citation2011), spectrophotometry (Prasad et al. Citation1987; Capitán-Vallvey, Valencia, and Nicolás Citation2004), and liquid chromatography (Perrin and Meyer Citation2002; Saad et al. Citation2007; Tarola, Milano, and Giannetti Citation2007; Wang et al. Citation2012, Citation2014). These techniques may be incorporated with electrochemical detection, mass spectrometry, or gas chromatography (Ivanovic, Guernet-Nivaud, and Guernet Citation1990; González, Gallego, and Valcárcel Citation1999; González, Gallego, and Valcárcel Citation1999; Guan et al. Citation2006; Delgado-Zamarreno et al. Citation2007; Dytkiewitz and Morlock Citation2008; Seiss et al. Citation2009; del Nogal Sánchez et al. Citation2010; Loegel et al. Citation2014). Generally, infrared spectroscopy responds to all components in lubricant, including the base stock. As the base stock affects the determination of antioxidants, the use of infrared spectroscopy to determine antioxidants poses constraints. In addition, chromatography methods include complex procedures that may involve the use of toxic solvents in sample preparation. Consequently, these methods are expensive and may include safety and health hazards.
Voltammetric methods are potential alternatives to infrared spectroscopy and chromatography for the determination of antioxidants that offer rapid analysis with relatively low-cost instrumentation (Ni, Wang, and Kokot Citation2000; Michalkiewicz, Mechanik, and Malyszko Citation2004). In addition, these procedures have minimal safety and health hazards. Caramit et al. (Citation2013) and Tomášková et al. (2014) have described voltammetric procedures for the determination of phenolic antioxidants in lubricating oils. Caramit et al. (Citation2013) simultaneously determined tert-butyl hydroquinone and butyl hydroxyanisole in biodiesel using a screen-printed electrode in a Britton–Robinson buffer (0.04 mol L−1) containing methanol (2.0%) and cetyltrimethylammonium bromide. Tomášková et al. (Citation2014) simultaneously determined butylated hydroxyanisole and butylated hydroxytoluene in mineral and synthetic oil by linear-sweep voltammetry using a gold disc electrode with dilute H2SO4 and isopropanol. They resolved the partial overlaps of the signals by a mathematical treatment specifically for the corresponding voltammograms. Their method required the mineral and synthetic oils to be treated with 96% ethanol prior to analysis.
Amine-based antioxidants have also been reported in the literature. Chýlková et al. (Citation2010) simultaneously determined butylated hydroxytoluene and N-phenyl-1-naphthylamine in lubricating oil with a supporting electrolyte of 0.2 mol L−1 H2SO4 in 3:1 ethanol and acetonitrile by linear-sweep voltammetry and fast-scan differential pulse voltammetry. The amines were directly determined in the supporting electrolyte. It was necessary to derivatize the amines to prevent interferences to accurately determine the phenolic antioxidants.
Here a voltammetric method is reported using a new supporting electrolyte and a glassy carbon electrode to determine dinonyl diphenylamine and butylated hydroxytoluene without special mathematical treatment. The relevant analytical parameters were optimized, and the procedure was used for the analysis of fortified mineral and synthetic oil.
Experimental
Chemicals and reagents
All chemicals were of analytical reagent grade and purchased commercially from Chinese suppliers. A stock solution of 1.6 m g mL−1 butylated hydroxytoluene was prepared by dissolving butylated hydroxytoluene in 96% ethanol. A stock solution of 1.6 m g mL−1 dinonyl diphenylamine was also prepared in 96% ethanol. Electrochemical measurements were performed in a supporting electrolyte composed of dilute H2SO4, ethanol, and sodium dodecyl sulfonate. The volume ratio between the dilute H2SO4 (50%, m/m) and ethanol was 1:74, and the concentration of sodium dodecyl sulfonate was 0.75 m g mL−1. The base stocks were pure mineral oil, synthetic oil 1427, and synthetic oil 1938. All measurements were performed using double-deionized water.
Instrumentation
Voltammetric analyses of antioxidants were conducted using an electrochemical analyzer (model “Parstat 2273,” Princeton Applied Research, USA) in conjunction with a three-electrode cell. The three-electrode cell consisted of 2-mm glassy carbon as the working electrode, Ag/AgCl (3 mol L−1 KCl) as the reference electrode, and a 2 × 5 mm Pt-plate as the counter electrode. The glassy carbon electrode was carefully polished by a cloth containing 0.05 µm alumina and 70% isopropanol. The polished electrode was activated by cleaning surface active groups by cyclic voltammetry and rinsed with ethanol and distilled water before each measurement. Furthermore, the reference and auxiliary electrodes were also washed with distilled water prior to use.
Procedure
Differential pulse voltammograms were recorded for 10 mL of supporting electrolyte and the antioxidant test solution. The electrodes were mechanically polished between measurements.
The limits of detection and quantification were determined by 3S/b and 10S/b, respectively, where S is the standard deviation of 10 voltammograms of the blank and b is the slope of the calibration curve (Ziyatdinova, Khuzina, and Budnikov Citation2012). The limits of detection and limits of quantification demonstrated that the sensitivity of the method was satisfactory.
Samples and sample preparation
Fortified mineral and synthetic oil were prepared by the addition of a known amount of antioxidant. Isolation of the antioxidants in the mineral and synthetic oil was performed by extraction. This procedure involved the addition of 0.5 to 1.0 g of oil to 10 mL of 96% ethanol with sonication for 15 min. The upper layer was isolated ensuring that the antioxidants were free of oil residue, and 1 mL of the upper layer of the extracted antioxidants was added to the supporting electrolyte.
Results and discussion
As oil may contain various antioxidants, phenolic antioxidants and amine antioxidants may better stabilize oils than only one type of stabilizer (Duangkaewmanee and Petsom Citation2011). Hence, the model mixtures to be studied here were butylated hydroxytoluene as a representative of phenolic antioxidants and dinonyl diphenylamine as an example of amine antioxidant.
Investigation of the electrochemistry of dinonyl diphenylamine
The relevant parameters for differential pulse voltammetry were optimized for the determination of dinonyl diphenylamine. The evaluation criterion for the selection is that the oxidation peak height of antioxidant is higher at the same concentration. The optimization of the parameters was investigated using 61.54 µg ml−1 dinonyl diphenylamine in supporting electrolyte. The range of conditions and optimized values for differential pulse voltammetry are listed in .
Table 1. Investigated differential pulse voltammetry parameters and their optimum values for the determination of dinonyl diphenylamine.
Differential pulse voltammetry was used to determine dinonyl diphenylamine, and the results are shown in . The peak oxidation potential of dinonyl diphenylamine was at 780 mV. A linear relationship was obtained between the concentration of dinonyl diphenylamine and peak height that was given by
Figure 1. Differential pulse voltammograms of various concentrations of dinonyl diphenylamine (µg mL−1) at a glassy carbon electrode in supporting electrolyte: (1) 0, (2) 15.84, (3) 31.37, (4) 46.6, (5) 61.54, (6) 90.57, (7) 118.52, (8) 132.11, and (9)160.
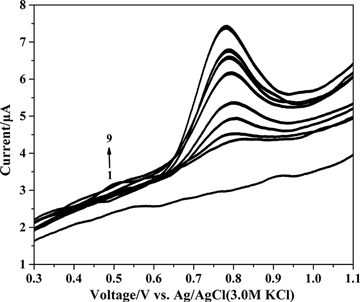
The repeatability of differential pulse voltammetry was determined for this system. Validation was performed by six differential pulse voltammetry measurements with the glassy carbon electrode in the supporting electrolyte, as shown in . The supporting electrolyte was composed of 61.54 µg mL−1 of dinonyl diphenylamine. The standard deviation was 2.20% for dinonyl diphenylamine. The reproducibility was evaluated for the measurements collected over a period of 5 days. The relative standard deviation was 1.86%.
Determination of dinonyl diphenylamine in mineral and synthetic oil
The method was used for determining dinonyl diphenylamine in fortified mineral and synthetic oil. The concentration of dinonyl diphenylamine in the oil was reported as the average of three replicates as shown in . Good accuracy was obtained with low values for the relative standard deviation. As there were no differences between the measured values and added concentrations of dinonyl diphenylamine in mineral and synthetic oil, the accuracy and precision of the specific voltammetric method are thus further demonstrated. Hence, the developed procedure may be used for the determination of this antioxidant in oil.
Table 2. Determination of dinonyl diphenylamine in oil by differential pulse voltammetry (n = 3).
Simultaneous determination of dinonyl diphenylamine and butylated hydroxytoluene
Caramit et al. (Citation2015), Tomášková et al. (Citation2013), Chýlková et al. (Citation2015), Freitas and Fatibello-Filho (Citation2010), and Medeiros, Rocha-Filho, and Fatibello-Filho (Citation2010) have shown that the type of working electrode and voltammetric method chosen are important for the simultaneous determination of antioxidants. Hence, the working electrode and voltammetric method were selected for the simultaneous determination of dinonyl diphenylamine and butylated hydroxytoluene. The dinonyl diphenylamine and butylated hydroxytoluene were simultaneously determined by linear scan voltammetry with glassy carbon and gold disc electrodes, as shown in . The peak oxidation potentials for dinonyl diphenylamine and butylated hydroxytoluene were too close by linear scan voltammetry with glassy carbon and gold disc electrodes. This interference hindered the simultaneous determination of the antioxidants. shows the simultaneous determination of dinonyl diphenylamine and butylated hydroxytoluene by differential pulse voltammetry method using glassy carbon and gold disc electrodes. The peak oxidation potential between dinonyl diphenylamine and butylated hydroxytoluene determined by differential pulse voltammetry was larger with the glassy carbon electrode compared with the gold disc electrode. Differential pulse voltammetry gave peak oxidation potentials at 798 mV for dinonyl diphenylamine and at 1045 mV for butylated hydroxytoluene. The 250 mV separation of the peak potentials clearly facilitated the simultaneous determination of the analytes. Hence, differential pulse voltammetry was used with a glassy carbon electrode for the simultaneous determination of dinonyl diphenylamine and butylated hydroxytoluene. The parameters for differential pulse voltammetry were optimized to include a pulse amplitude of 100 mV, a modulation time of 20 ms, and a scan rate of 20 mV s−1.
Figure 3. Linear scan voltammograms of 72.73 µg mL−1 dinonyl diphenylamine and 72.73 µg mL−1 butylated hydroxytoluene in supporting electrolyte on the (1) glassy carbon electrode and (2) gold disc electrode.
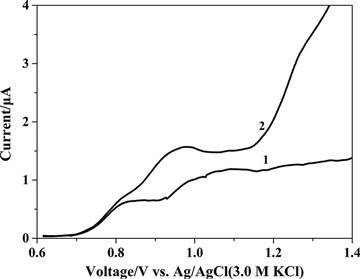
Figure 4. Differential pulse voltammograms for the simultaneous determination of 72.73 µg mL−1 dinonyl diphenylamine and 72.73 µg mL−1 butylated hydroxytoluene in supporting electrolyte on the (1) glassy carbon electrode and (2) gold disc electrode.
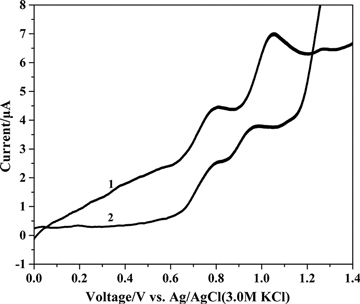
Subsequently, differential pulse voltammetry was used to determine dinonyl diphenylamine and butylated hydroxytoluene (). shows that a linear calibration relationship was observed from 15 to 145 µg mL−1 for dinonyl diphenylamine and butylated hydroxytoluene. The relationships between the electrochemical signal and concentration were given by
The limits of detection and quantification for dinonyl diphenylamine and butylated hydroxytoluene were 2.07 and 6.91 µg mL−1, and 1.01 and 3.36 µg mL−1, respectively.
Figure 5. Differential pulse voltammograms on the glassy carbon electrode for various concentrations of dinonyl diphenylamine and butylated hydroxytoluene (µg mL−1) in supporting electrolyte. (1) 0, (2) 15.69, (3) 30.77, (4) 59.26, (5) 72.73, (6) 85.71, (7) 98.24, (8) 110.34, (9) 122.03, (10) 133.33, and (11) 144.26.
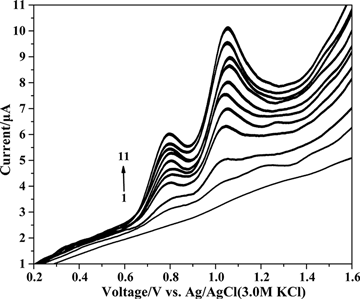
Further validation of repeatability was performed by 10 differential pulse voltammetry measurements with the glassy carbon electrode in supporting electrolyte containing 85.71 µg mL−1 of dinonyl diphenylamine and butylated hydroxytoluene. These measurements provided relative standard deviations of 3.86 and 2.92%, respectively (). The reproducibility of the method was subsequently evaluated by measuring the oxidation current values within 8 days, which gave relative standard deviations of 4.01 and 6.05% for dinonyl diphenylamine and butylated hydroxytoluene, respectively.
Simultaneous determination of dinonyl diphenylamine and butylated hydroxytoluene in mineral and synthetic oil
The developed method was used to analyze fortified mineral and synthetic oil mixed with dinonyl diphenylamine and butylated hydroxytoluene. The results are shown in . Low relative standard deviation values were achieved with good agreement between the added and measured values of dinonyl diphenylamine and butylated hydroxytoluene. The accuracy and precision of the voltammetric method demonstrates its application for the simultaneous determination of these antioxidants in oil.
Table 3. Simultaneous determination of dinonyl diphenylamine and butylated hydroxytoluene in oil by differential pulse voltammetry (n = 3).
Conclusion
Differential pulse voltammetry was used for the determination of dinonyl diphenylamine individually and simultaneously with butylated hydroxytoluene in oil. The method was used with a glassy carbon working electrode to provide suitable precision for the oxidation in supporting electrolyte that included sodium dodecyl sulfate, ethanol, and diluted H2SO4. The extraction of antioxidants from the oil samples involved the addition of 0.5–1.0 g of oil into 10 mL of 96% ethanol with ultrasonic treatment for 15 min followed by separation of the upper layer to isolate the antioxidants from the oil residue.
The results for the determination of dinonyl diphenylamine separately from supporting electrolyte suggested that the method was suitable for the analysis of mineral and synthetic oil. For the simultaneous determination of dinonyl diphenylamine and butylated hydroxytoluene, differential pulse voltammetry gave peak oxidation potentials for dinonyl diphenylamine at 798 mV and for butylated hydroxytoluene at 1045 mV. The separation of the peak potential by 250 mV allowed the simultaneous determination of both analytes with low relative standard deviations, good recovery, good sensitivity, and suitable precision and accuracy.
In conclusion, the method reported in this study was used to rapidly determine dinonyl diphenylamine alone and in the presence of butylated hydroxytoluene with satisfactory accuracy and precision. Moreover, comparison of differential pulse voltammetry with chromatography methods suggests that the former is simpler to operate and uses less expensive instrumentation. The results show the developed method may be used for the analysis of petroleum products and monitoring the degradation of oil.
Conflict of interest
The authors declare no competing financial interest.
References
- Bakunin, V. N., and O. P. Parenago. 1992. A mechanism of thermo-oxidative degradation of polyol ester lubricants. Journal of Synthetic Lubrication 9: 127–43. doi:10.1002/jsl.3000090204
- Capitán-Vallvey, L. F., M. C. Valencia, and E. A. Nicolás. 2004. Solid-phase ultraviolet absorbance spectrophotometric multisensor for the simultaneous determination of butylated hydroxytoluene and co-existing antioxidants. Analytica Chimica Acta 503: 179–86. doi:10.1016/j.aca.2003.10.027
- Caramit, R. P., A. S. A. Araújo, D. K. Fogliatto, L. H. Viana, M. A. G. Trindade, and V. S. Ferreira. 2015. Carbon-nanotube-modified screen-printed electrodes, a cationic surfactant, and a peak deconvolution procedure: Alternatives to provide satisfactory simultaneous determination of three synthetic antioxidants in complex samples. Analytical Methods 7: 3764–71. doi:10.1039/c4ay02875a
- Caramit, R. P., A. G. de Freitas Andrade, J. B. G. de Souza, T. A. de Araujo, L. H. Viana, M. A. G. Trindade, and V. S. Ferreira. 2013. A new voltammetric method for the simultaneous determination of the antioxidants TBHQ and BHA in biodiesel using multi-walled carbon nanotube screen-printed electrodes. Fuel 105: 306–13. doi:10.1016/j.fuel.2012.06.062
- Chýlková, J., O. Machalický, M. Tomášková, R. Šelešovská, and T. Navrátil. 2015. Voltammetric determination of nitro derivative of synthetic antioxidant 2, 6-di-tert-butyl-4-methyl-phenol. Analytical Letters 49: 92–106. doi:10.1080/00032719.2015.1007376 (just-accepted).
- Chýlková, J., R. Šelešovská, J. Machalíková, and L. Dušek. 2010. Differentiation between phenol-and amino-substances in voltammetry determination of synthetic antioxidants in oils. Central European Journal of Chemistry 8: 607–16. doi:10.2478/s11532-010-0024-0
- de Lira, L. F. B., M. S. de Albuquerque, J. G. A. Pacheco, T. M. Fonseca, E. H. de Siqueira Cavalcanti, L. Stragevitch, and M. F. Pimentel. 2010. Infrared spectroscopy and multivariate calibration to monitor stability quality parameters of biodiesel. Microchemical Journal 96: 126–31. doi:10.1016/j.microc.2010.02.014
- del Nogal Sánchez, M., P. Glanzer, J. L. P. Pavón, C. G. Pinto, and B. M. Cordero. 2010. Determination of antioxidants in new and used lubricant oils by headspace-programmed temperature vaporization-gas chromatography-mass spectrometry. Analytical and Bioanalytical Chemistry 398: 3215–24. doi:10.1007/s00216-010-4248-9
- Delgado-Zamarreno, M. M., I. Gonzalez-Maza, A. Sanchez-Perez, and R. C. Martínez. 2007. Analysis of synthetic phenolic antioxidants in edible oils by micellar electrokinetic capillary chromatography. Food Chemistry 100: 1722–27. doi:10.1016/j.foodchem.2005.10.018
- Duangkaewmanee, S., and A. Petsom. 2011. Synergistic and antagonistic effects on oxidation stability of antioxidants in a synthetic ester based oil. Tribology International 44: 266–71. doi:10.1016/j.triboint.2010.10.028
- Dytkiewitz, E., and G. E. Morlock. 2008. Analytical strategy for rapid identification and quantification of lubricant additives in mineral oil by high-performance thin-layer chromatography with UV absorption and fluorescence detection combined with mass spectrometry and infrared spectroscopy. Journal of AOAC International 91: 1237–44.
- Freitas, K. H. G., and O. Fatibello-Filho. 2010. Simultaneous determination of butylated hydroxyanisole (BHA) and butylated hydroxytoluene (BHT) in food samples using a carbon composite electrode modified with Cu3(PO4)2 immobilized in polyester resin. Talanta 81: 1102–08. doi:10.1016/j.talanta.2010.02.004
- González, M., M. Gallego, and M. Valcárcel. 1999. Gas chromatographic flow method for the preconcentration and simultaneous determination of antioxidant and preservative additives in fatty foods. Journal of Chromatography A 848: 529–36. doi:10.1016/s0021-9673(99)00411-2
- Gracia, N., S. Thomas, F. Thibault-Starzyk, O. Lerasle, and L. Duponchel. 2011. Combination of mid-infrared spectroscopy and curve resolution method to follow the antioxidant action of alkylated diphenylamines. Chemometrics and Intelligent Laboratory Systems 106: 210–15. doi:10.1016/j.chemolab.2010.08.017
- Guan, Y., Q. Chu, L. Fu, T. Wu, and J. Ye. 2006. Determination of phenolic antioxidants by micellar electrokinetic capillary chromatography with electrochemical detection. Food Chemistry 94: 157–62. doi:10.1016/j.foodchem.2005.01.015
- Ivanovic, D., E. Guernet-Nivaud, and M. Guernet. 1990. Determination of some antioxidants by derivative spectrophotometric method and high-performance liquid chromatography. Analytical Letters 23: 1123–37. doi:10.1080/00032719008053451
- Jun-bing, Y. A. O., G. Aguilar, and S. G. Donnelly. 2009. Study on the synergistic effects of the antioxidants for lubricant. Lubricating Oil 4: 015.
- Kauffman, R. E. 1994. CRC handbook of lubrication and tribology. In Rapid determination of remaining useful lubricant life, ed. R. E. Kauffman, 89–99. Scotia, New York: CRC Press.
- Lansdown, A. R. 2004. Lubrication and lubricant selection: A practical guide. In Lubricating oils, ed. A. R. Lansdown, 31–41. London and Bury St Edmunds, UK: ASME Press.
- Loegel, T. N., R. E. Morris, K. M. Myers, and C. J. Katilie. 2014. Analysis of phenolic antioxidants in navy mobility fuels by gas chromatography–mass spectrometry. Energy & Fuels 28: 6267–74. doi:10.1021/ef5013984
- Medeiros, R. A., R. C. Rocha-Filho, and O. Fatibello-Filho. 2010. Simultaneous voltammetric determination of phenolic antioxidants in food using a boron-doped diamond electrode. Food Chemistry 123: 886–91. doi:10.1016/j.foodchem.2010.05.010
- Michalkiewicz, S., M. Mechanik, and J. Malyszko. 2004. Voltammetric study of some synthetic antioxidants on platinum microelectrodes in acetic acid medium. Electroanalysis 16: 588–95. doi:10.1002/elan.200302826
- Ni, Y., L. Wang, and S. Kokot. 2000. Voltammetric determination of butylated hydroxyanisole, butylated hydroxytoluene, propyl gallate and tert-butylhydroquinone by use of chemometric approaches. Analytica Chimica Acta 412: 185–93. doi:10.1016/s0003-2670(00)00720-0
- Perrin, C., and L. Meyer. 2002. Quantification of synthetic phenolic antioxidants in dry foods by reversed-phase HPLC with photodiode array detection. Food Chemistry 77: 93–100. doi:10.1016/s0308-8146(01)00373-9
- Prasad, U. V., T. E. Divakar, K. Hariprasad, and C. S. P. Sastry. 1987. Spectrophotometric determination of some antioxidants in oils and fats. Food Chemistry 25: 159–64. doi:10.1016/0308-8146(87)90065-3
- Rudnick, L. R. 2009. Lubricant additives: Chemistry and applications. Antioxidants, ed. D. Jun and A. M. Cyril, 4–5. Wilmington, DE, USA: CRC Press.
- Saad, B., Y. Y. Sing, M. A. Nawi, N. Hashim, A. S. M. Ali, M. I. Saleh, and K. Ahmad. 2007. Determination of synthetic phenolic antioxidants in food items using reversed-phase HPLC. Food Chemistry 105: 389–94. doi:10.1016/j.foodchem.2006.12.025
- Seiss, M., C. Langer, R. Hickel, and F. X. Reichl. 2009. Quantitative determination of TEGDMA, BHT, and DMABEE in eluates from polymerized resin-based dental restorative materials by use of GC/MS. Archives of Toxicology 83: 1109–15. doi:10.1007/s00204-009-0470-7
- Tarola, A. M., F. Milano, and V. Giannetti. 2007. Simultaneous determination of phenolic compounds in red wines by HPLP-UV. Analytical Letters 40: 2433–45. doi:10.1080/00032710701577666
- Tomášková, M., J. Chýlková, V. Jehlička, T. Navrátil, I. Švancara, and R. Šelešovská. 2014. Simultaneous determination of BHT and BHA in mineral and synthetic oils using linear scan voltammetry with a gold disc electrode. Fuel 123: 107–12. doi:10.1016/j.fuel.2014.01.052
- Tomášková, M., J. Chýlková, O. Machalický, R. Šelešovská, and T. Navrátil. 2013. Voltammetric determination of different antioxidants in petroleum products by working gold electrode. International Journal of Electrochemical Science 8: 1664–77.
- Wang, J. Y., H. L. Wu, Y. Chen, Y. M. Sun, Y. J. Yu, X. H. Zhang, and R. Q. Yu. 2012. Fast analysis of synthetic antioxidants in edible vegetable oil using trilinear component modeling of liquid chromatography-diode array detection data. Journal of Chromatography A 1264: 63–71. doi:10.1016/j.chroma.2012.09.070
- Wang, J. Y., H. L. Wu, Y. M. Sun, H. W. Gu, Z. Liu, Y. J. Liu, and R. Q. Yu. 2014. Simultaneous determination of eight flavonoids in propolis using chemometrics-assisted high performance liquid chromatography-diode array detection. Journal of Chromatography B 947: 32–40. doi:10.1016/j.jchromb.2014.05.027
- Wu, Y., W. Li, M. Zhang, and X. Wang. 2013. Improvement of oxidative stability of trimethylolpropane trioleate lubricant. Thermochimica Acta 569: 112–18. doi:10.1016/j.tca.2013.05.033
- Ziyatdinova, G., A. Khuzina, and H. Budnikov. 2012. Determination of sterically hindered phenols and α-tocopherol by cyclic voltammetry. Analytical Letters 45: 1670–85. doi:10.1080/00032719.2012.677788