Abstract
Capsule Using game birds as surrogate carcasses for raptors could significantly bias calibration searches for wind turbine collision fatalities.
Increasing global wind power capacity and developing wind energy markets in all economic regions offers the potential to significantly reduce greenhouse gas emissions in the near (2020) and long term (2050) (Wiser et al. Citation2011, REN21 Citation2014). However, inappropriately sited wind turbine arrays are a demonstrated cause of substantial avian collision mortality (Barrios & Rodriguez Citation2004, Everaert & Stienen Citation2007, Follestad et al. Citation2007, Lekuona & Ursúa Citation2007, Smallwood & Thelander Citation2008) and, as the industry expands, it is increasingly important to provide accurate site-specific bird mortality estimates to evaluate population effects, and inform collision mitigation and species-specific conservation strategies. A principal bias affecting fatality estimates obtained from routine carcass search surveys at extant wind farms is the rate at which carcasses are removed by scavengers (Smallwood Citation2007, Ponce et al. Citation2010). To quantify this bias, experimental trials to assess carcass persistence are commonly conducted using readily available surrogate species to represent the species of interest at a particular wind farm. Collating results from a number of geographically widespread carcass persistence studies within the USA, Smallwood (Citation2007) reported that carcass persistence varied substantially amongst bird species, and highlighted a mismatch in carcass persistence between large raptors and any of the frequently used non-raptor surrogates. However, this review had limited access to trials involving persistence of raptor and non-raptor surrogates at the same site and under the same conditions. Here, we conducted concurrent carcass persistence trials for Buzzard Buteo buteo and Pheasant Phasianus colchicus surrogates, specifically to assess their suitability for calibrating Red Kite Milvus milvus collision mortality at the extant Braes of Doune wind farm, Stirlingshire, Scotland, and more generally to inform the design of studies that use searches for dead birds as a measure of fatality rates.
Buzzard and Pheasant carcasses were randomly assigned to trial plots of the same size (130 m by 130 m) as those searched for collision victims under turbines, one kilometre east of the Braes of Doune wind farm. The wind farm and trial plots were all sited between 470 and 490 m above sea level and shared similar vegetation and terrain characteristics, principally heather (Erica sp. or Calluna vulgaris) with deep (>2 m) peat hags.
We assumed that Buzzard and Pheasant were reasonable surrogates for Red Kite based on their similar size and weight and, following Kerns, Erickson & Arnett (Citation2005), we used fresh rather than frozen carcasses to better reflect realistic removal rates. All Pheasant carcasses were obtained from Argaty Estate, Doune and the Buzzard carcasses were provided by The Scottish Agricultural Science Agency. Handling was always performed with latex gloves and facemasks for Health and Safety reasons and to reduce the likelihood of carcasses becoming contaminated with human odour.
We conducted trials across two seasons, one between January and April, the other between September and December, referred to here as ‘spring’ and ‘autumn’ trials, respectively. We assumed, based on previous observations, that Red Fox Vulpes vulpes would be the principal scavenger and so attempted to provide consistency in scavenger activity levels by timing each trial to coincide with high activity periods within the fox breeding cycle; cubbing for the spring trial and presence of dispersed/dispersing young for the autumn trial.
We distributed carcasses randomly within trial plots. Each carcass was identified with a leg ring or metal tag and its location logged with a handheld GPS.
We used 40 Buzzard and 56 Pheasant carcasses; 13 Buzzard and 30 Pheasant for the spring trial and 27 Buzzard and 26 Pheasant for the autumn trial. All carcasses were placed within trial plots on the first day of each trial and carcass persistence was determined by searching at each carcass location to check for moved carcasses within the plot. Trial plot searches were made on days 1, 3, 5, 10, 15, 20, 25, then every 10 days up to the end of a 95-day period. Carcasses not found during a search or where the only evidence was a feather patch on the ground were recorded as removed by this search day.
Survival analysis routines were used to examine the carcass persistence results from the trial plots (Cox & Oakes Citation1984). The probability of a carcass surviving beyond a given visit day was estimated for the whole sample and seasonal subsamples using the Kaplan–Meier estimator () and a Log-Rank Test used to test for differences between survival curves generated for these estimates (Kaplan & Meier Citation1958). The Kaplan–Meier estimator does not allow effects of covariates on carcass persistence to be modelled. Carcass persistence is analogous with nest survival data and so we used a likelihood-based nest survival module in ‘program MARK’ (White & Burnham Citation1999) to evaluate the effects of species and season on carcass survival rates by testing the relative performance of a set of competing survival models that included covariates of season and species. We used Akaike's Information Criterion, corrected for a small sample size (AICc), to rank competing models, with the model best supporting the data identified as that with the lowest (AICc) value. The associated Akaike weight (Wi) was used to quantify the degree of support between competing models and we assumed that there was support for a difference between two models if the AICc values differed by greater than 2.0 (). We did not standardize covariates and used the default sine and logit link functions for constant survival and covariate models, respectively (White & Burnham Citation1999).
Figure 1. Kaplan–Meier survival estimates for Buzzard (black line) and Pheasant (red line) carcasses for (a) all data (Pheasant n = 56, Buzzard n = 40); (b) spring (Pheasant n = 30, Buzzard n = 13) and (c) autumn (Pheasant n = 26, Buzzard n = 27).
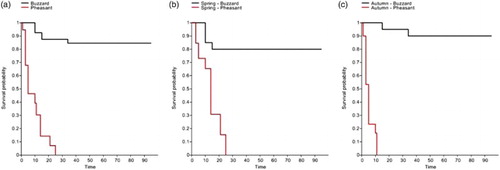
Table 1. Carcass survival models. AICc, Akaike's information criterion, corrected for small sample size; ΔAICc, difference in AICc between best-fitted model and other models; K, number of parameters, Wi, likelihood that model (i) is best model in the candidate set. S, daily survival rate.
All 56 Pheasant carcasses were removed within 25 days, and the majority (>62%) within 5 days of placement. The majority of Buzzard carcasses (85%) remained until the end of the study period (day 95) (a). Log-Rank Tests using pooled data from both trial periods showed that Buzzard carcasses persisted significantly longer than Pheasant carcasses (χ2 = 79.77, P < 0.0001). On average, Buzzard carcasses persisted for 63.53 days and Pheasant carcasses for 9.16 days. Differences in carcass persistence between species were also evident when survival curves were compared for spring and autumn trials separately (b and 1c): Buzzard carcasses persisted significantly longer than Pheasant during both the spring (χ2 = 25.58, P < 0.0001) and autumn (χ2 = 48.28, P < 0.0001) trials. Pheasant carcasses persisted for a significantly shorter period during the autumn (average 5.2 days) compared to the spring trial (average 13.7 days; χ2 = 26.29, P < 0.0001). In autumn the majority of Pheasant carcasses (77%) were removed within the first 5 days compared with 26% in the first 5 days of the spring trial. For Buzzard, the difference in persistence rates between spring and autumn trials was not significant (χ2 = 1.03, P > 0.05), with carcasses surviving an average of 57.4 days in spring and 69.65 days in autumn.
Likelihood-based survival models that included the intercept and an effect of either season or species received no support, indicating that neither season nor species effects individually provided a good fit for the data. The second best supported model included additive effects of season and species; however, including an interaction between species and season improved the model by ΔAICc 2.4. This best model had over three times more support (Wi = 0.77) than the model with only additive effects of season and species (Wi = 0.23). Foxes, the likely scavenger species in this study, exhibit food distastes that may change in response to factors such as competition for food (MacDonald Citation1977). The strong support for a model that included an interaction between season and species therefore may relate to seasonal differences in the availability of alternative food opportunities for foxes between the two trial periods.
In this study, we found a significant difference in carcass persistence rates between the Buzzard and Pheasant surrogates used to calibrate Red Kite mortality at the Braes of Doune wind farm. Buzzard carcasses persisted significantly longer than Pheasant, regardless of season. Our results agree with findings in Smallwood (Citation2007) that large raptor carcasses have a high persistence rate, substantially higher than that for a game bird species. We, therefore, reiterate the importance of matching surrogate species used in carcass removal trials with the species for which mortality estimates are required, and particularly when large raptors are the species of interest because our results suggest that game birds, while being readily available, are poor surrogates in carcass persistence trials to calibrate raptor mortality estimates.
Season also affected carcass persistence rate. We found a significant seasonal difference in carcass persistence for Pheasant and strongest support for a model where seasonal survival rate varied with species. In other carcass persistence studies (Ponce et al. Citation2010, Bispo et al. Citation2013) the presence, strength and direction of a seasonal effect varied between study locations, highlighting the site-specific nature of seasonal effects.
Accurate bird mortality estimates increase the likelihood of correctly assessing population effects on at-risk species, and assist designing appropriate collision risk mitigation and species specific conservation strategies. Until advances in surveillance technology allow collision fatalities to be reliably quantified, well designed scavenger removal trials are valuable, and because species of interest are often of high conservation status, less vulnerable species will often be required as surrogates. For all studies that use surrogate species to quantify bias in bird mortality estimates, we recommend that the at-risk and surrogate species are closely matched, and that trials should be scheduled to account for potential seasonal changes in scavenger behaviour.
ACKNOWLEDGEMENTS
The authors thank Scottish & Southern Energy for funding the carcass persistence field trials for this study, Argaty Estate and The Scottish Agricultural Science Agency for providing bird carcasses, and Phil Whitfield and an anonymous reviewer for providing comments that helped improve a previous draft.
ORCID
Blair Urquhart http://orcid.org/0000-0002-1758-0641
REFERENCES
- Barrios, L. & Rodriguez, A. 2004. Behavioural and environmental correlates of soaring-bird mortality at on-shore wind turbines. J. Appl. Ecol. 41: 72–81. doi: 10.1111/j.1365-2664.2004.00876.x
- Bispo, R., Bernardino, J., Marques, T. & Pestana, D. 2013. Modeling carcass removal time for avian mortality assessment in wind farms using survival analysis. Environ. Ecol. Stat. 20: 147–165. doi: 10.1007/s10651-012-0212-5
- Cox, D.R. & Oakes, D. 1984. Analysis of Survival Data. Chapman & Hall, London.
- Everaert, J. & Stienen, E.W.M. 2007. Impact of wind turbines on birds in Zeebrugge (Belgium). Significant effect on breeding tern colony due to collisions. Biodivers. Conserv. 16: 3345–3359. doi: 10.1007/s10531-006-9082-1
- Follestad, A., Flagstad, Ø., Nygård, T., Reitan, O. & Schulze, J. 2007. Vindkraft og fugl på Smøla 2003–2006 [Wind power and birds at Smøla 2003–2006 – English abstract]. NINA report 248. Norsk institutt for naturforskning, Trondheim.
- Kaplan, E.L. & Meier, P. 1958. Non parametric estimation from incomplete observation. J. Am. Stat. Assoc. 53: 457–481. doi: 10.1080/01621459.1958.10501452
- Kerns, J., Erickson, W.P. & Arnett, E.B. 2005. Bat and bird fatality at wind energy facilities in Pennsylvania and West Virginia. In Relationships Between Bats and Wind Turbines in Pennsylvania and West Virginia: An Assessment of Bat Fatality Search Protocols, Patterns of Fatality, and Behavioural Interactions with Wind Turbines. A final report submitted to the Bats and Wind Energy Cooperative. Bat Conservation International, Austin, Texas, 24–95.
- Lekuona, J.M. & Ursúa, C. 2007. Avian mortality in wind power plants of Navarra (northern Spain). In de Lucas, M., Janss, G. & Ferrer, M. (eds) Birds and Wind Farms: Risk Assessment and Mitigation, 177–192. Quercus, Madrid.
- MacDonald, D.W. 1977. On food preference in the red fox. Mammal Rev. 7: 7–23. doi: 10.1111/j.1365-2907.1977.tb00359.x
- Ponce, C., Alonso, J.C., Argandoña, G., García Fernández, A. & Carrasco, M. 2010. Carcass removal by scavengers and search accuracy affect bird mortality estimates at power lines. Anim. Conserv. 13: 603–612. doi: 10.1111/j.1469-1795.2010.00387.x
- REN21. 2014. Renewables 2014 Global Status Report. Paris: REN21 Secretariat.
- Smallwood, K.S. 2007. Estimating wind turbine-caused bird mortality. J. Wildl. Manage. 71: 2781–2791. doi: 10.2193/2007-006
- Smallwood, K.S. & Thelander, C. 2008. Bird mortality in the Altamont pass wind resource area, California. J. Wildl. Manage. 72: 215–223. doi: 10.2193/2007-032
- White, G.C. & Burnham, K.P. 1999. Program MARK: survival estimation from populations of marked animals. Bird Study 46: S120–S139. doi: 10.1080/00063659909477239
- Wiser, R., Yang, Z., Hand, M., Hohmeyer, O., Infield, D., Jensen, P.H., Nikolaev, V., O'Malley, M., Sinden, G. & Zervos, A. 2011. Wind Energy. In Edenhofer, O., Pichs-Madruga, R., Sokona, Y., Seyboth, K., Matschoss, P., Kadner, S., Zwickel, T., Eickemeier, P., Hansen, G., Schlömer, S. & von Stechow, C. (eds) IPCC Special Report on Renewable Energy Sources and Climate Change Mitigation, 535–608. Cambridge University Press, Cambridge.