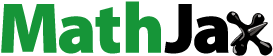
ABSTRACT
1. This study determined the effects of three protein sources (PS), each at two digestibility crude protein (DCP) levels, on performance, gut morphology and fermentation characteristics in the hindgut of broilers.
2. It was hypothesised that broilers fed ingredients high in indigestible CP, i.e. rapeseed meal (RSM) or maize gluten (MG), could potentially cause reduced growth, impaired gut health, and more protein fermentation products in caecal digesta. Increasing the DCP level in each of the indigestible CP diets may compensate for these detrimental effects.
3. In total, 288 one-d-old male Ross 308 broilers were used in a completely randomised 3 × 2 factorial design, with six replicate pens per treatment. Three PS: soybean meal (SBM), rapeseed meal (RSM) or maize gluten (MG), and two DCP levels: 15.8 and 17.2% were used.
4. Broilers fed SBM had increased feed intake and BWG and improved FCR compared with those fed RSM and MG diets. Broilers fed high DCP had better performance compared with those on low DCP. No significant effects of PS or DCP level were found on gastrointestinal tract development, caecal ammonia or volatile fatty acid concentrations.
5. Broilers fed SBM had longer villi, smaller crypts and increased villus height to crypt depth ratio compared with those fed RSM and MG diets. Broilers fed RSM diet had a lower caecal pH, and had 16.5% and 14.9% more branched chain fatty acid contents in caecal digesta compared with those fed SBM and MG diets, respectively, indicating more proteolytic fermentation.
6. Replacing SBM by RSM and MG negatively affected growth performance and gut morphology. Hindgut protein fermentation was substantially increased in RSM fed birds.
7. To a certain extent, retarded growth performance in RSM and MG fed birds could be counterbalanced by increasing the dietary level of digestible CP.
Introduction
Broilers can attain a BW of 2 kg by consuming 3 kg of feed within 35 d (Choct Citation2009). Such modern broilers have been selected to achieve a low FCR and a high growth rate. This rapid growth rate requires a high concentration of digestible protein (e.g. digestible essential amino acids). Due to the growing world human population and increasing demand for proteins for food (Alexandratos and Bruinsma Citation2012), in the coming decades increased competition for high-quality proteins between feed and food is expected. A scarcity of commonly-used protein sources for feed, e.g. soybean meal, might occur. As a consequence, the feed sector has to cope with low-quality protein sources, with increased levels of indigestible protein.
Some dietary proteins are not well digested in the small intestine, and consequently undigested dietary protein enters, together with undigested endogenous proteins, into the caeca and colon. Proteins, peptides and amino acids that bypass the ileum, are a potential substrate for fermentation by microbiota in the caeca. These undigested protein substances may stimulate the growth of N-utilising microbiota (Reid and Hillman Citation1999), leading to increased levels of toxic compounds, such as biogenic amines, phenols, and cresols (Apajalahti and Vienola Citation2016). These toxic compounds may be detrimental for bird performance and gut health (Thomke and Elwinger Citation1998). Moreover, as a result of protein fermentation, higher levels of branched chain fatty acids and elevated levels of ammonia per gram of non-starch polysaccharides (NSP) may be produced (Bikker et al. Citation2006).
Gut morphology is an important indicator of intestinal health (Zang et al. Citation2009). There is a scarcity of published data regarding the effects of protein source on gut morphology in broilers. In addition, the available data show contradictory results. Buwjoom et al. (Citation2010) found no significant effect for three different dietary CP levels (10, 16 and 22%) on villus height and crypt depth in broilers. Contrary to this, Laudadio et al. (Citation2012) observed higher villus heights in broilers fed soybean meal-based medium and high CP diets compared with those fed a low CP diet, and recommended a 20.5% dietary CP level to optimise growth performance. It is thought that feeding protein sources with similar amounts of essential amino acids, but with varying amounts of indigestible protein, may have a different impact on broilers.
It was hypothesised that broilers fed ingredients high in indigestible CP, i.e. rapeseed meal (RSM) or maize gluten (MG), would potentially show reduced growth, impaired gut health, and more protein fermentation products in the caecal digesta. Moreover, it was hypothesised that increasing DCP level to a certain extent might compensate for these negative effects, e.g. by modifying the ratio between digestible and indigestible CP, and by providing more digestible amino acids from other protein ingredients to stimulate bird performance. The current study investigated the effects of three dietary protein sources, varying in indigestible protein content, supplied at two dietary digestible CP levels on growth performance, gut morphology and hindgut protein fermentation characteristics in broiler chickens.
Materials and methods
Animal ethics
Experimental procedures were in accordance with the Wageningen University and the Netherlands Animal Experimental Committee guidelines and code of practice. Ethical approval was granted before the conduct of the study.
Bird management
In total, 288 male (Ross 308), one-d-old broilers were purchased from a commercial hatchery (Morren Breeds B.V., Lunteren, The Netherlands). Upon arrival, broilers were individually weighed and steel wing tagged before being allotted to one of 36 floor pens (eight birds per pen), equally distributed over three identical climate-controlled rooms, so that each pen had a similar initial total BW. Each pen (1.15 × 1.75 × 0.80 m; L × W × H) had three drinking nipples with a cup underneath connected to a water tank of 10 litres capacity. Pens were separated by solid walls to prevent contact between broilers from different treatment groups. A feeding tray was placed on the floor during week 1 and replaced by a feeding trough thereafter. Feed and water were available ad libitum throughout the experiment. Wood shavings were used as litter material. The lighting schedule was maintained at 23l:1d for the first three days and, thereafter, maintained at 16l:8d, with an intensity of approximately 20 lux at bird level, throughout the experimental period. During the first three days, room temperature was set at 32°C, and was gradually decreased to a constant value of 22°C in week 4, which was maintained until the end of the experiment.
Experimental design and treatments
The study was conducted as a completely randomised design (six replicate pens per treatment with eight birds per pen), and treatments were equally distributed over rooms. All birds received the same standard starter diet for the first 7 d of the experiment (CP: 21%; ME: 2895 kcal/kg). After week 1, six dietary treatments were provided which contained three different protein sources: soybean meal (SBM), rapeseed meal (RSM) or maize gluten (MG) each at 15.8% or 17.2% digestible crude protein (DCP) levels. The diets were formulated to meet or exceed the nutrient recommendations for boilers (CVB (Central Bureau for Livestock Feeding) Citation2007). These dietary treatments were randomly assigned to pens in three rooms (3 × 2 × 6 = 36). As shown in , the diets were formulated to contain different concentrations of indigestible CP, based on calculated faecal digestible protein contents. Each diet was formulated to have similar concentrations of digestible essential amino acids and to be iso-energetic, on an AMEn basis. Differences in DCP levels within PS were achieved by varying the digestible non-essential amino acid (NEAA) content, through upgrading other protein ingredients. All six dietary treatments were offered as pellets with a size of 2.5 mm for the starter and 4.0 mm for the grower diet. The diets did not contain antimicrobial growth promoters.
Table 1. Dietary ingredients, and calculated nutrient composition of the experimental diets (g/kg, as-fed basis).
Traits measured
Feed intake (FI), water intake (WI) and body weight (BW) gain per pen in each room were recorded at d 8, 15, 22, 29 and 33. Feed intake, BW gain and WI were expressed per bird per d. Weights of dead birds were determined and their BW gain and FI included in the calculation of feed conversion ratio (FCR) per pen.
At the end of the experiment (d 34), six of the eight birds per pen, selected to be closest to the average weight of the group, were euthanised by intravenous T-61 injection and the abdominal cavity opened. On the day of dissection, all birds had free access to feed and water up until the moment of euthanasia. Birds in a pen were euthanised in order of replicate number. The different parts of the gastrointestinal tract (GIT), i.e. the crop, proventriculus, gizzard, duodenum (from the pyloric junction to the pancreo-billiary duct), jejunum (from the pancreo-billiary duct to Meckle’s diverticulum), ileum (from Meckle’s diverticulum to the ileo-caecal junction), caecum (from the ostium) and colon were segmented. The digesta contents from each segment were immediately removed by gentle squeezing and the empty segments weighed. To obtain sufficient amount of material for analysis, the caecal content of six birds in a pen were quantitatively pooled, thoroughly mixed and the pH determined using a calibrated pH meter before the samples were freeze dried at −20°C pending volatile fatty acid (VFA) and ammonia analyses.
Tissue collection and histological measurements
A gut segment (approximately 2 cm) in the middle of the duodenum was excised, rinsed with cold physiological saline (0.9%) and immediately placed in Bouin’s fluid. Thereafter, the samples were transferred into 70% ethanol within 24 h. The samples were embedded in paraffin and sliced at 5 µm thickness for histological examination. Six cross-sections per bird were processed using standard haematoxylin and eosin methods, as described by Owusu-Asiedu et al. (Citation2002). Villus heights and crypt depths were measured on 10 intact, well-oriented villi (from 0.5 cm in the middle of the duodenum) per bird using a compound light microscope equipped with a video camera. Villus height was measured from the tip of the villous to the crypt-villous junction, whereas crypt depth was measured from the crypt-villous junction to the base.
Chemical analysis
Dry matter, organic matter and N contents in the experimental diets were analysed according to standard methods (AOAC International Citation2006). Ammonia-N in caecal digesta was analysed by the indole phenol-blue method (Novozamsky et al. Citation1974). The samples were deprotonated by adding 10% (w/v) trichloroacetic acid solution followed by centrifugation. The ammonium was transformed by phenol and hypochlorite in an alkaline solution into a blue coloured, indole phenol-blue by the Berthelot reaction and measured spectroscopically at 623 nm.
For the determination of VFAs, 5 g of caecal samples and 5 ml 0.1 M phosphoric acid were shaken at 100 rpm before being centrifuged (7000 x g) for 10 min. Residues were collected and the supernatants again centrifuged (20.817 x g) for 10 min. Thereafter 600 μl of the supernatant was taken in a crimp vial and mixed with 600 μl of phosphoric acid containing iso-capronic acid (2.29 g/l concentration) as an internal standard. Volatile fatty acids were separated by gas chromatography using an EM-1000 (30 m × 0.53 mm) column from Alltech (Deerfield, IL, USA) with helium as the mobile phase and detection by a fluorescent infrared detector. Quantification of VFAs was based on a chemical standard solution (Merck) after internal standard correction.
Statistical analysis
The data were analysed with the use of PROC MIXED in SAS (version 9.2; SAS Inst. Inc., Cary, NC) by using the following statistical model:
where Yijk was the measured response, μ overall mean effect, Pi the ith fixed protein source effect (i = SBM, RSM or MG), and Lj the jth fixed digestible CP level effect (j = 15.8 or 17.2%). Pi × Lj was the interaction between protein source and digestible CP level, and eijk the residual error. There were no significant room effects, and so this factor was omitted from the model. Differences were considered significant at a probability level of 5% (P < 0.05).
Results
Bird performance
Overall, mortality was low (1.7%) during the study. All performance data of broilers were corrected for mortality by day. PS influenced (P < 0.001) FI, whereby broilers fed the RSM (116.5 g/d) diet had a lower FI compared with those fed the SBM diet (120.5 g/d), and FI of birds fed the MG diet (118.9 g/d) was in between (). FI of broilers fed the high DCP diet had a higher (P < 0.001) FI compared with those fed the low DCP diet (120.1 vs. 117.2 g/d). PS affected (P < 0.001) BWG, with broilers fed the RSM (69.7 g/d) had a lower BWG compared with those fed the SBM diet (77.4 g/d), while BWG of birds fed the MG diet was in between (71.9 g/d). Broilers fed the high DCP diets had a higher (P < 0.001) BWG compared with those fed the low DCP diets (75.4 vs. 70.7 g/d), regardless of the protein source. FCR was affected (P < 0.001) by PS, indicating that broilers fed the SBM diet had an improved FCR (1.51) compared with those fed the RSM (1.63) and MG (1.61) diets. Broilers fed the high DCP diet showed an improved FCR (P = 0.001) compared with those fed the low DCP diet (1.56 vs. 1.61), regardless of dietary protein source.
Table 2. Least squares means1 of performance parameters in broilers from 9 to 33 days of age as affected by protein source and digestible crude protein level.
Results of WI and water to feed (WF) ratio were affected by a PSxDCP interaction, as shown in . In low DCP diet, the RSM fed birds had a lower WI compared to the SBM and MG fed birds, whereas in high DCP diet the RSM and MG fed birds had higher WI compared to the SBM fed birds. The difference in WI between broilers fed the low and high DCP level was 16.5% for the RSM diet, whereas this difference was 12.8% and 11.8% for broilers fed the MG and SBM diets, respectively. In the low DCP diet, WF ration was not affected by PS, whereas in the high DCP diet, WF ratio was increased in birds fed the MG and RSM diet compared to birds fed the SBM diet.
Table 3. Interaction effects of protein source and digestible crude protein level (%) on water intake and water to feed ratio in broilers from 9 to 33 d of age.
Digestive tract measurements
Protein source, as well as DCP level, did not influence the relative empty weights of the gut components and there were no interactions between these treatments ().
Table 4. Effects of protein source (PS) and digestible crude protein level (DCP, %) on mean relative weights1 (g/100 g BW) of empty gastrointestinal segments in broilers.
Protein source influenced (P < 0.001) duodenal morphology. Villus height was increased by 18.2% and 17.7%, whereas crypt depth was reduced by 15.5% and 18.1%, and villus height to crypt depth ratio was increased by 29.0% and 30.9% in broilers fed the SBM compared with those fed the RSM and MG diets, respectively (). Duodenal morphology was not significantly affected by DCP level, nor by the interaction between protein source and DCP level.
Table 5. Effects of protein source (PS) and digestible crude protein level (DCP, %) on villus height (μm), crypt depth (μm) and villus height to crypt depth ratio (VCR) in the duodenum of broilers1.
Caecal digesta characteristics
Protein source influenced (P = 0.005) caecal pH (). Broilers fed the RSM diet resulted in lower pH in the caecal contents compared to birds fed the MG and SBM diets. Digestible CP level did not alter caecal pH. There were no interactions between DCP level and protein source for caecal pH. Total VFA contents and ammonia concentration in the caecal digesta were not influenced by either dietary protein source or DCP level.
Table 6. Effects of protein source (PS) and digestible crude protein (DCP, %) level on cecal digesta characteristics in broilers1.
Caecal branched chain fatty acids (BCFA) were altered by dietary protein source (P = 0.030; ) and were increased by 16.5% and 14.9% in broilers fed RSM compared with those fed SBM and MG diets, respectively. The increase in BCFA in samples from birds fed the RSM diet was caused by a significant increase in iso-butyric acid (P = 0.023) and a tendency to increased iso-valeric acid (P = 0.072). Caecal propionic acid content was reduced by 11.8% in broilers fed RSM compared with those fed MG diets, where propionic acid content of the SBM fed birds was in between (P = 0.046). Caecal valeric acid content was highest in birds fed the RSM diet (2.03 mmol/kg DM), but was reduced in birds fed the MG diet (1.74 mmol/kg DM) and further reduced in birds fed the SBM diet (1.49 mmol/kg DM) (P < 0.001).
Table 7. Effects of protein source (PS) and digestible crude protein (DCP, %) level on cecal volatile fatty acids (VFA (mmol/kg DM)) concentrations1 in broilers.
Discussion
The present study was designed to investigate the impact of three PS (SBM, RSM and MG), which are known to differ in their level of indigestible protein, on performance, gut morphology and caecal digesta characteristics in broilers. It was hypothesised that increasing the level of indigestible protein of a certain PS would result in more protein fermentation in the hindgut, which coincides with poor performance and reduced gut health. Therefore, gut morphology and fermentation characteristics were studied as explanatory variables. Additionally, it was questioned whether increasing the level of digestible CP, by either the PS or by the other digestible protein ingredients, could alleviate the negative effects caused by protein fermentation. Therefore, each PS was fed at two DCP levels.
Bird performance as related to gut health
Feed intake of MG fed birds did not differ from FI of SBM fed birds. The reduced FI for broilers fed the RSM diets was in agreement with several studies reporting adverse effects of RSM on FI if its inclusion level was more than 10% (Ahmad et al. Citation2007; Aftab Citation2009), potentially due to its poor palatability because of the presence of glucosinolates (Zeb Citation1998), and high fibre content (Naseem et al. Citation2006). RSM, additionally, contains a high amount of sulphur (1.14 vs. 0.44%) compared with SBM (Summers Citation1995), which may interact with calcium due to an alteration in anion-cation balance, resulting in poor FI (Summers and Bedford Citation1994). Increasing the DCP level for all PS diets resulted in an increase of FI by 2.4% (2.7%, 1.4% and 3.2% for RSM, MG and SBM, respectively). Ferguson et al. (Citation1998) reported an overall lower FI level in broilers fed low dietary DCP levels.
BWG was reduced in birds fed the MG or RSM supplemented diets compared to SBM fed birds, potentially due to increased hindgut fermentation for the RSM fed birds. This was indicated by lower levels of caecal pH and higher BCFA, as well as poorer gut morphology in both MG and RSM-fed birds, indicated by shorter villi and higher crypt depths. Differences in caecal pH and BCFA between MG and RSM fed birds were not expected, because the calculated amounts of indigestible protein were similar in both diets. Whether or not protein is able to enter the caeca depends on its solubility and particle size. The caecal opening is controlled by an interdigitating meshwork of villi and musculature that acts as a filter, only allowing entry of fluid and fine particles (Ferrando et al. Citation1987; Clench and Mathias Citation1995). Most likely, the RSM particles that passed through the ileum met those criteria better than the MG particles.
The reduced growth performance recorded for the RSM diets might have been related to the presence of certain toxic compounds in RSM such as glucosinolates, tannins, phytase, erucic acid and sinapine (Khajali and Slominski Citation2012). The enzymatic degradation of these glucosinolates may lead to the production of goitrin, which inhibits proper functioning of the thyroid glands and suppresses the secretion of thyroxin (Tripathi and Mishra Citation2007). This may lead to a reduced BWG in broilers fed RSM. The isothiocyanates in RSM, likewise, may result in reduced FI and, consequently, impaired growth (McNeill et al. Citation2004).
Increasing the levels of non-essential amino acids in the high DCP diets increased overall BWG by 4.7 g/d compared to the BWG of birds fed the low DCP diets, which was in line with earlier findings (Corzo et al. Citation2005). Increasing DCP in all PS diets resulted in an increase in BWG by 6.6% (8.4%, 5.1% and 6.5% for RSM, MG and SBM, respectively). The alleviating effect of high DCP on BWG in RSM-fed birds from 66.9 to 72.5 g/d was not sufficient to reach the BWG levels seen in the low DCP-SBM fed birds (75.0 g/d). This indicated that additional supplementation of digestible NEAA could not fully compensate for the negative effects of increased indigestible protein levels and/or antinutritional factors in the diet. The alleviating effect of high DCP levels on BWG in MG-fed birds (an increase from 70.1 to 73.7 g/d) seemed to be sufficient to reach the same BWG levels in the low DCP-SBM fed birds (75.0 g/d).
Low protein digestibility in a diet means less amino acids were available for growth and potentially larger amounts of indigestible CP could enter the hindgut, leading to proteolytic fermentation. The latter findings were supported by the results of De Lange et al. (Citation2003). Processing and excretion of nitrogenous compounds require more energy (Birkett and De Lange Citation2001), resulting in less energy availability for growth.
High DCP, as a treatment factor, was accompanied by high dietary CP levels. High DCP levels induced high WI as well as high WF ratios. Such findings have been previously reported by Alleman and Leclercq (Citation1997), and Ziaei et al. (Citation2008). It was reported that a 1% increase in protein content resulted in 3% more water consumption (Larbier and Leclercq Citation1992). Difference in WI and WF ratio between the three proteins sources were, however, small.
Gut morphology
Gut morphology is a marker for gut health and can be assessed by villus height and crypt depth (Awad et al. Citation2009). There is a scarcity of published data regarding the effect of CP sources and their digestible levels on these parameters in broilers. The duodenum is the major site for digestion and absorption of nutrients in the small intestine. Duodenal histology, therefore, was measured to monitor the expected negative effects of nitrogenous substances on villus height (Nousiainen Citation1991). Shorter villi indicate a decrease in surface area for absorption of nutrients from the gut, as these structures are the functional areas for nutrient absorption (Zang et al. Citation2009). In rabbits, an increase in height enhanced nutrient transport across the villus surface (Tufarelli et al. Citation2010). The shorter villi with greater crypt depth in broilers fed RSM and MG diets may be an indication of more damage to the gut by harmful compounds produced by microbial fermentation. A deeper crypt indicated increased turnover of enterocytes and, thus, more protein and energy demand for this purpose. Crypt depth is an indicator of the number of crypt cells produced (Hampson Citation1986). It has been reported that broilers spend approximately 12% of their synthesised protein on GIT turnover (Choct Citation2009). The absence of significant effects for DCP levels on villus height and crypt depth were confirmed by the findings of Buwjoom et al. (Citation2010) in broilers.
Caecal digesta characteristics
Feeding a diet with a low-digestible protein, such as one containing RSM, have approximately 7% of the seed nitrogen (N) in a tightly bound form, which will increase the amount of indigestible protein entering the caeca (Finlayson et al. Citation1973). Low ileal digestibility of protein in the RSM and MG diets compared to the SBM diet, likely resulted in more undigested protein entering the hindgut. This can stimulate protein fermentation (Hobbs et al. Citation1996), particularly if fermentable (insoluble) carbohydrates are present at low levels in the diet, as seen in the RSM diet (). Fermentable (insoluble) carbohydrates provide additional energy to gut microbes, and decrease the concentration of harmful compounds that are the result of protein fermentation (Swanson et al. Citation2002). The MG and RSM diets had similar high amounts of indigestible CP. The higher amounts of NSP in MG diets (186 and 185 g/kg, for low and high digestible CP levels, respectively) compared to RSM diets (167 and 172 g/kg), may be related to certain fermentation characteristics between MG and RSM diets. This was confirmed by the data in the current trial. For example, greater caecal BCFA and lower propionic acid concentrations in broilers fed the RSM diet compared to those fed the MG diet indicated more protein and less carbohydrate fermentation in broilers fed RSM. Propionic acid has been suggested to result from carbohydrate fermentation (Rodriguez et al. Citation2013), although propionic acid can be synthesised by bacterial fermentation of alanine and threonine (Macfarlane and Gibson Citation1995). BCFA are supposed to be a marker for protein fermentation (Macfarlane et al. Citation1992). The BCFA, i.e. isobutyric acid, 2-methylbutyric acid and isovaleric acid, are exclusively formed upon fermentation of the branched amino acids valine, isoleucine and leucine, respectively (Blachier et al. Citation2007; Gilbert et al. Citation2018). In the current study, caecal levels of isobutyric acid and total BCFA were higher in birds fed the RSM diets compared to the other diets, which indicated higher fermentation levels, especially for valine, and leucine. Moreover, caecal pH in broilers fed RSM diets was lower than in those fed the MG diets, and the highest pH was observed in broilers fed the SBM diets. Low caecal pH potentially indicated more proteolytic fermentation. RSM contains more sulphur amino acids (methionine, cysteine and taurine) compared to SBM (Okrouhlá et al. Citation2012). Fermentation of these by sulphate reducing bacteria results in the production of hydrogen sulphide (H2S) which in turn lowers the pH (Lewis and Cochrane Citation2007).
Conclusions
In conclusion, feeding diets containing RSM and MG as the major protein source compared to SBM to broilers, resulted in poorer performance, reduced villus height and deeper crypts. Increased caecal BCFA concentrations, as observed in the RSM-fed birds, were indicative of proteolytic fermentation in the hindgut, which may cause poor gut morphology and impair FCR. The results of this study showed that rapeseed meal could be used as a model for hindgut protein fermentation in broilers. To a certain extent, retarded growth performance for RSM- and MG-fed birds could be counterbalanced by increasing the dietary level of digestible CP. The compensation in growth performance, however, was limited.
Acknowledgments
The authors gratefully acknowledge the funding of the Higher Education Commission (HEC) of Pakistan for pursuing a PhD for S.N. Qaisrani in the Netherlands. Technicians of the animal facility ‘Carus’ (Wageningen University, the Netherlands) are also thanked for their assistance in performing the trial.
Disclosure statement
No potential conflict of interest was reported by the authors.
References
- Aftab, U. 2009. “Utilization of Alternative Protein Meals with or without Multiple-enzyme Supplementation in Broilers Fed Low Energy Diets.” The Journal of Applied Poultry Research 18: 292–296.
- Ahmad, G., T. Mushtaq, M. A. Mirza, and Z. Ahmed. 2007. “Comparative Bioefficacy of Lysine from L-lysine Hydrochloride or L-lysine Sulfate in Basal Diets Containing Graded Levels of Canola Meal for Female Broiler Chickens.” Poultry Science 86: 525–530.
- Alexandratos, N., and J. Bruinsma. 2012. “World Agriculture Towards 2030/2050: The 2012 Revision.” FAO, ESA working paper No. 12–03. Rome, Italy. Accessed 13 December 2016. http://www.fao.org/docrep/016/ap106e/ap106e.pdf.
- Alleman, F., and B. Leclercq. 1997. “Effect of Dietary Protein and Environmental Temperature on Growth Performance and Water Consumption of Male Broiler Chickens.” British Poultry Science 38: 607–610.
- AOAC International. 2006. Official Methods of Analysis of AOAC International. 18th ed. Gaithersburg, MD: AOAC Int.
- Apajalahti, J., and K. Vienola. 2016. “Interaction between Chicken Intestinal Microbiota and Protein Digestion.” Animal Feed Science and Technology 221: 323–330.
- Awad, W. A., K. Ghareeb, S. Abdel-Raheem, and J. Bohm. 2009. “Effects of Dietary Inclusion of Probiotic and Synbiotic on Growth Performance, Organ Weights and Intestinal Histomorphology of Broiler Chickens.” Poultry Science 88: 49–56. doi:10.3382/ps.2008-00244.
- Bikker, P., A. Dirkzwager, J. Fledderus, P. Trevisi, I. Le Huërou-Luron, J. P. Lallès, and A. Awati. 2006. “The Effect of Dietary Protein and Fermentable Carbohydrates Levels on Growth Performance and Intestinal Characteristics in Newly Weaned Piglets.” Journal of Animal Science 84: 3337–3345.
- Birkett, S., and K. De Lange. 2001. “A Computational Framework for A Nutrient Flow Representation of Energy Utilization by Growing Monogastric Animals.” British Journal of Nutrition 86: 661–674.
- Blachier, F., F. Mariotti, J. F. Huneau, and D. Tome. 2007. “Effects of Amino Acid-derived Luminal Metabolites on the Colonic Epithelium and Physiopathological Consequences.” Amino Acids 33: 547–562.
- Buwjoom, T., K. Yamauchi, T. Erikawa, and H. Goto. 2010. “Histological Intestinal Alterations in Chickens Fed Low Protein Diet.” Journal of Animal Physiology and Animal Nutrition 94: 354–361.
- Choct, M. 2009. “Managing Gut Health through Nutrition.” British Poultry Science 50: 9–15.
- Clench, M. H., and J. R. Mathias. 1995. “The Avian Cecum - A Review.” Wilson Bulletin 107: 93–121.
- Corzo, A., C. A. Fritts, M. T. Kidd, and B. J. Kerr. 2005. “Response of Broiler Chicks to Essential and Non-essential Amino Acid Supplementation of Low Crude Protein Diets.” Animal Feed Science and Technology 118: 319–327.
- CVB (Central Bureau for Livestock Feeding). 2007. Veevoedertabel, Centraal Veevoederbureau. The Netherlands: Lelystad.
- De Lange, L., C. Rombouts, and G. Oude Elferink. 2003. “Practical Application and Advantages of Using Total Digestible Amino Acids and Undigestible Crude Protein to Formulate Broiler Diets.” World’s Poultry Sciences Journal 59: 447–457.
- Ferguson, N. S., R. S. Gates, J. L. Taraba, A. H. Cantor, A. J. Pescatore, M. J. Ford, and D. J. Burnham. 1998. “The Effect of Dietary Crude Protein on Growth, Ammonia Concentration, and Litter Composition in Broilers.” Poultry Science 77: 1481–1487.
- Ferrando, C., P. Vergara, M. Jimenez, and E. Gonalons. 1987. “Study of the Rate of Passage of Food with Chromium Mordanted Plant Cells in Chickens (gallus Gallus).” Quarterly Journal of Experimental Physiology and Cognate Medical Sciences 72: 251–259.
- Finlayson, A. J., J. Krzymanski, and R. K. Downey. 1973. “Comparison of Chemical and Agronomic Characteristics of Two Brassica Napus L. Cultivars, Bronowski and Target.” Journal of the American Oil Chemists’ Society 50: 407–410.
- Gilbert, M. S., N. Ijssennagger, A. K. Kies, and S. W. C. Van Mil. 2018. “Protein Fermentation in the Gut; Implications for Intestinal Dysfunction in Humans, Pigs, and Poultry.” American Journal of Physiology-Gastrointestinal and Liver Physiology 315: G159–G170. doi:10.1152/ajpgi.00020.2018.
- Hampson, D. J. 1986. “Alterations in Piglet Small Intestinal Structure at Weaning.” Research in Veterinary Science 40: 32–40.
- Hobbs, P. J., B. F. Pain, R. M. Kay, and P. A. Lee. 1996. “Reduction of Odorous Compounds in Fresh Pig Slurry by Dietary Control of Crude Protein.” Journal of the Science of Food and Agriculture 71: 508–514.
- Khajali, F., and B. A. Slominski. 2012. “Factors that Affect the Nutritive Value of Canola Meal for Poultry.” Poultry Science 91: 2564–2575. doi:10.3382/ps.2012-02332.
- Larbier, M., and B. Leclercq. 1992. “Consommation d’aliment et d’eau.” In Nutrition et alimentation des volailles, edited by INRA, 17–25. Paris, France: INRA.
- Laudadio, V., L. Passantino, A. Perillo, G. Lopresti, A. Passantino, R. U. Khan, and V. Tufarelli. 2012. “Productive Performance and Histological Features of Intestinal Mucosa of Broiler Chickens Fed Different Dietary Protein Levels.” Poultry Science 91: 265–270. doi:10.3382/ps.2011-01675.
- Lewis, S., and S. Cochrane. 2007. “Alteration of Sulfate and Hydrogen Metabolism in the Human Colon by Changing Intestinal Transit Rate.” American Journal of Gastroenterology 102: 624–633. doi:10.1111/j.1572-0241.2006.01020.x.
- Macfarlane, G. T., and G. R. Gibson. 1995. “Microbiological Aspects of the Production of Short-chain Fatty Acids in the Large Bowel.” In Physiological and Clinical Aspects of Short-chain Fatty Acids, edited by J. H. Cummings, J. L. Rombeau, and T. Sakata, 87–105. Cambridge: Cambridge University Press.
- Macfarlane, G. T., G. R. Gibson, E. Beatty, and J. H. Cummings. 1992. “Estimation of Short-chain Fatty Acid Production from Protein by Human Intestinal Bacteria Based on Branched-chain Fatty Acid Measurements.” FEMS Microbiology Letters 101: 81–88. doi:10.1111/fml.1992.101.issue-2.
- McNeill, L., K. Bernard, and M. G. MacLeod. 2004. “Food Intake, Growth Rate, Food Conversion and Food Choice in Broilers Fed on Diets High in Rapeseed Meal and Pea Meal, with Observations on Sensory Evaluation of the Resulting Poultry Meat.” British Poultry Science 45: 519–523. doi:10.1080/00071660412331286235.
- Naseem, M., S. Khan, and M. Yousaf. 2006. “Effect of Feeding Various Levels of Canola Meal on the Performance of Broiler Chicks.” The Journal of Animal and Plant Sciences 16: 78–80.
- Nousiainen, J. 1991. “Comparative Observations on Selected Probiotics and Olaquindox as Feed Additives for Piglets around Weaning. 2. Effect on Villus Length and Crypt Depth in the Jejunum, Cecum, and Colon.” Journal of Animal Physiology and Animal Nutrition 66: 224–230. doi:10.1111/jpn.1991.66.issue-1-5.
- Novozamsky, I., R. Van Eck, J. C. H. Showenburg, and F. Walinga. 1974. “Total Nitrogen Determination in Plant Material by Means of the Indole-phenol Blue Method.” Netherlands Journal of Agricultural Science 22: 3–5.
- Okrouhlá, M., R. Stupka, J. Čitek, M. Šprysl, L. Brzobohaty, and E. Kluzakova. 2012. “The Effect of Replacing Soybean Meal with Rapeseed Meal on the Production Performance and Meat Chemical Composition in Pigs.” Research in Pig Breeding 6: 36–39.
- Owusu-Asiedu, A., S. K. Baidoo, C. M. Nyachoti, and R. R. Marquardt. 2002. “Response of Early-weaned Pigs to Spray-dried Porcine or Animal Plasma-based Diets Supplemented with Egg-yolk Antibodies against Enterotoxigenic Escherichia Coli.” Journal of Animal Science 80: 2895–2903. doi:10.2527/2002.80112895x.
- Reid, C. A., and K. Hillman. 1999. “The Effects of Retrogradation and Amylose/amylopectin Ratio of Starches on Carbohydrate Fermentation and Microbial Populations in the Porcine Colon.” Animal Science 68: 503–510. doi:10.1017/S1357729800050529.
- Rodriguez, B. A., C. C. Stowers, V. Pham, and B. M. Cox. 2013. “The Production of Propionic Acid, Propanol, Propylene via Sugar Fermentation: An Industrial Perspective on the Progress, Technical Challenges and Future Outlook.” Green Chemistry 16:: 1066–1076. doi:10.1039/C3GC42000K.
- Summers, J. D. 1995. “Canola Meal and Acid-base Balance.” Animal Feed Science and Technology 53: 109–115. doi:10.1016/0377-8401(95)02015-R.
- Summers, J. D., and M. Bedford. 1994. “Canola Meal and Diet Acid-base Balance for Broilers.” Canadian Journal of Animal Science 74: 335–339. doi:10.4141/cjas94-045.
- Swanson, K. S., C. M. Grieshop, E. A. Flickinger, L. L. Bauer, J. Chow, B. W. Wolf, K. A. Garleb, and G. C. Fahey Jr. 2002. “Fructooligosaccharides and Lactobacillus Acidophilus Modify Gut Microbial Populations, Total Tract Nutrient Digestibilities and Fecal Protein Catabolite Concentrations in Healthy Adult Dogs.” The Journal of Nutrition 132: 3721–3731. doi:10.1093/jn/132.12.3721.
- Thomke, S., and K. Elwinger. 1998. “Growth Promotants in Feeding Pigs and Poultry. III. Alternatives to Antibiotic Growth Promotants.” Animal Research 47: 245–271. doi:10.1051/animres:19980402.
- Tripathi, M. K., and A. S. Mishra. 2007. “Glucosinolates in Animal Nutrition: A Review.” Animal Feed Science and Technology 132: 1–27. doi:10.1016/j.anifeedsci.2006.03.003.
- Tufarelli, V., S. Desantis, S. Zizza, and V. Laudadio. 2010. “Performance, Gut Morphology and Carcass Characteristics of Fattening Rabbits as Affected by Particle Size of Pelleted Diets.” Archives of Animal Nutrition 64: 373–382. doi:10.1080/1745039X.2010.496945.
- Zang, J. J., X. S. Piao, D. S. Huang, J. J. Wang, X. Ma, and Y. X. Ma. 2009. “Effects of Feed Particle Size and Feed Form on Growth Performance, Nutrient Metabolizability and Intestinal Morphology in Broiler Chickens.” Asian-Australasian Journal of Animal Sciences 22: 107–112. doi:10.5713/ajas.2009.80352.
- Zeb, A. 1998. “Possibilities and Limitations of Feeding Rapeseed Meal to Broiler Chicks.” PhD Thesis, Georg-August University Gottingen, Germany.
- Ziaei, N., J. H. Guy, S. A. Edwards, P. J. Blanchard, J. Ward, and D. Feuerstein. 2008. “Effect of Reducing Dietary Mineral Content on Growth Performance, Water Intake, Excreta Dry Matter Content and Blood Parameters of Broilers.” British Poultry Science 49: 195–201. doi:10.1080/00071660801953238.