ABSTRACT
1. The objective of this study was to identify the effects of the antioxidant alpha-tocopherol acetate (ATA) and alpha lipoic acid (ALA) which have anti-inflammatory effects on developmental onset, severity and the progression of wooden breast (WB) based on Pectoralis major (P. major) muscle morphology and expression of genes associated with WB during the first three weeks post-hatch.
2. A total of 160 newly hatched Ross 708 broiler chicks were randomly assigned in a replicated trial to either a control group or three dietary treatments (ATA 160 mg/kg feed, ALA 500 mg/kg feed or in combination).
3. Microscopic changes associated with WB began at one week of age in all groups. The ATA acetate and ALA fed in combination decreased WB severity at two weeks of age (P = 0.05) and ATA alone or in combination reduced severity at three weeks of age compared to the control group (P = 0.05). Expression of myogenic determination factor 1 and peroxisome proliferator-activated receptor gamma was reduced in all dietary treatments compared to the control at three weeks of age (P ≤ 0.05), which suggested reduced muscle degeneration and lipid deposition.
4. ATA and ALA fed both independently and in combination had a positive effect on mitigating WB severity microscopically as early as two weeks of age.
Introduction
Broiler breast muscle myopathies have increased in recent years, especially in broilers with higher growth rates and breast muscle yield (Brewer et al. Citation2012; Tijare et al. Citation2016). Among the myopathies, wooden breast (WB) is of great concern as it has been reported worldwide (Sihvo et al. Citation2014; Kuttappan et al. Citation2016; Xing et al. Citation2019). Economic losses of over 200 USD million have been reported from WB condemnation of breast meat (Sihvo et al. Citation2014; Kuttappan et al. Citation2016). Wooden breast is phenotypically characterised by palpation of a rigid Pectoralis major (P. major) breast muscle (Sihvo et al. Citation2014) and histologically by myodegeneration, including myofiber necrosis (Papah et al. Citation2017), fibrosis (Sihvo et al. Citation2014; Velleman and Clark Citation2015), and immune cell infiltration (Sihvo et al. Citation2014, Citation2017). Recent studies have linked WB to oxidative stress (Mutryn et al. Citation2015; Abasht et al. Citation2016; Brothers et al. Citation2019), inflammation (Mutryn et al. Citation2015; Zambonelli et al. Citation2017), and dysregulation of lipid metabolism (Abasht et al. Citation2019; Brothers et al. Citation2019; Lake and Abasht Citation2020). Genes involved in oxidative stress and inflammation, such as selectin E (SELE) (Wang et al. Citation2020a), and lipid metabolism, such as peroxisome proliferator-activated receptor gamma (PPARγ) and CCAAT/enhancer binding protein alpha (CEBPα) (Brothers et al. Citation2019; Lake et al. Citation2019), have been shown to be differentially expressed in WB affected breast muscle compared to non-affected breast muscle.
Wooden breast has been identified in broilers as early as 18 d of age and the severity of WB increases with age and growth of the birds (Sihvo et al. Citation2017). The development of WB is closely associated with skeletal muscle growth. During the immediate post-hatch period, muscle growth is dependent on the adult myoblast satellite cells fusing with existing myofibers, which leads to muscle growth through hypertrophy (Stockdale and Holtzer Citation1961; Moss and Leblond Citation1971). The satellite cells require the expression of myogenic transcriptional regulatory factors, such as myogenic determination factor 1 (MYOD1) and myogenin (MYOG), for their proliferation and differentiation (Brunetti and Goldfine Citation1990; Yablonka-Reuveni et al. Citation1999). One of the transmembrane extracellular matrix heparan sulphate proteoglycans, syndecan-4 (SDC4), plays an essential role in muscle growth and development through regulating satellite cell proliferation, migration and differentiation (Velleman et al. Citation2007; Shin et al. Citation2013). Satellite cell mitotic activity is highest in the first week after hatch (Halevy et al. Citation2000) and can be affected by nutrition (Halevy et al. Citation2000; Velleman et al. Citation2010; Powell et al. Citation2014). Hence, nutritional strategies during the immediate post-hatch period can be used to influence breast muscle development and growth and reduce the incidence of WB.
Alpha-tocopherol acetate (ATA) and alpha lipoic acid (ALA) are two nutrients that have important antioxidant capacities (Rymer and Givens Citation2010; Cheng et al. Citation2016; El-Senousey et al. Citation2018). They can terminate lipid peroxidation by reacting with free radicals, which mitigates cell membrane oxidation (Niki et al. Citation1993). The nutrient requirement of vitamin E is 10 mg/kg feed for broilers according to National Research Council (NRC Citation1994). However, this may not meet the current requirement for commercial broilers. Higher levels of vitamin E are required for a higher level of oxidative stress in current rapid-growth broilers. Gaal et al. (Citation2000) found that supplementation of 90 mg/kg vitamin E increased tissue oxidative stability. Other research has shown that 250 mg/kg vitamin E improved antioxidant status (Harsini et al. Citation2012) and immune responses in broilers (Rehman et al. Citation2017).
Alpha-tocopherol acetate is one of the eight forms of vitamin E commonly used in the poultry industry with efficiency in preventing tissue oxidative damage (Hosomi et al. Citation1997; Panda and Cherian Citation2014; Niki Citation2016). Previous studies have shown that ATA supplementation (180 mg/kg) early after hatch reduced WB severity in broilers at 58 d of age both phenotypically (Wang et al. Citation2020b) and microscopically (Wang et al. Citation2020a). Additionally, ALA is a short chain fatty acid with anti-inflammatory properties achieved by inhibiting the release of pro-inflammatory cytokines, such as tumour necrosis factor alpha (Li et al. Citation2014). Both its antioxidant and anti-inflammatory properties make ALA a good candidate to reduce the WB severity. El-Senousey et al. (Citation2013) showed that the optimal concentration of ALA was between 400 and 800 mg/kg when supplemented in broiler diets from 22 to 42 d of age, resulting in an improvement in meat quality. Combining ALA and ATA has been shown to function synergistically through the enhancement of antioxidant activity (Gonzalez-Perez and Gonzalez-Castaneda Citation2006). After being reduced into dihydrolipoic acid, ALA helps recycle vitamin E from its oxidised form (Sohaib et al. Citation2018). Therefore, ATA and ALA, both independently and in combination, are highly likely to influence the onset and development of WB myopathy through anti-oxidative and anti-inflammatory effects during the early growth period. Although trials (Wang et al. Citation2020b, Citation2020a) have shown that ATA reduced the severity of WB at 58 d of age in broilers, there is no information about how ATA and ALA combined may influence its onset and development and at what age the beneficial changes can be initiated. The objective of the current study was to evaluate the effects of ATA, ALA alone and in combination on the onset, severity and progression of WB myopathy during the early growing period. The effects were determined based on developmental changes in P. major muscle morphological structure and the expression of genes related with muscle formation and growth, adipogenesis, extracellular matrix, oxidative stress and inflammation from one to three weeks of age in commercial broilers.
Materials and methods
The birds used in this study were the same birds that were used in an intestinal development study (Wang et al. Citation2021). Hence, materials and methods specific to the birds and diets are the same. All bird protocols were approved by the Institutional Animal Care and Use Committee of Ohio State University.
Birds and experimental diets
A total of 160 newly hatched commercial Ross 708 male broiler chicks were individually weighed, wing banded and placed into pens immediately after hatching. Chicks were randomly divided into four groups, including a control group (corn-soybean meal basal diet), ATA (160 mg/kg) supplemented group, ALA (500 mg/kg) supplemented group, and a combination of ATA (160 mg/kg) and ALA (500 mg/kg). There were 10 pens per treatment and each pen contained four birds. Broilers had ad libitum access to feed and water. Diets were formulated to meet or exceed all NRC nutritional requirements and recommendations from the Ross broiler production handbook (Aviagen Citation2016). Feed ingredients and calculated nutrient composition in the starter phase and grower phase are shown in , respectively.
Table 1. Feed ingredients and calculated nutritional composition of starter diets1.
Table 2. Feed ingredients and calculated nutritional composition of grower diets1.
Growth performance
The body weight of each bird was measured weekly throughout the trial and average weekly gain (AWG) was calculated. Feed intake (FI) was determined weekly and feed conversion ratio (FCR) was calculated as the ratio of feed intake and body weight. Only one chick in the control group died at the beginning of the study and was not used for any performance calculations. At one, two and three weeks of age, one chick from each pen was randomly selected and the final body weight was taken prior to death by exsanguination. The left P. major muscle was weighed and the right P. major muscle was used for morphological analysis and gene expression analysis. The P. major weight was calculated as twice the weight of the left muscle.
Phenotypic wooden breast score
Phenotypic WB score was determined by palpation of the left P. major muscle. The evaluation was based on firmness using a zero to three scale, as described by Tijare et al. (Citation2016). A score of zero represented no WB with the fillets being flexible in the entire muscle. A score of one indicated mild WB with fillets firmness mainly in the anterior region. A score of two indicated moderate WB and the fillets were firm along the entire muscle, except from the mid to caudal region. A score of three represented severe WB where fillets were extremely firm throughout the entire P. major muscle.
Pectoralis major muscle morphology
The right P. major muscle was collected according to the method of Velleman et al. (Citation2003). Muscle fibres in the anterior portion of the muscle were dissected following their orientation and tied to wooden applicator sticks to prevent contraction, fixed in 10% (vol/vol) buffered formalin (pH 7.0), and stored at 4°C. Histological samples were dehydrated in a graded series of alcohols, cleared in Pro Par Clearant (Anatech, Battle Creek, MI) and embedded in paraffin according to the procedure of Jarrold et al. (Citation1999). Paraffin blocks were cross sectioned at 5 µm, mounted on Starfrost Adhesive slides (Mercedes Medical, Sarasota, FL), and haematoxylin and eosin stained as described by Jarrold et al. (Citation1999). Each slide contained a minimum of four sections.
Digital photomicrographs were taken with an Olympus IX70 fluorescent microscope (Olympus America, Mellville, NY) and QImaging digital camera (QImaging, Burnaby, BC, Canada) equipped with CellSens Imaging software (Olympus America). Four photomicrographs from each section were taken at 100× and 200× magnification for P. major muscle morphology score and microscopic WB score evaluation. The morphology score was evaluated as described by Velleman et al. (Citation2003). Samples were scored independently using a one to five scale by three trained panelists. A score of one was given to samples with limited or no perimysial or endomysial connective tissue spacing, and excessive myofiber degradation. A score of five was given to samples with well-structured muscle fibre bundles and myofibers with ample perimysial and endomysial connective tissue spacing. Scores of two to four were intermediate. Microscopic WB score was identified based on the degree of fibrosis, necrosis and immune cell infiltration, with a score of zero representing no necrosis, fibrosis or immune cell infiltration, one representing minimal necrosis, fibrosis, and immune cell infiltration, two being intermediate, and three representing severe necrosis, fibrosis and immune cell infiltration.
Myofiber width, perimysial and endomysial width were measured from four photomicrographs per bird. At least 20 measurements per characteristic were taken in each photomicrograph at 200× magnification using Image Pro software (Media Cybernectics, Bethesda, MD).
RNA extraction and real-time quantitative PCR
A ~ 0.5 g sample from the right P. major muscle was placed in RNAlater (Ambion, Grand Island, NY) for gene expression analysis from each bird after euthanasia. After 24 h, RNAlater was removed and samples were maintained at −20°C until RNA extraction. Total RNA was extracted from muscle samples using RNAzol RT (Molecular Research Center, Madison, MI) according to manufacturer’s protocol. Concentration of the total RNA was measured with a Nanodrop spectrophotometer ND-1000 (Thermo Fisher Scientific, Waltham, MA). The cDNA was synthesised with M-MLV reverse transcriptase (Promega, Madison, WI) and real-time quantitative PCR (qPCR) was performed with the DyNAmo Hot Start SYBR Green qPCR kit (ThermoFisher, Waltham, MA) as described in Velleman et al. (Citation2014). The reaction consisted of 1 µl of cDNA, 5 µl of DyNAmo Hot Start SYBR Green qPCR master mix, 0.5 µl of primer mixture (10 µM), and 3.5 µl of RNase-DNase free water. Genes, whose expression is associated with muscle formation and growth, adipogenesis, extracellular matrix, oxidative stress and inflammation, were selected as target sequences. Primer sequences of these and the housekeeping gene, glyceraldehyde-3-phosphate dehydrogenase (GAPDH), are listed in . The qPCR was performed with appropriate cycling conditions as follows: denaturation (94°C for 15 min), amplification and quantification (35 cycles of 94°C for 30 s, 53°C for SELE; 55°C for PPARγ, CEBPα, and GAPDH; 58°C for MYOD1 and MYOG; and 60°C for SDC4 for 30 s, and 72°C for 30 s), and final extension (72°C for 5 min). The PCR products were analysed on a 1.0% agarose gel to confirm amplification specificity. Gene expression was calculated as arbitrary units using the standard curve method, which was constructed for each target gene and housekeeping gene using serial dilutions of purified PCR products. Target gene expression was then normalised using GAPDH expression with each cDNA product concentration being divided by GAPDH concentration.
Table 3. Primer sequences for real-time quantitative PCR
Statistical analysis
Growth performance, P. major muscle morphology, and gene expression were analysed as a completely randomised design using the PROC MIXED procedure of SAS version 9.4 software (SAS Institute INC., Cary, NC). Individual pen was identified as the experimental unit. Data were collected from 10 pens per treatment. Dietary treatments were used as a fixed effect. Each pen was considered as a random effect. Least square means were estimated with the LSMEANS procedure and separated with the PDIFF option and a Tukey adjustment. Significance was accepted at P ≤ 0.05. Categorical data for WB score were analysed using the PROC FREQ procedure of SAS. Significance was established at P ≤ 0.05. In addition, the effects of ATA, ALA, or in combination were determined by setting up orthogonal contrasts using data from each treatment to test the responses of the treatment against the control group, respectively, for all data.
Results
Growth performance
Broiler growth performance is shown in . Broilers fed different dietary treatments did not show any significant differences in AWG, FCR, final body weight, or P. major weight at one, two or three weeks of age (P > 0.05). However, broilers supplemented with ATA and ALA independently had decreased FI at three weeks of age compared to the control group and the combination of ATA and ALA group (P < 0.01).
Table 4. Effect of alpha-tocopherol acetate and alpha lipoic acid on broiler growth performance from 1 to 3 weeks post-hatch
Pectoralis major muscle morphology
No phenotypic WB was identified by palpation from one to three weeks of age. However, mild WB with microscopic WB score of one was detected beginning at one week of age in all groups (), whereby 50% of the P. major muscles had mild microscopic WB and 50% had no WB in the control and ALA group, respectively. Broiler chicks in the ATA and combination groups had 40% mild WB and 60% no WB at one week of age. At two weeks of age, the combination treatment group had less WB severity compared to the control group (P = 0.05). At this age in the control group, 60% of the P. major muscle samples exhibited a mild level of WB and 20% were moderate. In contrast, no moderate WB cases were found in ATA, ALA or in combination at two weeks of age, although 50% had mild WB and 50% had no WB. The combined treatment group had 70% no WB and 30% mild WB at this age.
Table 5. Effect of alpha-tocopherol acetate and alpha lipoic acid on microscopic wooden breast (WB) score of broiler Pectoralis major muscle
At three weeks of age, wooden breast severity was increased in the control group whereby 10% had none, 80% mild and 10% moderate WB. The ATA and combination groups had 60% of samples showing no WB and 40% with mild WB. The ALA group had 50% of samples with no WB and 50% mild WB. Hence, WB severity was decreased in the ATA and combination groups compared to the control group (P = 0.05). Representative photomicrographs showing the morphological structure of the P. major muscles are shown in . Fibrotic changes, with fibrillar collagen replacing the myofibers, were observed in the WB affected P. major muscle in all groups () and hypertrophic myofibers and fat cells were found in the WB affected tissues. In contrast, the WB unaffected P. major muscles did not have these microscopic changes usually associated with fibrosis ().
Figure 1. Representative photomicrographs of wooden breast affected (a,c,e,g) or not affected (b,d,f,h) Pectoralis major muscle samples in the four treatments at 3 weeks of age. Broilers in the control group (a,b) were fed corn-soybean meal basal diet. Alpha-tocopherol acetate (ATA; 160 mg/kg) was supplemented in the ATA group (c,d) and alpha lipoic acid (ALA; 500 mg/kg) was supplemented in the ALA group (e,f). Combination of ATA and ALA was supplemented in the ATA and ALA group (g,h). C = Collagen; F = Fat cell; H = Hypercontracted myofiber; P = Perimysial connective tissue; and E = Endomysial connective tissue. Scale bar = 100 μm
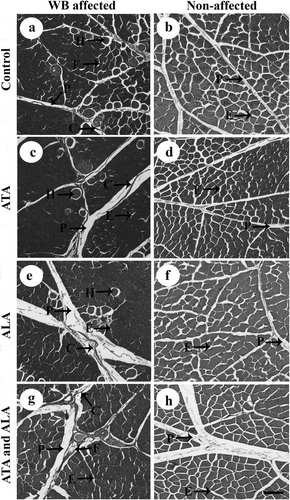
Interestingly, there was no significant difference in fibre width, perimysial and endomysial connective tissue spacing, or morphology score among the dietary treatments within each age (P > 0.05; ).
Table 6. Effect of alpha-tocopherol acetate and alpha lipoic acid on fibre width, perimysial and endomysial connective tissue spacing, and morphology score of broiler Pectoralis major muscle
Pectoralis major muscle gene expression
In terms of genes associated with muscle formation and growth (), MYOD1 expression was reduced by 6.6%, 9.0%, and 13.6% at three weeks of age for broilers fed diets supplemented with ATA and ALA independently or in combination compared to the control group, respectively (P < 0.01). Broilers fed dietary ALA and the combination treatment had 37.9% and 65.1% decrease in MYOG expression at three weeks of age compared to the control group, respectively (P < 0.01). With regard to genes associated with adipogenesis, supplementation of ATA and ALA independently or in combination resulted in 62.1%, 30.6%, and 51.6% reduction in PPARγ expression at two weeks of age, and 50.0%, 285.7%, and 107.7% reduction at three weeks of age compared to the control group, respectively (P < 0.01). The single ATA and ALA supplementation reduced CEBPα expression by 11.1% and 7.1% at two weeks of age compared to the control group, respectively (P < 0.01). Additionally, ALA and the combination treatment group decreased CEBPα expression by 30.0% at three weeks of age compared to the control group (P < 0.01). Dietary ALA resulted in a 13.0% and 20.0% reduction in SELE, a gene related with oxidative stress and inflammation, at two (P = 0.02) and three weeks of age (P < 0.01) compared to the control group, respectively. In terms of gene related with extracellular matrix, there was no significant difference in SDC4 expression among the dietary treatments within each age (P > 0.05).
Table 7. Effect of alpha-tocopherol acetate and alpha lipoic acid on gene expression in broiler Pectoralis major muscle
Discussion
Prior morphological and genomic analyses have shown that severe oxidative stress (Mutryn et al. Citation2015; Abasht et al. Citation2016) and inflammation (Mudalal et al. Citation2015; Sihvo et al. Citation2017) are present in WB affected breast muscle. Oxidative stress and inflammation are thought to be caused by rapid post-hatch growth (Kong et al. Citation2017). Since muscle growth is dependent on satellite cells which are most active the first week after hatch (Mozdziak et al. Citation2002) and are sensitive to nutritional changes (Halevy et al. Citation2000; Velleman et al. Citation2010, Citation2014; Powell et al. Citation2014), strategies to reduce oxidative stress and inflammation during the early growth period can be used to influence muscle growth and reduce the severity and incidence of myopathies like WB. Previous studies have identified that supplementation with the antioxidant ATA during the starter (0 to 10 d) or grower phase (11 to 24 d), reduced the severity of WB (Wang et al. Citation2020b) and improved P. major muscle morphological structure (Wang et al. Citation2020a) at 58 d of age in broilers. The ALA augments immunity through anti-inflammatory activities and improves antioxidant defence system (Ma et al. Citation2015). Combination of ATA and ALA can function synergistically and will further increase antioxidant activity (Gonzalez-Perez and Gonzalez-Castaneda Citation2006). Therefore, the present study investigated the effects of ATA and ALA individually and in combination on the onset, severity, and progression of WB based on developmental changes in P. major muscle morphological structure and expression of genes associated with WB during the first three weeks after hatching.
In general, dietary ATA and ALA did not have an impact on growth performance, except for FI. Supplementation of ATA and ALA independently decreased FI at three weeks of age, which was consistent with previous studies showing that ATA (Konieczka et al. Citation2018) and ALA incorporation (Zhang et al. Citation2014) reduced growth performance. El-Senousey et al. (Citation2013) reported that 800 and 1200 mg/kg ALA in feed resulted in decreased FI, while 400 mg/kg ALA did not influence FI or FCR. Hence, different concentrations of ALA have varied effects on FI, with higher levels of ALA having potential negative effects. However, combining ATA and ALA ameliorated any negative influence on growth performance because of their synergistic effect on alleviating oxidative stress (Srilatha et al. Citation2010; Parveen et al. Citation2013), which was confirmed in the present study. Sohaib et al. (Citation2012) suggested that dietary supplementation of ATA along with ALA improved antioxidant potential and lipid stability of broiler breast meat.
Wooden breast has been detected as early as 18 d of age by palpation in Ross 508 broiler chicks (Sihvo et al. Citation2017) and by Griffin et al. (Citation2018) at 23 days in Ross 708 broiler chicks. In the current study with Ross 708 broiler chicks, there was no phenotypic detection of WB at three weeks of age using palpation. These differences in phenotypic identification by palpation could be related to the differences in organisation of the collagen between distinct genetic broiler lines (Velleman et al. Citation2017; Tonniges et al. Citation2019). Velleman et al. (Citation2017) reported that in a fast-growing line with a high frequency of WB detection, tightly packed crosslinked collagen fibrils were identified. In another fast-growing line with a low frequency of WB phenotypic detection, collagen fibrils were randomly aligned with a low level of crosslinking. In another fast-growing line with no detectable WB, collagen fibres were sparsely distributed. The tightly packed collagen associated with a high level of crosslinking has been attributed to hardness of P. major muscle. In contrast, diffuse collagen with a low level of crosslinking does not impact the toughness of the breast muscle. The non-reversible trivalent hydroxylysylpyridinium (HP) crosslinks increase with age (Palokangas et al. Citation1992; Haus et al. Citation2007) and such higher amounts result in less tender meat (McCormick Citation1999; Purslow Citation2018).
Microscopically, mild WB was observed in the first week of age in all groups, but supplemental ATA and ALA altered the onset and development of microscopic WB in the present study. The control group had increasingly severe WB, as identified microscopically, from one to three weeks of age, while the ATA group and combination of ATA and ALA group did not show any increase in WB incidence during this period. Combined supplementation decreased WB severity at two weeks of age and ATA alone or in combination reduced WB severity at three weeks of age compared to the control group. This was consistent with previous studies where supplementation of ATA (180 mg/kg) reduced WB severity when assessed by palpation (Wang et al. Citation2020b) and by microscopic evaluation (Wang et al. Citation2020a) in broilers at 58 d. Taken together, these data demonstrated that appropriate dietary supplementation in the early growth period can positively affect long-term breast muscle development.
In terms of P. major muscle morphology, fibre width, perimysial and endomysial connective tissue spacing were not influenced by dietary ATA and ALA supplementation both independently and in combination. Morphology score, which was evaluated based on perimysial and endomysial connective tissue spacing and myofiber structure, was not impacted. Similar findings were observed by Wang et al. (Citation2020a) in broilers at 58 d.
In contrast, gene expression in P. major muscle was significantly influenced by dietary supplementation. Genes associated with muscle proliferation and differentiation, MYOD1 and MYOG, were differentially expressed in the dietary treatments at three weeks of age. Muscle growth after hatch is dependent on satellite cells (Moss and Leblond Citation1971), which are most active in the first week, and then gradually become quiescent (Halevy et al. Citation2000). However, satellite cells can be reactivated to undergo regeneration when the muscle is damaged (Schultz Citation1989). They proliferate to increase satellite cell number and differentiate by fusing with existing myofibers (Moss and Leblond Citation1971). The MYOD1 and myogenic factor 5 transcriptional regulatory factors are necessary for proliferation (Rudnicki et al. Citation1993) while MYOG is required for differentiation leading to the formation of multinucleated myotubes (Hasty et al. Citation1993). The reduction in MYOD1 expression in ATA and ALA groups both independently and in combination, and reduced MYOG expression in the ALA and combination fed groups compared to the control group at three weeks of age suggested decreased proliferation and differentiation. These data suggested that fewer muscle fibres were damaged and entered the regeneration process. The higher MYOD1 and MYOG expression along with the higher microscopic WB severity in the control group compared to the other dietary treatments at three weeks of age were in agreement with Velleman and Clark (Citation2015) whereby expression of genes related with myogenic proliferation and differentiation were increased in the WB affected breast muscle.
Expression of SELE, which is related with oxidative stress and inflammation, was decreased at two and three weeks of age when the diets were supplemented with ALA compared to the control group. As an important adhesion molecule, SELE plays a key role in chronic and acute inflammation (Lundberg Citation2000; Ley Citation2003) and helps mediate leukocyte migration to inflammatory sites through leukocyte-endothelial interactions (Fries et al. Citation1993; De Luca et al. Citation1994). When there are oxidative signals involved in endothelium or intracellular oxidative damage, extracellular inducers, such as cytokines, are recruited, and nuclear factor-κB is further activated initiating a cascade of kinases and regulating SELE expression (Collins Citation1993; Collins et al. Citation1995; Ghosh et al. Citation1998). In the current study, ALA supplementation reduced SELE expression at two and three weeks of age, which explained the reduced severity of WB in these broiler chicks and suggested a lower level of oxidative stress and inflammation in the breast muscle.
Recent studies have shown that dysregulation of lipid deposition is closely associated with WB (Abasht et al. Citation2016; Zambonelli et al. Citation2017; Papah et al. Citation2017, Citation2018; Lake et al. Citation2019). Thus, genes involved in lipid metabolism, such as PPARγ and CEBPα, are important markers for WB development. Both PPARγ and CEBPα are adipogenic genes mediating differentiation of muscle cells into adipocytes (Hu et al. Citation1995) and fatty acids metabolism (Kliewer et al. Citation1997; Rosen et al. Citation1999). The PPARγ gene is expressed early in adipogenesis, leading to up-regulation in the expression of CEBPα (Tontonoz et al. Citation1994; Yeh et al. Citation1995). Higher expression of PPARγ and CEBPα is commonly related to increased intramuscular lipid deposition (Liu et al. Citation2017; Cui et al. Citation2018). In the present study, broilers supplemented with ATA and ALA individually or in combination had decreased PPARγ and CEBPα expression, which suggested reduced fat deposition and WB severity.
In terms of genes related with extracellular matrix, there was no significant effect on SDC4 expression among the treatments within each age. A transmembrane heparan sulphate proteoglycan, SDC4, is associated with focal adhesion formation and cell migration (Longley et al. Citation1999; Couchman Citation2003). The SDC4 activates protein kinase C alpha through the its cytoplasmic domain (Woods and Couchman Citation1992; Lee et al. Citation1998; Lim et al. Citation2003; Shin et al. Citation2013) and then activates Ras homolog family member A (RhoA) to modulate focal adhesion, cell migration, and proliferation (Song et al. Citation2012; Woods et al. Citation2000; Dovas et al. Citation2006). No effect in SDC4 expression indicated that there was no effect of the dietary supplementation on SDC4 regulation of RhoA mediated cell migration.
In conclusion, administration of ATA and ALA independently and in combination during the first three weeks post hatch may mitigate the onset and development of WB. Genes involved in muscle growth and development, adipogenesis, oxidative stress and inflammation were differentially expression in broiler chicks supplemented with ATA and ALA independently and in combination at two and three weeks of age, which indicated reduced P. major muscle degeneration and less dysregulated lipid deposition. These findings showed that ATA and ALA may be used to reduce the incidence of WB in early growth, which may be used to reduce WB incidence and severity in broilers grown to market age. Future study should determine the effects of ATA and ALA on the developmental onset, severity, and progression of WB of the broilers to an older age.
Acknowledgments
This study was supported by US Poultry and Egg grant (No. 710) to SGV and SKJ and the China Scholarship Council (No. 201706350026) to JW. The authors would like to thank Janet McCormick for technical assistance.
Disclosure Statement
No potential conflict of interest was reported by the author(s).
Additional information
Funding
References
- Abasht, B., M. F. Mutryn, R. D. Michalek, and W. R. Lee. 2016. “Oxidative Stress and Metabolic Perturbations in Wooden Breast Disorder in Chickens.” PLoS ONE 11 (4): 1–16. https://doi.org/10.1371/journal.pone.0153750.
- Abasht, B., N. Zhou, W. R. Lee, Z. Zhuo, and E. Peripolli. 2019. “The Metabolic Characteristics of Susceptibility to Wooden Breast Disease in Chickens with High Feed Efficiency.” Poultry Science 98 (8): 3246–3256. https://doi.org/10.3382/ps/pez183.
- Aviagen. 2016. Ross Broiler Management Manual. Huntsville, AL: Aviagen.
- Brewer, V. B., V. A. Kuttappan, J. L. Emmert, J. F. C. Meullenet, and C. M. Owens. 2012. “Small Bird Programs: Effect of Strain, Sex, and Debone Time on Meat Quality of Broilers.” Poultry Science 91 (2): 248–254. https://doi.org/10.3382/ps.2011-01706.
- Brothers, B., Z. Zhuo, M. B. Papah, and B. Abasht. 2019. “Rna-seq Analysis Reveals Spatial and Sex Differences in Pectoralis Major Muscle of Broiler Chickens Contributing to Difference in Susceptibility to Wooden Breast Disease.” Frontiers in Physiology 10: 764. https://doi.org/10.3389/fphys.2019.00764.
- Brunetti, A., and I. D. Goldfine. 1990. “Role of Myogenin in Myoblast Differentiation and Its Regulation by Fibroblast Growth Factor.” The Journal of Biological Chemistry 265 (11): 5960–5963. https://doi.org/10.1016/S0021-9258(19)39275-0.
- Cheng, K., Y. Niu, X. C. Zheng, H. Zhang, Y. P. Chen, M. Zhang, X. X. Huang, L. L. Zhang, Y. M. Zhou, and T. Wang. 2016. “A Comparison of Natural (D-α-Tocopherol) and Synthetic (DL-α-Tocopherol Acetate) Vitamin E Supplementation on the Growth Performance, Meat Quality and Oxidative Status of Broilers.” Asian-Australasian Journal of Animal Sciences 29 (5): 681–688. https://doi.org/10.5713/ajas.15.0819.
- Collins, T. 1993. “Endothelial Nuclear Factor-Kappa B and the Initiation of the Atherosclerotic Lesion.” Laboratory Investigation; a Journal of Technical Methods and Pathology 68 (5): 499–508.
- Collins, T., M. A. Read, A. S. Neish, M. Z. Whitley, D. Thanos, and T. Maniatis. 1995. “Transcriptional Regulation of Endothelial Cell Adhesion Molecules: NF‐κB and Cytokine‐inducible Enhancers.” The FASEB Journal 9 (10): 899–909. https://doi.org/10.1096/fasebj.9.10.7542214.
- Couchman, J. R. 2003. “Syndecans: Proteoglycan Regulators of Cell-Surface Microdomains?.” Nature Reviews Molecular Cell Biology 4 (12): 926–937. https://doi.org/10.1038/nrm1257.
- Cui, H., M. Zheng, G. Zhao, R. Liu, and J. Wen. 2018. “Identification of Differentially Expressed Genes and Pathways for Intramuscular Fat Metabolism between Breast and Thigh Tissues of Chickens.” BMC Genomics 19 (1): 55. https://doi.org/10.1186/s12864-017-4292-3.
- De Luca, D. R., M. Z. Johnson, T. C. Whitleyflll, and J. S. Pober. 1994. “Camp and Tumor Necrosis Factor Competitively Regulate Transcriptional Activation through and Nuclear Factor Binding to the cAMP-Responsive Element/Activating Trancscription Factor Element of the Endothelial Leukocyte Adhesion Molecule-1 (E-selectin) Promo.” The Journal of Biological Chemistry 269 (30): 19193–19196.
- Dovas, A., A. Yoneda, and J. R. Couchman. 2006. “PKC-α-Dependent Activation of RhoA by Syndecan-4 during Focal Adhension Formation.” Journal of Cell Science 119 (13): 2837–2846. https://doi.org/10.1242/jcs.03020.
- El-Senousey, H. K., B. Chen, J. Y. Wang, A. M. Atta, F. R. Mohamed, and Q. H. Nie. 2018. “Effects of Dietary Vitamin C, Vitamin E, and Alpha-Lipoic Acid Supplementation on the Antioxidant Defense System and Immune-Related Gene Expression in Broilers Exposed to Oxidative Stress by Dexamethasone.” Poultry Science 97 (1): 30–38. https://doi.org/10.3382/ps/pex298.
- El-Senousey, H. K., A. M. Fouad, J. H. Yao, Z. G. Zhang, and Q. W. Shen. 2013. “Dietary Alpha Lipoic Acid Improves Body Composition, Meat Quality and Decreases Collagen Content in Muscle of Broiler Chickens.” Asian-Australasian Journal of Animal Sciences 26 (3): 394–400. https://doi.org/10.5713/ajas.2012.12430.
- Fries, J. W. U., A. J. Williams, R. C. Atkins, W. Newman, M. F. Lipscomb, and T. Collins. 1993. “Expression of VCAM-1 and E-Selectin in an in Vivo Model of Endothelial Activation.” American Journal of Pathology 143 (3): 725–737.
- Gaal, T., L. Wagner, F. Husveth, H. A. Manilla, P. Vajdovich, N. Balogh, I. Loth, and S. K. Nemeth. 2000. “Effects of Saturated and Unsaturated Fats with Vitamin E Supplementation on the Antioxidant Status of Broiler Chicken Tissues.” Acta Veterinaria Hungarica 48 (1): 69–79. https://doi.org/10.1556/avet.48.2000.1.8.
- Ghosh, S., M. J. May, and E. B. Kopp. 1998. “NF-ΚB and REL Proteins: Evolutionarily Conserved Mediators of Immune Responses.” Annual Review of Immunology 16 (1): 225–260. https://doi.org/10.1146/annurev.immunol.16.1.225.
- Gonzalez-Perez, O., and R. E. Gonzalez-Castaneda. 2006. “Therapeutic Perspectives on the Combination of α-Lipoic Acid and Vitamin E.” Nutrition Research 26 (1): 1–5. https://doi.org/10.1016/j.nutres.2005.11.011.
- Griffin, J. R., L. Moraes, M. Wick, and M. S. Lilburn. 2018. “Onset of White Striping and Progression into Wooden Breast as Defined by Myopathic Changes Underlying Pectoralis Major Growth. Estimation of Growth Parameters as Predictors for Stage of Myopathy Progression.” Avian Pathology 47 (1): 2–13. https://doi.org/10.1080/03079457.2017.1356908.
- Halevy, O., A. Geyra, M. Barak, Z. Uni, and D. Sklan. 2000. “Early Posthatch Starvation Decreases Satellite Cell Proliferation and Skeletal Muscle Growth in Chicks.” Journal of Nutrition 130 (4): 858–864. https://doi.org/10.1093/jn/130.4.858.
- Harsini, S. G., M. Habibiyan, M. M. Moeini, and A. R. Abdolmohammadi. 2012. “Effects of Dietary Selenium, Vitamin E, and Their Combination on Growth, Serum Metabolites, and Antioxidant Defense System in Skeletal Muscle of Broilers under Heat Stress.” Biological Trace Element Research 148 (3): 322–330. https://doi.org/10.1007/s12011-012-9374-0.
- Hasty, P., A. Bradley, J. H. Morris, D. G. Edmondson, J. M. Venutit, E. N. Olson, and W. H. Kleln. 1993. “Muscle Deficiency and Neonatal Death in Mice with a Targeted Mutation in the Myogenin Gene.” Nature 364: 501–506. https://doi.org/10.1038/364501a0.
- Haus, J. M., J. A. Carrithers, S. W. Trappe, and T. A. Trappe. 2007. “Collagen, Cross-Linking, and Advanced Glycation End Products in Aging Human Skeletal Muscle.” Journal of Applied Physiology 103 (6): 2068–2076. https://doi.org/10.1152/japplphysiol.00670.2007.
- Hosomi, A., M. Arita, Y. Sato, C. Kiyose, T. Ueda, O. Igarashi, H. Arai, and K. Inoue. 1997. “Affinity for α-Tocopherol Transfer Protein as a Determinant of the Biological Activities of Vitamin E Analogs.” FEBS Letters 409 (1): 105–108. https://doi.org/10.1016/S0014-5793(97)00499-7.
- Hu, E., P. Tontonoz, and B. M. Spiegelman. 1995. “Transdifferentiation of Myoblasts by the Adipogenic Transcription Factors PPARγ and C/EBPα.” Proceedings of the National Academy of Sciences of the United States of America 92 (21): 9856–9860. https://doi.org/10.1073/pnas.92.21.9856.
- Jarrold, B. B., W. L. Bacon, and S. G. Velleman. 1999. “Expression and Localization of the Proteoglycan Decorin during the Progression of Cholesterol Induced Atherosclerosis in Japanese Quail: Implications for Interaction with Collagen Type I and Lipoproteins.” Atherosclerosis 146 (2): 299–308. https://doi.org/10.1016/S0021-9150(99)00154-9.
- Kliewer, S. A., S. S. Sundseth, S. A. Jones, P. J. Brown, G. B. Wisely, C. S. Koble, P. Devchand, et al. 1997. “Fatty Acids and Eicosanoids Regulate Gene Expression through Direct Interactions with Peroxisome Proliferator-Activated Receptors α and γ.” Proceedings of the National Academy of Sciences of the United States of America 94 (9): 4318–4323. https://doi.org/10.1073/pnas.94.9.4318.
- Kong, B. W., N. Hudson, D. Seo, S. Lee, B. Khatri, K. Lassiter, D. Cook, et al. 2017. “RNA Sequencing for Global Gene Expression Associated with Muscle Growth in a Single Male Modern Broiler Line Compared to a Foundational Barred Plymouth Rock Chicken Line.” BMC Genomics 18 (1): 1–19. https://doi.org/10.1186/s12864-016-3471-y.
- Konieczka, P., M. Barszcz, M. Choct, and S. Smulikowska. 2018. “The Interactive Effect of Dietary N-6: N-3 Fatty Acid Ratio and Vitamin E Level on Tissue Lipid Peroxidation, DNA Damage in Intestinal Epithelial Cells, and Gut Morphology in Chickens of Different Ages.” Poultry Science 97 (1): 149–158. https://doi.org/10.3382/ps/pex274.
- Kuttappan, V. A., B. M. Hargis, and C. M. Owens. 2016. “White Striping and Woody Breast Myopathies in Modern Poultry Industry: A Review.” Poultry Science 95 (11): 2724–2733. https://doi.org/10.3382/ps/pew216.
- Lake, J. A., and B. Abasht. 2020. “Glucolipotoxicity : A Proposed Etiology for Wooden Breast and Related Myopathies in Commercial Broiler Chickens.” Frontiers in Physiology 11: 169. https://doi.org/10.3389/fphys.2020.00169.
- Lake, J. A., M. B. Papah, and B. Abasht. 2019. “Increased Expression of Lipid Metabolism Genes in Early Stages of Wooden Breast Links Myopathy of Broilers to Metabolic Syndrome in Humans.” Genes 10 (10): 746. https://doi.org/10.3390/genes10100746.
- Lee, D., E. S. Oh, A. Woods, J. R. Couchman, and W. Lee. 1998. “Solution Structure of a Syndecan-4 Cytoplasmic Domain and Its Interaction with Phosphatidylinositol 4,5-Bisphosphates.” Journal of Biological Chemistry 273 (21): 13022–13029. https://doi.org/10.1074/jbc.273.21.13022.
- Ley, K. 2003. “The Role of Selectins in Inflammation and Disease.” Trends in Molecular Medicine 9 (6): 263–268. https://doi.org/10.1016/S1471-4914(03)00071-6.
- Li, Y., Q. G. Ma, L. H. Zhao, H. Wei, G. X. Duan, J. Y. Zhang, and C. Ji. 2014. “Effects of Lipoic Acid on Immune Function, the Antioxidant Defense System, and Inflammation-Related Genes Expression of Broiler Chickens Fed Aflatoxin Contaminated Diets.” International Journal of Molecular Sciences 15 (4): 5649–5662. https://doi.org/10.3390/ijms15045649.
- Lim, S. T., R. L. Longley, J. R. Couchman, and A. Woods. 2003. “Direct Binding of Syndecan-4 Cytoplasmic Domain to the Catalytic Domain of Protein Kinase Cα (PKCα) Increases Focal Adhesion Localization of PKCα.” Journal of Biological Chemistry 278 (16): 13795–13802. https://doi.org/10.1074/jbc.M208300200.
- Liu, L., H. Cui, R. Fu, M. Zheng, R. Liu, G. Zhao, and J. Wen. 2017. “The Regulation of IMF Deposition in Pectoralis Major of Fast- and Slow- Growing Chickens at Hatching.” Journal of Animal Science and Biotechnology 8 (1): 77. https://doi.org/10.1186/s40104-017-0207-z.
- Longley, R. L., A. Woods, A. Fleetwood, G. J. Cowling, J. T. Gallagher, and J. R. Couchman. 1999. “Control of Morphology, Cytoskeleton and Migration by Syndecan-4.” Journal of Cell Science 112 (20): 3421–3431. https://doi.org/10.1242/jcs.112.20.3421.
- Lundberg, I. E. 2000. “The Role of Cytokines, Chemokines, and Adhesion Molecules in the Pathogenesis of Idiopathic Inflammatory Myopathies.” Current Rheumatology Reports 2 (3): 216–224. https://doi.org/10.1007/s11926-000-0082-y.
- Ma, Q., Y. Li, Y. Fan, L. Zhao, H. Wei, C. Ji, and J. Zhang. 2015. “Molecular Mechanisms of Lipoic Acid Protection against Aflatoxin B1-Induced Liver Oxidative Damage and Inflammatory Responses in Broilers.” Toxins 7 (12): 5435–5447. https://doi.org/10.3390/toxins7124879.
- McCormick, R. J. 1999. “Extracellular Modifications to Muscle Collagen: Implications for Meat Quality.” Poultry Science 78 (5): 785–791. https://doi.org/10.1093/ps/78.5.785.
- Moss, F. P., and C. P. Leblond. 1971. “Satellite Cells as the Source of Nuclei in Muscles of Growing Rats.” Anatomical Record 170 (4): 421–435. https://doi.org/10.1002/ar.1091700405.
- Mozdziak, P. E., T. J. Walsh, and D. W. McCoy. 2002. “The Effect of Early Posthatch Nutrition on Satellite Cell Mitotic Activity.” Poultry Science 81 (11): 1703–1708. https://doi.org/10.1093/ps/81.11.1703.
- Mudalal, S., M. Lorenzi, F. Soglia, C. Cavani, and M. Petracci. 2015. “Implications of White Striping and Wooden Breast Abnormalities on Quality Traits of Raw and Marinated Chicken Meat.” Animal 9 (4): 728–734. https://doi.org/10.1017/s175173111400295x.
- Mutryn, M. F., E. M. Brannick, W. Fu, W. R. Lee, and B. Abasht. 2015. “Characterization of a Novel Chicken Muscle Disorder through Differential Gene Expression and Pathway Analysis Using RNA-Sequencing.” BMC Genomics 16 (1): 399. https://doi.org/10.1186/s12864-015-1623-0.
- Niki, E. 2016. “Oxidative Stress and Antioxidants: Distress or Eustress?.” Archives of Biochemistry and Biophysics 595: 19–24. https://doi.org/10.1016/j.abb.2015.11.017.
- Niki, E., N. Noguchi, and N. Gotoh. 1993. “Mechanisms of Free Radical Damage in the Vascular and Central Nervous Systems and Control by Antioxydant Intervention.” Biochemical Society Transactions 21: 313–317. https://doi.org/10.1042/bst0210313.
- NRC (National Research Council). 1994. Nutrient Requirements of Poultry. 9th ed ed. Washington, DC: National Academy Press.
- Palokangas, H., V. Kovanen, R. Duncan, and S. P. Robins. 1992. “Age-related Changes in the Concentration of Hydroxypyridinium Crosslinks in Functionally Different Skeletal Muscles.” Matrix 12 (4): 291–296. https://doi.org/10.1016/S0934-8832(11)80081-8.
- Panda, A. K., and G. Cherian. 2014. “Role of Vitamin E in Counteracting Oxidative Stress in Poultry.” Journal of Poultry Science 51: 109–117. https://doi.org/10.2141/jpsa.0130134.
- Papah, M. B., E. M. Brannick, C. J. Schmidt, and B. Abasht. 2017. “Evidence and Role of Phlebitis and Lipid Infiltration in the Onset and Pathogenesis of Wooden Breast Disease in Modern Broiler Chickens.” Avian Pathology 46 (6): 623–643. https://doi.org/10.1080/03079457.2017.1339346.
- Papah, M. B., E. M. Brannick, C. J. Schmidt, and B. Abasht. 2018. “Gene Expression Profiling of the Early Pathogenesis of Wooden Breast Disease in Commercial Broiler Chickens Using RNA-Sequencing.” PLoS ONE 13 (12): e0207346. https://doi.org/10.1371/journal.pone.0207346.
- Parveen, R., A. Asghar, F. M. Anjum, M. I. Khan, M. S. Arshad, and A. Yasmeen. 2013. “Selective Deposition of Dietary α-Lipoic Acid in Mitochondrial Fraction and Its Synergistic Effect with α-Tocoperhol Acetate on Broiler Meat Oxidative Stability.” Lipids in Health and Disease 12 (1): 52. https://doi.org/10.1186/1476-511X-12-52.
- Powell, D. J., D. C. McFarland, A. J. Cowieson, W. I. Muir, and S. G. Velleman. 2014. “The Effect of Nutritional Status and Muscle Fiber Type on Myogenic Satellite Cell Fate and Apoptosis.” Poultry Science 93 (1): 163–173. https://doi.org/10.3382/ps.2013-03450.
- Purslow, P. 2018. “Contribution of Collagen and Connective Tissue to Cooked Meat Toughness.” Meat Science 144: 127–134. https://doi.org/10.1016/j.meatsci.2018.03.026.
- Rehman, Z., N. Chand, and R. U. Khan. 2017. “The Effect of Vitamin E, L-carnitinE, and Ginger on Production Traits, Immune ResponsE, and Antioxidant Status in Two Broiler Strains Exposed to Chronic Heat Stress.” Environmental Science and Pollution Research 24 (34): 26851–26857. https://doi.org/10.1007/s11356-017-0304-8.
- Rosen, E. D., P. Sarraf, A. E. Troy, G. Bradwin, K. Moore, D. S. Milstone, B. M. Spiegelman, and R. M. Mortensen. 1999. “PPARγ Is Required for the Differentiation of Adipose Tissue in Vivo and in Vitro.” Molecular Cell 4 (4): 611–617. https://doi.org/10.1016/S1097-2765(00)80211-7.
- Rudnicki, M. A., P. N. J. Schnegelsberg, R. H. Stead, T. Braun, H. H. Arnold, and R. Jaenisch. 1993. “Myod or Myf-5 Is Required for the Formation of Skeletal Muscle.” Cell 75 (7): 1351–1359. https://doi.org/10.1016/0092-8674(93)90621-V.
- Rymer, C., and D. I. Givins. 2010. “Effects of Vitamin E and Fish Oil Inclusion in Broiler Diets on Meat Fatty Acid Composition and on the Flavour of a Composite Sample of Breast Meat.” Journal of the Science of Food and Agriculture 90 (10): 1628–1633. https://doi.org/10.1002/jsfa.3991.
- Schultz, E. 1989. “Satellite Cell Behavior during Skeletal Muscle Growth and Regeneration.” Medicine and Science in Sports and Exercise 21 (5 Suppl): S181–S186. https://doi.org/10.1249/00005768-198910001-00010.
- Shin, J., D. C. McFarland, and S. G. Velleman. 2013. “Migration of Turkey Muscle Satellite Cells Is Enhanced by the Syndecan-4 Cytoplasmic Domain through the Activation of RhoA.” Molecular and Cellular Biochemistry 375 (1–2): 115–130. https://doi.org/10.1007/s11010-012-1534-1.
- Sihvo, H. K., K. Immonen, and E. Puolanne. 2014. “Myodegeneration with Fibrosis and Regeneration in the Pectoralis Major Muscle of Broilers.” Veterinary Pathology 51 (3): 619–623. https://doi.org/10.1177/0300985813497488.
- Sihvo, H. K., J. Linden, N. Airas, K. Immonen, J. Valaja, and E. Puolanne. 2017. “Wooden Breast Myodegeneration of Pectoralis Major Muscle over the Growth Period in Broilers.” Veterinary Pathology 54 (1): 119–128. https://doi.org/10.1177/0300985816658099.
- Sohaib, M., F. M. Anjum, M. I. Khan, M. S. Arshad, and M. Shahid. 2012. “Enhancement of Lipid Stability of Broiler Breast Meat and Meat Products Fed on Alpha Lipoic Acid and Alpha Tocopherol Acetate Supplemented Feed.” Lipids in Health and Disease 11: 57. https://doi.org/10.1186/1476-511X-11-57.
- Sohaib, M., F. M. Anjum, M. Nasir, F. Saeed, M. S. Arshad, and S. Hussain. 2018. “Alpha-lipoic Acid: An Inimitable Feed Supplement for Poultry Nutrition.” Journal of Animal Physiology and Animal Nutrition 102 (1): 33–40. https://doi.org/10.1111/jpn.12693.
- Song, Y., D. McFarland, and S. Velleman. 2012. “Syndecan-4 Cytoplasmic Domain Regulation of Turkey Satellite Cell Focal Adhesions and Apoptosis.” Molecular Biology Reports 39 (8): 8251–8264. https://doi.org/10.1007/s11033-012-1673-1.
- Srilatha, T., V. R. Redely, S. Qudratullah, and M. V. L. N. Raju. 2010. “Effect of Alpha-Lipoic Acid and Vitamin E in Diet on the Performance, Antioxidation and Immune Response in Broiler Chicken.” International Journal of Poultry Science 9 (7): 678–683. https://doi.org/10.3923/ijps.2010.678.683.
- Stockdale, F. E., and H. Holtzer. 1961. “DNA Synthesis and Myogenesis.” Experimental Cell Research 24 (3): 508–520. https://doi.org/10.1016/0014-4827(61)90450-5.
- Tijare, V. V., F. L. Yang, V. A. Kuttappan, C. Z. Alvarado, C. N. Coon, and C. M. Owens. 2016. “Meat Quality of Broiler Breast Fillets with White Striping and Woody Breast Muscle Myopathies.” Poultry Science 95 (9): 2167–2173. https://doi.org/10.3382/ps/pew129.
- Tonniges, J. R., D. L. Clark, and S. G. Velleman. 2019. “The Effect of the Wooden Breast Fibrotic Myopathy in Broilers on Fibrillar Collagen Organization and Decorin-collagen Binding.” Avian Diseases 63 (1): 48–60. https://doi.org/10.1637/11985-102218-Reg.1.
- Tontonoz, P., E. Hu, R. A. Graves, A. I. Budavari, and B. M. Spiegelman. 1994. “MPPAR/2: Tissue-Specific Regulator of an Adipocyte Enhancer.” Genes and Development 8 (10): 1224–1234. https://doi.org/10.1101/gad.8.10.1224.
- Velleman, S. G., J. Anderson, and K. E. Nestor. 2003. “Effect of Selection for Growth Rate on Muscle Damage during Turkey Breast Muscle Development.” Poultry Science 82 (7): 1069–1074. https://doi.org/10.1093/ps/82.7.1069.
- Velleman, S. G., and D. L. Clark. 2015. “Histopathologic and Myogenic Gene Expression Changes Associated with Wooden Breast in Broiler Breast Muscles.” Avian Diseases 59 (3): 410–418. https://doi.org/10.1637/11097-042015-reg.1.
- Velleman, S. G., D. L. Clark, and J. R. Tonniges. 2017. “Fibrillar Collagen Organization Associated with Broiler Wooden Breast Fibrotic Myopathy.” Avian Diseases 61 (4): 481–490. https://doi.org/10.1637/11738-080217-reg.1.
- Velleman, S. G., C. S. Coy, and D. A. Emmerson. 2014. “Effect of the Timing of Posthatch Feed Restrictions on Deposition of Fat during Broiler Breast Muscle Development.” Poultry Science 93: 2622–2627. https://doi.org/10.3382/ps.2013-03813.
- Velleman, S. G., C. S. Coy, and D. C. McFarland. 2007. “Effect of Syndecan-1, Syndecan-4, and Glypican-1 on Turkey Muscle Satellite Cell Proliferation, Differentiation, and Responsiveness to Fibroblast Growth Factor 2.” Poultry Science 86 (7): 1406–1413. https://doi.org/10.1093/ps/86.7.1406.
- Velleman, S. G., K. E. Nestor, C. S. Coy, I. Harford, and N. B. Anthony. 2010. “Effect of Posthatch Feed Restriction on Broiler Breast Muscle Development and Muscle Transcriptional Regulatory Factor Gene and Heparan Sulfate Proteoglycan Expression.” International Journal of Poultry Science 9 (5): 417–425. https://doi.org/10.3923/ijps.2010.417.425.
- Wang, J., D. L. Clark, S. K. Jacobi, and S. G. Velleman. 2020a. “Effect of Early Posthatch Supplementation of Vitamin E and Omega-3 Fatty Acids on the Severity of Wooden Breast, Breast Muscle Morphological Structure, and Gene Expression in the Broiler Breast Muscle.” Poultry Science 99 (11): 5925–5935. https://doi.org/10.1016/j.psj.2020.08.043.
- Wang, J., D. L. Clark, S. K. Jacobi, and S. G. Velleman. 2020b. “Effect of Vitamin E and Omega-3 Fatty Acids Early Posthatch Supplementation on Reducing the Severity of Wooden Breast Myopathy in Broilers.” Poultry Science 99 (4): 2108–2119. https://doi.org/10.1016/j.psj.2019.12.033.
- Wang, J., D. L. Clark, S. K. Jacobi, and S. G. Velleman. 2021. “Effect of Vitamin E and Alpha Lipoic Acid on Intestinal Development Associated with Wooden Breast Myopathy in Broilers.” Poultry Science 100: 100952. https://doi.org/10.1016/j.psj.2020.12049.
- Woods, A., and J. R. Couchman. 1992. “Protein Kinase C Involvement in Focal Adhesion Formation.” Journal of Cell Science 101 (2): 277–290. https://doi.org/10.1242/jcs.101.2.277.
- Woods, A., R. L. Longley, S. Tumova, and J. R. Couchman. 2000. “Syndecan-4 Binding to the High Affinity Heparin-Binding Domain of Fibronectin Drives Focal Adhesion Formation in Fibroblasts.” Archives of Biochemistry and Biophysics 374 (1): 66–72. https://doi.org/10.1006/abbi.1999.1607.
- Xing, T., X. Zhao, L. Zhang, J. L. Li, G. H. Zhou, X. L. Xu, and F. Gao. 2019. “Characteristics and Incidence of Broiler Chicken Wooden Breast Meat under Commercial Conditions in China.” Poultry Science 0 (1): 1–9. https://doi.org/10.3382/ps/pez560.
- Yablonka-Reuveni, Z., M. A. Rudnicki, A. J. Rivera, M. Primig, J. E. Anderson, and P. Natanson. 1999. “The Transition from Proliferation to Differentiation Is Delayed in Satellite Cells from Mice Lacking MyoD.” Developmental Biology 210 (2): 440–455. https://doi.org/10.1006/dbio.1999.9284.
- Yeh, W. C., Z. Cao, M. Classon, and S. L. McKnight. 1995. “Cascade Regulation of Terminal Adipocyte Differentiation by Three Members of the C/EBP Family of Leucine Zipper Proteins.” Genes and Development 9 (2): 168–181. https://doi.org/10.1101/gad.9.2.168.
- Zambonelli, P., M. Zappaterra, F. Soglia, M. Petracci, F. Sirri, C. Cavani, and R. Davoli. 2017. “Detection of Differentially Expressed Genes in Broiler Pectoralis Major Muscle Affected by White Striping – Wooden Breast Myopathies.” Poultry Science 95 (12): 2771–2785. https://doi.org/10.3382/ps/pew268.
- Zhang, Y., R. Jia, C. Ji, Q. Ma, J. Huang, H. Yin, and L. Liu. 2014. “Effects of Dietary Alpha-Lipoic Acid and Acetyl-l-Carnitine on Growth Performance and Meat Quality in Arbor Acres Broilers.” Asian-Australasian Journal of Animal Sciences 27 (7): 996–1002. https://doi.org/10.5713/ajas.2013.13436.