Abstract
The changes in cattle acetylcholinesterase (AChE) and butyrylcholinesterase (BChE) activities after exposure to tebuconazole-based fungicides (Orius and Prosaro®) in vitro were determined spectrophotometrically according to the Ellman method. The fungicides induced the decrease in AChE and BChE levels in a dose-dependent manner. Statistically significant inhibition of erythrocyte AChE levels was observed in the concentration range from 150 to 300 μg ml−1 (p < 0.001) for Orius and from 100 to 300 μg ml−1 for Prosaro® (p < 0.01 and p < 0.001, respectively). Levels of BChE activity were more reduced for the fungicide Orius (40–50%) than for Prosaro®. Statistical significance in the inhibition of BChE activity was found after exposure of cattle plasma to both fungicides at concentrations starting from 150 μg ml−1 (p < 0.001). Our results indicate that tebuconazole-based fungicides are able to reduce cattle AChE and BChE activities.
Introduction
Triazoles represent a class of fungicides used in protection of fruit, vegetables, cereals and seeds against fungal diseases (Goetz et al. Citation2006). The mechanism of their antifungal action is based on inhibition of CYP51 (lanosterol 14α-demethylase), a key enzyme for ergosterol biosynthesis in fungi, resulting in alterations of fungal wall and fungal cell growth suppression (Berg et al. Citation1984, Van den Bossche Citation1985).
Extensive use of pesticides in agriculture is responsible for their presence and persistence in the environment and a number of adverse effects on non-target organisms as well. For example, application of pesticides is associated with a wide spectrum of adverse events affecting human health: short-term effects such as headaches and nausea, or chronic effects such as cancer or reproductive and/or endocrine disruptions (Berrada et al. Citation2010). Several studies have shown that the triazole fungicide tebuconazole may cause liver tumours in mice, ocular lesions in dogs and developmental anomalies in mice, rabbits and rats (US Environmental Protection Agency Citation1999, 2010).
Animal cholinesterases are divided into two classes according to their substrate specificity and susceptibility to inhibitors: acetylcholinesterase or true cholinesterase (AChE) and butyrylcholinesterase (BChE) (Dorko et al. Citation2011). Acetylcholine (ACh) is a neurotransmitter that plays a major role in the creation and transmission of neural impulses in the central and peripheral nervous systems (Lotti Citation2001). When inhibition of AChE activity occurs, it can cause overactivation of brain and muscular tissue. This state is caused by insufficient ACh hydrolysis in the nerve synapses and neuromuscular junctions (Roex et al. Citation2003). BChE is also able to decompose acetylcholine, but less efficiently than AChE (Darvesh et al. Citation2003). It is considered to be an endogenous bioscavenger of organophosphorus compounds (Kovarik et al. Citation2010).
Inhibiting effects on cholinesterase activity have been widely studied in fish (Gruber and Munn Citation1998; Dembélé et al. Citation2000; Rodrigues et al. Citation2011), rats (Kim et al. Citation2005; Acker et al. Citation2011; Roszczenko et al. Citation2012), and in farm animals (Karanth and Pope Citation2003; Mohammad et al. Citation2007; Berger et al. Citation2008) after exposure to organophosphate and carbamate pesticides. In contrast to these studies, reports dealing with AChE activity after exposure to triazole fungicides are rare. For example, Toni et al. (Citation2011) described an increase in AChE activity in the brain when Cyprinus carpio was exposed to tebuconazole.
The aim of our study was to spectrophotometrically assess changes in AChE and BChE activity in vitro in plasma and erythrocytes of cattle after exposure to different concentrations of technical triazole fungicides (Orius 25EW and Prosaro 250EC®). We used cattle as the model because grazing animals may be most affected by the harmful influence of pesticides via their feed, and thus serve as indicators of environmental exposure (Pistl et al. Citation2003). One of the precise methods used for monitoring exposure of animals to pesticides is determination of blood cholinesterase (ChE) activity. It has been reported that ChE activity in ruminants is inherently low in the plasma, and 90% of the total level is present in red blood cells (Pardio et al. Citation2007; Miao et al. Citation2010).
Materials and methods
Chemicals
The tebuconazole-based fungicides, trade name Orius 25EW (α-terc.butyl–α-4-chlorophenylethyl}-1H-1,2,4-triazolyl-ethanol, 25% of active agent) and Prosaro 250EC® (12.5% tebuconazole plus 12.5% prothioconazole) were obtained from the Research & Plant Station, Slovak Republic and used in the experiments at concentrations of 50, 100, 150, 200, 250 and 300 μg ml−1 for AChE and BChE measurements. Enzyme substrate, acetylthiocholine iodide (ATCh), thiol reagent DTNB {5,5′-dithiobis (2-nitrobenzoic acid)}, and ethanol (96%; all purchased from Sigma Chemical, St. Louis, MO, USA) were used in cholinesterase activity measurements. Both the cattle erythrocytes (source of AChE) and plasma (source of BChE) were used for experiments. All experiments were done in 0.1 M sodium phosphate buffer, pH 7.4 at 25°C.
Sample preparation
Blood samples were collected by jugular venipuncture from six healthy bulls (6 months old, 150–200 kg, Slovakian spotted cattle (Bos taurus L.)). Plasma was obtained after whole blood centrifugation at 1000 rpm (120 g) for 10 min. Subsequently erythrocytes were washed with phosphate buffer (0.1 M, pH 7.4) and twice centrifuged. Dilutions (25 to 100-fold) were made in the plasma, while the final dilution of red blood cells was 800-fold. The samples were then frozen (–20°C) and used in in vitro experiments.
Cholinesterase activity measurements
The native enzymes activities were measured spectrophotometrically according to Ellman et al. (Citation1961) using thiol reagent DTNB at a concentration of 0.3 mM, and substrate ATCh at a concentration of 1 mM. The values of absorbance (ΔA min−1) were read at 436 nm and 412 nm up to 5 min for AChE and BChE respectively (Reiner et al. Citation2000). No additional inhibitor was used in the native enzymes activity measurements. The effects of Orius and Prosaro® were assessed after 5 min incubation with different concentrations of the fungicides. Ethanol (96%) at the concentration of 4% (v/v) was used as a fungicide solvent and was also added to the control sample. The final reaction volume for both enzymes was 1 ml. Spectrophotometric measurements were performed on a CARY 300 spectrophotometer (Varian, Mulgrave, Australia).
ChE activities were calculated using a DTNB extinction coefficient of 11.0 × 103 M−1 cm−1 for AChE and 14.22 × 103 M−1 cm−1 for BChE (Eyer et al. Citation2003), and are expressed as U/L (1 U = 1 μmol min−1).
Statistical analysis
Data were evaluated using ANOVA; and then Tukey’s multiple comparison test was used to estimate the inhibition of AChE and BChE.
Results
The baseline levels of cattle AChE and BChE activities are presented in Table ; the mean values ± SD from six animals are included. ChE activity was higher in the cattle erythrocytes than in the plasma.
Table 1. Native cattle ChE activities in erythrocytes and plasma samples. Peripheral blood of six animals was used in the experiments.
In vitro inhibition of AChE after treatment of cattle erythrocytes with the fungicides Orius and Prosaro® is presented in Figure . Dose dependence of the decrease in AChE levels was found after exposure to both fungicides tested. Statistically significant inhibition (p < 0.001) was found after treatment of cattle erythrocytes with the fungicides; for Orius in the concentration range from 150 to 300 μg ml−1, and for Prosaro® from 100 to 300 μg ml−1 (p < 0.01, and p < 0.001 respectively; Figure ).
Figure 1. In vitro inhibition of AChE activity in cattle erythrocytes after exposure to tebuconazole-based fungicides (Orius and Prosaro®) from six animals.
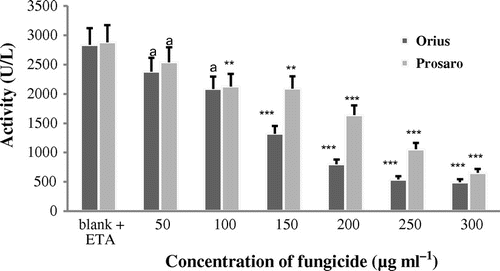
The BChE activity responses after exposure to Orius and Prosaro® fungicides are shown in Figure . No significant inhibition was recorded at the lower fungicide concentration (50 and 100 μg ml−1). High, statistically significant inhibition was detected after exposure of cattle plasma to both fungicides, starting from the concentration 150 μg ml−1 (p < 0.001).
Discussion
Our results show that the tebuconazole-based fungicides Orius and Prosaro® have the potential to inhibit ChE activities in vitro. Significant AChE inhibition was observed after exposure of cattle erythrocytes to Orius at concentrations ranging from 150 to 300 μg ml−1 (p < 0.001), and to Prosaro® at concentrations from 100 to 300 μg ml−1 (p < 0.01 and p < 0.001 respectively, Figure ). We assume that differences in the initial levels of inhibition could be caused by the different concentrations of the active agent in the fungicides tested (25% tebuconazole in Orius), in comparison with Prosaro® (12.5% tebuconazole plus 12.5% prothioconazole). Similar results were seen in BChE inhibition (p < 0.001) after treatment of the cattle plasma with both fungicides. Levels of BChE activity were more reduced for the fungicide Orius than for Prosaro®.
The distribution of cattle ChE activities between plasma and erythrocytes is presented in Table . Our results correspond with the data reported by other researchers (Tecles and Ceron Citation2001; Karanth and Pope Citation2003) in cows, but the values of BChE activities in cattle plasma were somewhat lower than those described by these authors.
There is a lack of reports dealing with ChE activity in animals after exposure to triazole fungicides. It is known that the mode of triazole action is different from typical anticholinesterase compounds, such as OPs. Ergosterol-biosynthesis-inhibiting (EBI) fungicides are known to amplify toxicity of OPs. They induce cytochrome P450 forms responsible for activation of the OP. This activation can lead to an increase in cholinesterase inhibition. Johnston et al. (Citation1994) assessed the interaction between EBI and OP in the red-legged partridge. They found that pre-treatment of the red-legged partridge with EBI fungicides (prochloraz, propiconazole and penconazole) and subsequent exposure to OP insecticides resulted in enhanced toxicity. Similar results were described after exposure of California quail to myclobutanil and OP insecticides (Wilson et al. Citation2001); and in male rats after exposure to clotrimazole and parathion or malathion insecticides (Ronis et Badger 1995). Barsh et al. (Citation2008) reported that OPs and carbamates inhibit AChE, the enzyme that re-sets nerve synapses at the end of transmission, while triazoles interfere with γ amino butyric acid receptors on the animal neurons and cause stuttering.
ChE activity after exposure of Cyprinus carpio to tebuconazole-based fungicide was investigated by Toni et al. (Citation2011). Carp fish were exposed to different concentrations of the fungicide in a rice field (31.95 μg l−1) and in laboratory conditions (33.47 μg l−1 and 36.23 μg l−1) for 7 days. A significant increase in brain AChE activity was found only in the field conditions, whereas no differences in muscle AChE activity were observed. The authors assumed that the different responses in enzyme activity (in brain and muscle) could be caused by reaction in the fish to possible stress induced by tebuconazole. Similarly, the increase in AChE activity was described after exposure of unfertilized eggs, embryos, tissues and organs of sea squirt Ciona intestinalis by tributyltin (Cangialosi et al. Citation2009; Manuseto et al. Citation2012). The authors assumed that the increase in AChE activity could be caused by increased gene expression for enzyme synthesis.
In contrast to these studies, depression of ChE activity was seen in early stage embryos of zebrafish (Danio renio) after exposure to prochloraz (Domingues et al. Citation2013). Both adults and embryos were exposed to the fungicide at concentrations of 0.3, 0.6, 1.2, 2.4, 4.8 and 9.6 mg l−1 for 96 h, and also for 6 days. Inhibition of ChE activity was detected in larvae after 96 h exposure to the fungicide at the highest concentrations (4.8 mg l−1). It was supposed that besides the role in neurotransmission, ChE is also important for neuronal and muscular development in zebrafish (Behra et al. Citation2002). Domingues et al. (Citation2011) presumed that there was possible interference as a result of the embryos’ mechanical movements necessary for hatching. In addition to this, none of the embryos was observed to hatch after prochloraz treatment at doses of 4.8 and 9.6 mg l−1. Prochloraz can also interact with the iron atom of cytochrome P450 and catalase and even induce an increase in antioxidant enzymes and decrease in glutathione (GSH) levels (Sanchez et al. Citation2008). A connection between the decrease in ChE activity and strong inhibition of glutathione-S-transferase (GST) and catalase was assumed by Schreck et al. (Citation2008) in the earthworm Aporrectodea caliginosa nocturna after exposure to a mixture of pesticides (myclobutanil, matelaxyl-M, fosatyl-Al, folpet). The highest ChE inhibition (p < 0.05) was detected after 3 days of exposure to the fungicide at the maximum dose. It is likely that inhibition of GST and catalase (p < 0.1) indicates serious toxic stress. The enzymes probably cannot cope with this stress because of cellular dysfunction or apoptosis (Sweet et al. Citation1999; Brown et al. Citation2004).
Changes in ChE activity were very often recorded in association with oxidative stress, e.g. after in vivo exposure of Cyprinus carpio to imazethapyr and imazapic (Moraes et al. Citation2011), lindane and DDT in rats (Koner et al. Citation1997) and malathion in humans (Seth et al. Citation2001).
Conclusion
In conclusion, our results indicate the sensitivity of cattle AChE and BChE activities to exposure to triazole fungicides. Data on the inhibitory effect of triazole fungicides on ChE activity would appear to be a suitable biomarker for evidence of exposure in environmental studies (Pardio et al. Citation2001).
Acknowledgements
This work was supported by the Ministry of Education and Science of the Slovak Republic under contract No. VEGA 1/0125/11, No. VEGA 1/0117/13, and part of the study was supported by the Ministry of Science, Education and Sport, Republic of Croatia, Grant No. 022-0222148-2889, awarded to Zrinka Kovarik, Croatia.
References
- Acker CI, Souza ACG, Pinton S, Trevisan da Rocha J, Friggi CA, Zanella R, Nogueira CW. 2011. Repeated malathion exposure induces behavioural impairment and AChE activity inhibition in brains of rat pups. Ecotoxicol Environ Safe. 74(8):2310–2315.
- Barsh R, Bell J, Halliday H, Clifford M, Mottet G. 2008. Preliminary survey of pyrethroid pesticides and surfactants in San Juan County surface waters. Lopez, WA: KWIAHT, Centre for the Historical Ecology of the Salish Sea.
- Behra M, Cousin X, Bertrand C, Vonesch J, Biellmann D, Chatonet A, Strähle U. 2002. Acetlycholinesterase is required for neuronal and muscular development in the zebrafish embryo. Nat Neurosci. 5(2):111–118.
- Berg D, Regel E, Harenberg HE, Plempel L. 1984. Bifonazole and clotrimazole. Their mode of action and the possible reason for the fungicidal behaviour of bifonazole. Arzneimittelforsch. 34(2):139–146.
- Berger J, Valdez S, Puschner B, Leutenegger CM, Gardner IA, Madigan JE. 2008. Effects of oral tetrachlorvinphos fly control (Equitrol®) administration in horses: physiological and behavioural findings. Vet Res Commun. 32(1):75–92.
- Berrada H, Fernández M, Ruiz MJ, Moltó JC, Mañes J, Font G. 2010. Surveillance of pesticide residues in fruits from Valencia during twenty months (2004/05). Food Contr. 21(1):36–44.
- Brown PJ, Long SM, Spurgeon DJ, Svendsen C, Hankard PK. 2004. Toxicological and biochemical responses of the earthworm Lumbricus rubellus to pyrene, a non-carcinogenic polycyclic aromatic hydrocarbon. Chemosphere. 57(11):1675–1681.
- Cangialosi MV, Mansueto V, Puciia E, Corsi I, Bonacci S, Focardi S, Mazzola A. 2009. A biochemical study of the effects of tributyltin on unfertilized eggs, embryos and larvae of the sea squirt Ciona intestinalis. Caryologia. 62(4):309–315.
- Darvesh S, Hopkins DA, Geula C. 2003. Neurobiology of butyrylcholinesterase. Nat Rev Neurosci. 4(2):131–138.
- Dembélé K, Haubruge E, Gaspar Ch. 2000. Concentration effects of selected insecticides on brain acetylcholinesterase in the common carp (Cyprinus carpio L.). Ecotoxicol Environ Safe. 45(1):49–54.
- Domingues I, Oliveira R, Musso CM, Cardoso M, Soares AMVM, Loureiro S. 2013. Prochloraz effect on biomarkers activity in Zebrafish early life stages and adults. Environ Toxicol.28(3):155–163.
- Dorko F, Danko J, Flešárová S, Boroš E, Sobeková A. 2011. Effect of pesticide bendiocarbamate on distribution of acetylcholine- and butyrylcholine-positive nerves in rabbit’s thymus. Eur J Histochem. 55(4):206–209.
- Ellman GL, Courtney KD, Andres V Jr, Featherstone RM. 1961. New and rapid colorimetric determination of acetylcholinesterase activity. Biochem Pharmacol. 7(2):88–95.
- Eyer P, Worek F, Kiderlen D, Sinko G, Stuglin A, Simeon-Rudolf V, Reiner E. 2003. Molar absorption coefficients for the reduced Ellman reagent: reassessment. Analyt Biochem. 312(2):224–227.
- Goetz AK, Bao W, Ren H, Schmid JE, Tully DB, Wood C, Rockett JC, Narotsky MG, Sun G, Lambert GR, Thai S-F, Wolf DC, Nesnow S, Dix DJ. 2006. Gene expression profiling in the liver of CD-1 mice to characterize the hepatotoxicity of triazole fungicides. Toxicol Appl Pharmacol. 215 (2):274–284.
- Gruber SJ, Munn MD. 1998. Organophosphate and carbamate insecticides in agricultural waters and cholinesterase (ChE) inhibition in common carp (Cyprinus carpio). Arch Environ Contam Toxicol. 35(3):391–396.
- Johnston G, Walker CH, Dawson A. 1994. Interactive effects between EBI fungicides (prochloraz, propiconazole and penconazole) and OP insecticides (dimethoate, chlorpyrifos, diazinon and malathion) in the hybrid red-legged partridge. Environ Toxicol Chem. 13(4):615–620.
- Karanth S, Pope C. 2003. In vitro inhibition of blood cholinesterase activities from horse, cow and rat by tetrachlorvinphos. Int J Toxicol. 22(6):429–433.
- Kim J-R, Kim H-J, Kwon OS. 2005. Acetylcholinesterase and neuropathy target esterase activity in female and male rats exposed to pesticide terbufos. Environ Toxicol Pharmacol. 20(1):149–156.
- Koner BC, Banarjee BD, Ray A. 1997. Modulation of gamma glutamyl transpeptidase activity in lymphoid system by organochlorine pesticides in rats. Indian J Exp Biol. 35(10):1132–1134.
- Kovarik Z, Katalinić M, Šinko G, Binder J, Holas O, Jung Y-S, Musilova L, Jun D, Kuča K. 2010. Pseudo-catalytic scavenging: Searching for suitable reactivator of phosphorylated butyrylcholinesterase. Chem-Biol Interact. 187(1-3):167–171.
- Lotti M. 2001. Clinical toxicology of anticholinesterase agents in humans. In: Krieger R, editor. Handbook of pesticide toxicology. Vol. 2. USA Academic Press. p. 1043–1086.
- Mansueto V, Cangialosi MV, Arukwe A. 2012. Acetylcholinesterase activity in juvenile Ciona intestinalis (Ascidiacea, Urochordata) after exposure to tributyltin. Caryologia. 65 (1):18–26.
- Miao Y, He N, Zhue J-J. 2010. History and new developments of assay for cholinesterase activity and inhibition. Chem Rev. 110(9):5216–5234.
- Mohammad FK, Al-Zubaidy MHI, Alias AS. 2007. Electrometric determination of erythrocyte, plasma and whole blood cholinesterase activities in sheep, goats and cattle and their in vitro inhibition by anticholinesterase insecticides. J Pharmacol Toxicol. 2(2):131–141.
- Moraes BS, Clasen B, Loro VL, Pretto A, Toni C, de Avila LA, Marchesan E, de Oliveira Machado SL, Zanella R, et al. 2011. Toxicological responses of Cyprinus carpio after exposure to a commercial herbicide containing imazethapyr and imiazapic. Ecotoxicol Environ Safe. 74 (3):328–335.
- Pardio VT, Ibarra N, Rodriguez MA, Waliszewski KN. 2001. Use of cholinesterase activity in monitoring organophosphate pesticide exposure of cattle produced in tropical areas. J Agric Food Chem. 49(12):6057–6062.
- Pardio VT, Ibarra Nde J, Waliszewski KN, López KM. 2007. Effect of coumaphos on cholinesterase activity, hematology, and biochemical blood parameters of bovines in tropical regions of Mexico. J Environ Sci Health B. 42(4):359–366.
- Pistl J, Kovalkovičová N, Holovská V, Legáth J, Mikula I. 2003. Determination of the immunotoxic potential of pesticides on functional activity of sheep leukocytes in vitro. Toxicology. 188(1):73–81.
- Reiner E, Šinko G, Škrinjarić-Špoljar M, Simeon-Rudolf V. 2000. Comparison of protocols for measuring activities of human blood cholinesterases by the Ellman method. Arhiv za Higijenu Rada i Toksikologiju. 51(1):13–18.
- Rodrigues SR, Caldeira C, Castro BB, Gonçalves F, Nunes B. 2011. Cholinesterase (ChE) inhibition in pumpkinseed (Lepomis gibbosus) as environmental biomarker: ChE characterization and potential neurotoxic effects of xenobiotics. Pestic Biochem Physiol. 99(2):181–188.
- Roex EWM, Keijzers R, Gestel CAM. 2003. Acetylcholinesterase inhibition and increased food consumption rate in the zebra fish, Danio renio, after chronic exposure to parathion. Aquat Toxicol. 64(4):451–460.
- Ronis MJJ, Badger TM. 1995. Toxic interactions between fungicides that inhibit ergosterol biosynthesis and phosphorothioate insecticides in the mail rat and Bobwhite quail (Colinus virginianus). Toxicol Appl Pharmacol. 130(2):221–228.
- Roszczenko A, Rogalska J, Moniuszko-Jakoniuk J, Brzóska MM. 2012. The effect of exposure to chlorfenvinphos on lipid metabolism and apoptotic and necrotic cells death in the brain of rats. Exp Toxicol Pathol. 65(5):531–539.
- Sanchez W, Piccini B, Porcher JM. 2008. Effect of prochloraz fungicide on biotransformation enzymes and oxidative stress parameters in three-spined stickleback (Gasterosteus aculeatus). J Environ Sci Health B. 43(1):65–70.
- Schreck E, Geret F, Gontier L, Treilhou M. 2008. Neurotoxic effect and metabolic responses induced by a mixture of six pesticides on the earthworm Aporrectodea caliginosa nocturna. Chemosphere. 71(10):1832–1839.
- Seth V, Banerjee BD, Bhattacharya A, Pasha ST, Chakravorty AK. 2001. Pesticide induced alterations in acetylcholinesterase and gamma glutamyl transpeptidase activities and glutathione level in lymphocytes of human poisoning cases. Clin Biochem. 34(5):427–429.
- Sweet L, Passino-Reader D, Meier P, Omann G. 1999. Xenobiotic induced apoptosis: significance and potential application as a general biomarker of response. Biomarkers. 4(4):237–253.
- Tecles F, Ceron JJ. 2001. Determination of whole blood cholinesterase in different animal species using specific substrates. Res Vet Sci. 70(3):223–238.
- Toni C, Loro VL, Santi A, de Menezes CC, Cattaneo R, Clasen BE, Zanella R. 2011. Exposure to tebuconazol in rice field and laboratory conditions induces oxidative stress in carp (Cyprinus carpio). Comp Biochem Physiol. 153(1):128–132.
- US Environmental Protection Agency. 1999. Tebuconazole. Pesticide Tolerance. Federal Register. 64(5):1132–1138.
- US Environmental Protection Agency. 2010. Tebuconazole. Pesticide Tolerances. Federal Register. 75(86):24421–24428.
- Van den Bossche H. 1985. Biochemical targets for antifungal azole derivates:hypothesis on the mode of action. Curr Top Med Mycol. 1:313–351.
- Wilson L, Martin PA, Elliott JE, Mineau P, Cheng KM. 2001. Exposure of California quail to organophosphorus insecticides in apple orchards in the Okanagan Valley, British Columbia. Ecotoxicology. 10(2):79–90.