Abstract
Developmental aspects of anther, pollen grains, ovule, embryo and seed has described in Onobrychis persica Sirj. and Rech.f. (Fabaceae) under bright field, polarizing and fluorescence microscopy. Anther development starts when the flowers are very small. The anther is tetrasporangiate, and its wall development follows the dicotyledonous type and consists of four layers: epidermis, endothecium, middle layer and a secretory tapetum. Cytokinesis is simultaneous and arrangement of microspores is tetrahedral and tetragonal. Fibrous thickenings are developed in the endothecium when shed. Ellipsoidal tricolpate pollen grains are two-celled when anthers dehisce. The young hemianatropous ovule changes to a anatropous, crassinucellar and bitegumic mature one with zigzag micropyle. Meiosis of megasporocytes results in a T-shaped tetrad. The chalazal megaspore develops into an eight-nucleate embryo sac with the pattern of Polygonum type. The polar nuclei remain separated before fertilization. After cellularization of endosperm, peripheral cells show dense lipid content. The axial embryo shows fleshy cotyledons, which accumulate lipid and starch. The inner integument differentiates into an endothelium and largely vanishes during development while the outer one produces several layers and establishes the typical seed coat structure: macrosclereid cells, osteosclereids and parenchyma cells. Different compounds, such as starch and lipid content were demonstrated with special staining in the tissues. The systematic significance of the embryological characters is discussed in O. persica.
Introduction
The genus Onobrychis belonging to the tribe Hedysareae, from the Fabaceae family, with nearly 130 perennial and annual species, is mainly distributed in the Anatolia–Iran–Caucasian triangle (Arslan and Ertuğrul Citation2010). Onobrychis is an economically important genus used to improve the quality of the soil. It is also harvested as dried, fresh and purebred fodder (Arslan and Ertuğrul Citation2010).
This genus is subdivided into two subgenera, namely Onobrychis and Sisyrosema Bunge (Schischkin and Bobrov Citation1972; Rechinger Citation1984; Ahangarian et al. Citation2007; Duan et al. Citation2015). Onobrychis and Sisyrosema are represented by different karyotype characters, molecular investigations and geographical origins (Rechinger Citation1984; Hesamzadeh Hejazi and Ziaei Nasab Citation2010; Karamian et al. Citation2012; Ranjbar et al. Citation2010, Citation2012; Lewke Bandara et al. Citation2013).
In Iran, 54 species were treated under eight sections: Dendrobrychis, Lophobrychis, Onobrychis, Laxiflorae, Anthyllium, Afghanicae, Heliobrychis and Hymenobrychis (Rechinger Citation1984). There are many contradictions and uncertainty in the taxonomy of this genus, which is often confused with Hedysarum (Boissier Citation1872; Aktoklu Citation2001). Lewke Bandara et al. (Citation2013) considered that the species delimitation of sect. Onobrychis is difficult.
O. persica, with the meiotic chromosome number of 2n = 2x = 14 (Karamian et al. Citation2012), belongs to the Onobrychis section and is endemic in Iran. Embryological characters of this species are reported here for the first time.
Embryological studies in Papilionoideae (Ashrafunnisa and Pullalah Citation1999; Riahi et al. Citation2003; Faigo´n-Soverna et al. Citation2003; Moço and Mariath Citation2003; Zulkarnain Citation2005; Galati et al. Citation2006; Rezanejad Citation2007; Rodriguez-Pontes Citation2008; Riahi and Zarre Citation2009; Salinas-Gamboa et al. Citation2016) and a few embryological studies in Onobrychis (Chehregani et al. Citation2011; Ghassempour and Majd Citation2012) have provided useful characters in embryogenesis and classification. Therefore, in this study we investigate detailed embryological processes in O. persica using bright field, polarizing and fluorescence microscopy and compare them with some spices in this genus. These valuable characters can be used to evaluate the genera of this tribe and investigate their position.
Materials and methods
The voucher specimen is deposited at the Bu–Ali Sina University Herbarium (BASUH 1098) and labeled as follows: Iran, Hamedan, Kabudar Ahang, Subashy village, alt. 2236 m.
Light microscopy (LM)
Tiny buds, flowers and fruits in different developmental stages, at least 30 samples for each developmental stage from the same population, were fixed in FAA70, stored in 70% ethanol, embedded in paraffin, and sectioned at 4–6 μm with Micro DC 4055 microtome. Staining was carried out with the periodic acid Schiff (PAS) and Meyer’s hematoxylin techniques. Several sections were viewed under a Zeiss Axiostar Plus bright field microscope for each embryological developmental stage.
The sections of seeds were stained with different histochemical techniques: toluidine blue O (TB-O) 0.5% aqueous solution, pH 3.0 (Merck, Darmstadt, Germany) was used in order to identify phenols and acidic polysaccharides (O’Brien et al. Citation1964; Gordon and McCandless Citation1973); periodic acid–Schiff (PAS) to identify neutral polysaccharides (Gahan Citation1984); Lugol to identify starch grains (Johansen Citation1940); and Coomassie Brilliant Blue (CBB) 0.4% in Clarke’s solution (Serva, Heidelberg, Germany) to identify proteins (Fisher Citation1968; Gahan Citation1984). Sudan Black B (SB–B) staining was used to identify lipids (Johansen Citation1940). Sections were analyzed with a Canon G11 digital camera (Tokyo, Japan) attached to a Zeiss Axiostar Plus microscope (Medac, Wedel, Germany). Polarized light was also used for detection of birefringent molecules.
Fluorescence microscopy (FM)
The sliced specimens, without staining or after staining, were analyzed using an ultraviolet (UV) light-emitting diode (wavelength of 405 nm) in the epifluorescent microscope (Bell, Monza, Italy) equipped with the Image Capture Q Capture Pro 5.1 Software (Qimaging Corporation, Austin, TX, USA). Fluorochromes (aniline blue) and autofluorescence investigation were used in an epifluorescence system equipped with a UV filter (WU: 330–385 nm), a dichroic mirror (400 nm) and a barrier filter (420 nm).
Pollen tubes were also examined using fluorescence microscopy. The samples were softened in 1.0 M NaOH for 1 h, rinsed in distilled water for 0.5 h, and stained in 0.1% solution of aniline blue in 0.1 M K2HPO4 for 4 h. Pollen tubes were viewed with UV epifluorescence using a BP 355–425 excitation filter and a LP 460 barrier filter.
Results
Male gametophyte
Anther development starts when the flowers are very small. They are ovoid shaped and tetrasporangiated (Figures ). The anther wall development follows the dicotyledonous type, consists of four layers: epidermis, endothecium, the middle layer and a secretory tapetum (Figure ). Microspore mother cells (MC) are surrounded by a callose coat. They contain a big nucleus at the center (Figures ). The tapetal cells are uni-nucleate (Figure ). Cytokinesis is simultaneous in MC, after meiosis and the arrangement of microspores is tetrahedral and tetragonal. They are surrounded by a callose coat (Figure ).
Microspores undergo unequal mitotic division and produce a small generative and a large vegetative cell (Figure ). Ellipsoidal tricolpate pollen grains are two-celled at the time of shedding (Figure ). In this time tapetum degenerates completely and only endothecium and epidermis are visible. Fibrous thickenings are developed in the endothecium when shed (Figure ). Growing and branching of pollen tube in the pistil and style is visible using fluorescence microscopy. Aniline blue staining showed pollen tube by fluorescence microscopy (Figures ).
Female gametophyte
Megasporocytes undergo successive meiosis to forms unequal dyad cells (Figure ) and then four megaspores in a T-shaped tetrad (Figure ). The functional megaspore, which is located in the chalazal position, undergoes three successive stages of mitotic divisions and develops into an eight-nucleate embryo sac. The pattern of the embryo sac development follows a Polygonum type: the egg cell and two synergids that formed egg apparatus, the central cell that is the largest cell and three antipodal (Figure ).
Figures 1-15. Longitudinal sections showing embryo sac development under bright field and fluorescence microscopes and different magnifications. 1: Young ovary containing a young ovule (right) showing dyad stage (right and left) (black arrowhead). 2: Two different magnifications of a T-shaped tetrad (black arrowheads). The outer integument (oi) grows faster than inner one (ii). 3: Three nuclei of four-nucleated embryo sac (black arrowheads). 4: Anatropous, crassinucellar and bitegmic ovules with nucellus and provascular bundle (PVB). The cone-shaped embryo sac with egg apparatus, two polar nuclei, antipodal cells and zigzag micropyle. 5: One of the synergids (SY) and oospher with its polarity are visible under bright field (black arrowheads) and fluorescence microscopes. 6: Synergids (black arrowheads), egg cell (EC), two polar nuclei before fusion and degeneration of micropylar end is visible. 7: Two polar nuclei and their finger-like tubers. 8: Egg apparatus and secondary nucleus (black arrowhead) formation after fusion of polar nuclei under bright field (left) and fluorescence (right) microscopes. 9: Mature embryo sac with differentiated egg (white arrowhead) and its polarity. 10: Sagittal section through the embryo sac under bright field and fluorescence microscopes with two different magnifications. Nucellar cells are degraded in the middle of embryo sac but persistent adjacent to the chalazal areae. Endothelium layer (En) is distinguishable at the inner integument. 11: Longitudinal section of zygote (Z) and synergids. 12: The primary endosperm nucleus (PEN), apical and basal cells (black arrowheads). 13: Basal cell and 2-celled proembryo (black arrowheads). 14: The U-shaped embryo sac and proembryo (Pe) under bright field (right) and fluorescence microscopes (left). The ovary with trichomes (in different magnifications; black arrowheads), long style (black arrow). 15: Globular embryo (Em) with young suspensor (S) surrounded by coenocytic endosperm. Staining with lugol indicates accumulation of starch grains at the base of embryo (black arrow).
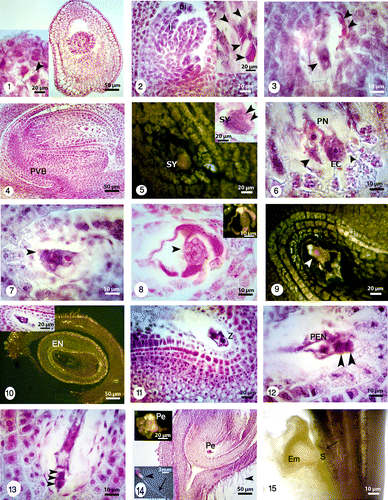
The young ovule is hemianatropous but the mature one is anatropous, crassinucellar (Figure ) and bitegmic; integuments form a zigzag micropyle. A vascular bundle (procambium) begins to differentiate in the funiculus (Figure ).
After cellularization of the embryo sac two polar nuclei are close to the egg apparatus (Figures ). The polar nuclei remain separated from each other until fertilization. The multilayered coenocytic nucellus becomes partly cellular (Figures ). As the embryo sac enlarges, the cone-shaped embryo sac changes to a U-shaped one and the nucellar cells begin to degenerate (Figures ). After fertilization, the inner layer of the inner integument forms endothelium with cube-shaped cells (Figures ).
The inner integument consists of two cell layers, while the outer one is composed of four to five layers at its thinner part (Figures ). In O. persica, the ovary has long peduncle and trichomas (Figure ). The young ovary has one ovule and the mature legume contained only one seed, but rarely a two-seed legume is possible (Figure ). In zygote stage, the nucleus of the zygote is not easy to distinguish due to density of cytoplasmic materials (Figure ).
Figures 16-25. Different developmental stages of seed and embryo in Onobrychis persica under bright field and fluorescence microscopes with different magnifications. 16: Early heart-shaped embryo (EM) with suspensor (S) consisting of one column of cells. 17: The heart-shaped embryo (EM) and suspensor (S). 18: Onobrychis mature legume contained just one seed but the legumes with two seeds were also observed. 19: Longitudinal section of seed stained with methylene blue shows the distinguishable layers in seed. 20: Transverse section of seed stained with Sudan black B and polarizing microscopy shows lipid reserves in cuticle layer (C) and endosperm (EN) specially in aleurone layer (black arrowhead). 21: Staining of seed coat and endosperm with toluidine blue indicates starch grains (black arrowheads); positive reaction for phenolic compounds gives a green color. Starch grains are absent in macrosclereids (MS) but there are high accumulation of starch grains in osteosclereids (OS) and parenchyma cells (P). Cuticle layer (C) is thick. 22: Staining with lugol indicates starch grains (black arrows). Storage of starch grains during embryogenesis is visible in the seeds. The tracheid bar is distinguishable (white arrowhead). 23: At the hilar pole, double palisade layer (white arrowheads), subhilar parenchyma, and tracheid bar is distinguishable. The aleurone layer is also noticeable (black arrowhead) in the larger figure. 24: A part of testa showing macrosclereids (MS), osteosclereids (OS) and parenchyma cells (P), and accumulation of starch grains. 25: Cotyledon (CO) stage which accumulate starch. In this stage suspensor shows signs of degeneration (black arrow). Note: In Figures 19–25 seed sections were stained with different staining techniques and different microscopes were used (bright field, polarizing and fluorescence).
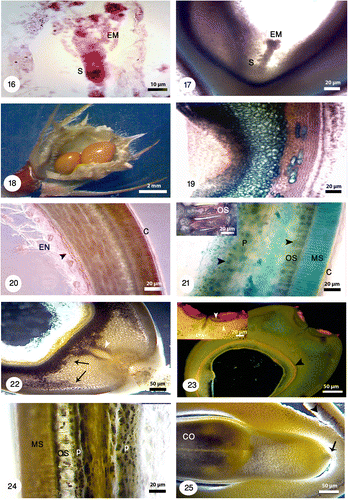
Figures 26-33. Anther structure under bright field and fluorescence microscopes with different magnifications. 26: Microspore mother cells surrounding by a callose coat (white arrow) in a young anther with undifferentiated wall (black arrow). The vascular bundles are distinguishable (white arrowhead). 27: Microspore mother cells are surrounded by a callose coat (black arrow). A big nucleus is visible at the center. 28: The arrangement of tetrads inside the callose walls is tetrahedral (white arrow) and tetragonal (white arrowhead). 29: Released microspores in polar and equatorial view (white arrowheads). The tapetal cells are uni-nucleate (white arrow). 30: Microspores undergoes mitotic division and produces a vegetative cell (black arrowhead) and a generative cell (black arrow). The anther wall consists of four layers: epidermis (E), endothecium (EN), the middle layer (ML) and a secretory tapetum (T). 31: Fibrous thickenings are developed in the endothecium (white arrowhead). 32: The anther is tetrasporangiate with thick exine schahuensis are T-shaped microspores (black arrow). The anther wall layers are distinguishable with fluorescence microscope (white arrow). 33: Two-celled mature pollen with generative and vegetative nuclei (black arrowheads) in a mature anther.
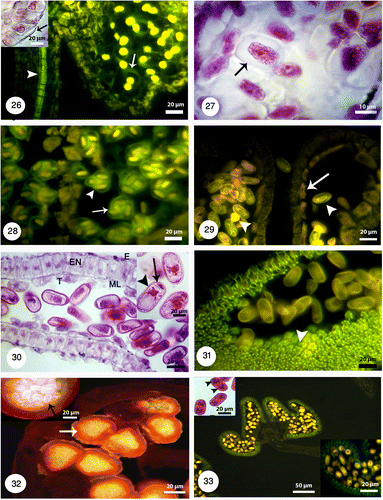
Figures 34-37. 34: Degeneration of micropylar end during fertilization (white arrow). Pollen tube growth with fluorescence microscopy and aniline blue staining: 35: Pollen tubes stained with aniline blue (white arrowheads) shows growing and branching on the tube in the space of between integuments. 36: Longitudinal section of the pistil showing pollen tube growth (white arrowheads) through the short open stylar canal that leads to an ovule. 37: Pollen tube growing through the micropyle (white arrowheads).
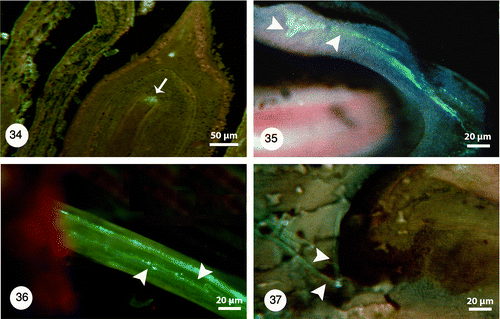
The zygote undergoes a transverse division to form the apical and basal cells (Figure ). A small proembryo forms after several divisions (Figure ). It differentiates into a globular embryo and a short suspensor (Figure ). The coenocytic endosperm in this stage is still around the embryo. Staining with lugol indicates starch grain accumulation at the base of embryo (Figure ).
At the heart stage, the suspensor is composed of one cell column. At cotyledon stage the suspensor shows signs of degeneration (Figure ).
During embryogenesis the inner integument begins to degenerate (Figure ) and the outer integument produces several distinct cell layers and establishes the “typical” seed coat structure. The outer integument cells elongate and differentiate to the macrosclereid cells, characteristic for the testa of Fabaceae, with phenolic compounds that give a green color by toluidine blue (Figure ). The macrosclereids are large with a well-developed light line in the outer wall and thick cuticle (Figures ).
In seed coat a sub-epidermal layer of cells is differentiated into osteosclereid layer with large intercellular spaces (Figure ). Beneath these are thin-walled parenchymatous cell layers. Parenchyma cells are the innermost part of the seed coat with the inner layer in direct contact with the endosperm. This layer of endosperm (aleuronic layer) remained attached to the parenchyma layer (Figures ). Lipid reserves were determined in endosperm, aleuronic layer and cotyledons; by staining with Sudan black B which reacts positively for lipids (Figure ).
Starch grains are absent in macrosclereids but there are high accumulation of these grains in osteosclereids, and parenchyma cells by staining with lugol (Figures ). Cotyledons accumulate starch (Figure ).
At the hill pole, the Faboideae seed characteristic structure develops, with double palisade layer, subhilar parenchyma, and tracheid bar (Figures ). This strip of large, pitted and lignified tracheids is commonly found in legumes.
Thus, we can show several compound and different layers with different staining beside hematoxylin techniques.
Discussion
Comparative embryology has been an important factor in revealing taxonomic relationships of taxa at higher levels (Johri et al. Citation1992). Comparative embryology of our present, previous results on Onobrychis and literature for Fabaceae are summarized in Table . O. persica shares a number of embryological characters with other species of Onobrychis and Fabaceae (): tetrasporangiate anthers, fibrous endothecium, secretory tapetum, two-celled mature pollens, tricolpate pollen, Polygonum type embryo sac, crassinucellate, bitegmic and anatropous ovule, chalazal functional megaspore and number of layers in inner integument.
Table 1. Embryological comparison among five species of Onobrychis and Fabaceae.
In O. persica the mature embryo-sac has a Polygonum type of development that is common in this family (Riahi et al. Citation2003; Moço and Mariath Citation2003; Galati et al. Citation2006; Rezanejad Citation2007; Rodriguez-Pontes Citation2008; Bakar Büyükkartal Citation2009). This type is the most common in angiosperms and is considered as the primitive embryological character (Liu et al. Citation2003).
The ovules are crassinucellate in Fabaceae (Faigo´n-Soverna et al. Citation2003; Riahi et al. Citation2003; Moço and Mariath Citation2003; Galati et al. Citation2006; Rezanejad Citation2006), which were also found in O. persica. Asymmetrical growth at the funicular region is the reason for this anatropous curvature in O. persica, like other legumes (Bouman and Boesewinkel Citation1991; Galati et al. Citation2006; Rezanejad Citation2006; Riahi and Zarre Citation2009) and our study indicates that the young ovule is hemianatropous and then it change to anatropous one.
The present study indicates that the chalazal megaspore is functional like other species of Onobrychis that we studied before (Chehregani et al. Citation2011) and Vigna unguiculata L. Walp. (Salinas-Gamboa et al. Citation2016). But variations in the position of the functional megaspore have been reported in other Fabaceae, e.g. Trifolium repens (Martin Citation1914) and Vicia faba L. (Mitchell Citation1975) with an epichalazal position in Milletia ovalifolia Kurz (Pal Citation1960), Trifolium hybridum (Kazimierski and Kazimierski Citation1979) and in some Australian species of the tribe Mirbelieae (Cameron and Prakash Citation1994), where it may be in a chalazal, micropylar or epichalazal position.
In O. persica, the inner integument, as our previous studies on Onobrychis (Chehregani and Tanaomi Citation2010; Chehregani et al. Citation2011) and most Fabaceae, consists of two cell layers (Smith Citation1956; Dnyansagar Citation1957; Hindmarsh Citation1964; Deshpande and Bhasin Citation1976; Rembert Junior Citation1977; Ashrafunnisa and Pullaiah Citation1994, Citation1999; Moço and Mariath Citation2003; Riahi and Zarre Citation2009; Vardar Citation2013). However, in Glycine javanica L., Tephrosia, Clitoria ternata L., Pongamia glabra Vent. (Anantaswamy Citation1951), Teramnus labialis (L.f.) Spreng. (Anantaswamy Citation1953), Psophocarpus tetragonolobus (L.) DC. (Lim and Prakash Citation1994) and also in the species of Cassia (Pantulu Citation1945) it is formed by more than two layers.
Although there is the same developmental pattern in O. persica, other species of Onobrychis, and Fabaceae, comparative study showed several significant embryological features of O. persica that differ from those (Table ). These will be discussed in detail below.
The number of middle layer differs between different species. There is one middle layer, dicotyledonous type, or sometimes two middle layers, basic type, in Fabaceae. In O. persica, there is just one middle layer in the anther wall so the anther wall formation is of dicotyledonous type.
The tapetal secretory cells are regularly uni-nucleate in O. persica, Onobrychis altissima Grossh., Onobrychis melanotricha Bornm., Onobrychis andalanica Bornm. and most of Fabaceae. But in Onobrychis schahuensis Bornm. these cells have one or two nuclei (). Our results indicate that the arrangement of microspores in Onobrychis species and often in Fabaceae is tetrahedral and tetragonal with simultaneous cytokinesis ().
The tetrads of megaspores in O. persica, most legumes and our previous study on Onobrychis except for O. schahuensis are T-shaped () (Chehregani and Majd Citation1992; Faigo´n-Soverna et al. Citation2003; Riahi et al. Citation2003; Chehregani et al. Citation2011). But the tetrad shape in Fabaceae can be different (Moço and Mariath Citation2003; Galati et al. Citation2006; Rodriguez-Pontes Citation2008). Rembert Junior (Citation1966, 1967, 1969a, 1969b, 1971) used the marked variability in the great megaspore tetrads to form phylogenetic hypotheses in Leguminosae.
In the chalazal end of embryo sac the antipodal cells are relatively persistent (). Suspensor morphology is variable in the Fabaceae (Lersten Citation1983). In Phaseolus it attains a club-like shape and contains about 200 cells (Cionini Citation1987), while the suspensors of Senna corymbosa (Rodriguez-Pontes Citation2007) and the subtribe Cassiinae (De-Paula and Oliveira Citation2012) are poorly developed. In the present study the suspensor is composed of one column of cells with its maximal length at the heart-shaped stage of the embryo, and begins to degenerate at the cotyledon stage, like in Astragalus cemerinus and A. ruscifolius (Riahi and Zarre Citation2009). Our results showed that the mature legume contains one seed, but every so often the legume contains two seeds. Typically Onobrychis is identified and separated from the other groups because of its one seed legume (Mabberley Citation1990; Lock Citation2005) and there are numerous seeds in different Fabacean species (Riahi and Zarre Citation2009; Chehregani et al. Citation2011). It is a remarkable finding that we have observed two seeds in legume of O. persica. The outer integument with several cell layers is common in Fabaceae (Galati et al. Citation2006; Rodriguez-Pontes Citation2008; Vardar Citation2013). In O. persica, the outer integument has four or five layers, but in Astragalus cemerinus, A. ruscifolius and Swainsona formosa the outer integument is two or three cell layers thick (Zulkarnain Citation2005; Riahi and Zarre Citation2009).
Differentiation of the integuments first occurs in the inner integument with the formation of the endothelium at the early stages of ovule development. The endothelium is a new limiting layer of the embryo sac in many ovules (Bouman Citation1984). In Phaseolus coccineus L. the endothelium is present until the cotyledon stage (Yeung and Clutter Citation1978), but in O. persica it is distinguishable until heart embryo stage. In Vicia faba the degradation of the inner integument and additional maternal tissues occur during the initial development of the embryo (Johansson and Walles Citation1994). Our results indicate that the outer integument begins to take on the morphology of the mature testa at early stages of development.
In O. persica the development of the large macrosclereid with a well-developed light line and osteosclereid was similar to the development pattern observed in Pisum sativum L. (Harris Citation1984), Glycine max Merr. (Harris Citation1987), Erythrina lysistemon Hutch. (Manning and Van Staden Citation1985), and Trifolium pratense L. (Algan and Bakar Büyükkartal Citation2000). The macrosclereid layer is responsible for water impermeability in Fabaceae seeds (Riahi et al. Citation2003; Rodriguez-Pontes Citation2007, 2008; Muneratto and Souza Citation2013; Varela and Albornoz Citation2013). A layer of parenchyma tissue is present in all studied Fabaceae (Riahi et al. Citation2003; Rodriguez-Pontes Citation2007, 2008; Muneratto and Souza Citation2013; Varela and Albornoz Citation2013).
The storage of lipid and starch grains during embryogenesis can be illustrated with different staining techniques. In some species, starch is ephemeral in the seeds, and is a temporary form of reserve. In Glycine max these grains were not observed in endosperm (Chamberlin et al. Citation1994). Morphological characteristics of seeds are often used in taxonomical classifications in Fabaceae (Riahi and Zarre Citation2009; De-Paula and Oliveira Citation2012; Güneş Citation2013). Also with different staining we can recognize valuable characters in seeds that can be very useful in taxonomical analysis, such as the number of layers, presence or absence of some layers, thickness of layers and many different compounds in each layer. We can also study different developmental stages of each seed layers with this specific staining.
Based the embryological data () we can conclude that O. persica has the most similarity with O. altissima. These two species are placed in O. section and have a chromosome number of x = 7 (Hesamzadeh Hejazi and Ziaei Nasab Citation2010; Ranjbar et al. Citation2010; Arslan et al. Citation2012). These embryological findings confirm the position of the species based on morphological and karyological analysis. Our embryological findings indicated that O. persica is different from O. melanotricha and O. andalanica (from Heliobrychis section) in terms of seed number and number of layers in outer integument. According to , embryological characters of O. persica is most different to O. schahuensis, from Hymenobrychis section: in terms of number of tapetal cell nuclei, curvature of ovules, megaspore tetrads, seed number, amyloplast accumulation in embryo sac and ovary peduncle, and hair. In conclusion, our embryological findings are in agreement with the taxonomical positions of these species.
Funding information
This research was party funded by a grant provided by the research councils of Kharazmi University and Bu–Ali Sina University.
Disclosure statement
No potential conflict of interest was reported by the authors.
References
- Ahangarian S, Kazempour Osaloo S, Maassoumi AA. 2007. Molecular phylogeny of the tribe Hedysareae with special reference to Onobrychis (Fabaceae) as inferred from nrDNA ITS sequences. Iran J Bot. 13 (5):64–74.
- Aktoklu E. 2001. Two new varieties and a new record in Onobrychis from Turkey. Turk J Bot. 25(5):359–363.
- Algan G, Bakar Büyükkartal HN. 2000. Ultrastructure of seed coat development in the natural tetraploid Trifolium pretense L. J Agron Crop Sci. 184:205–213.
- Anantaswamy RM. 1951. The endosperm in some of the Papilionaceae. Phytomorphology. 1:153–158.
- Anantaswamy RM. 1953. Some observations on the endosperm in Papilionaceae. Phytomorphology. 3:209–222.
- Arslan E, Ertuğrul K. 2010. Genetic relationships of the genera Onobrychis, Hedysarum and Sartoria using seed storage proteins. Turk J Bot. 34:67–73.
- Arslan E, Ertuğrul K, Tugay O, Dural H. 2012. Karyological studies of the genus Onobrychis Mill. and related genera Hedysarum L. and Sartoria Boiss. & Heldr. (Fabaceae, Hedysareae) from Turkey. Caryologia. 65(1):11–17.
- Ashrafunnisa A, Pullaiah T. 1994. Embryology of Galactia (Fabaceae). Phytomorphology. 44:253–260.
- Ashrafunnisa A, Pullaiah T. 1999. Embryology of Teramnus labialis (Fabaceae). Phytomorphology. 49:192–202.
- Bakar Büyükkartal HN. 2009. Ultrastructural changes of the egg apparatus associated with fertilization of natural tetraploid Trifolium pratense L. (Fabaceae). Biol Res. 42:25–30.
- Boissier E. 1872. Onobrychis. In: Boissier E, editor. Flora Orientalis. vol 2. Geneva: Basilease and Lugundi; p. 545–553.
- Bouman F. 1984. The ovule. In: Johri BM, editor. Embryology of Angiosperms. Berlin: Springer-Verlag; p. 123–157.
- Bouman F, Boesewinkel FD. 1991. The campylotropous ovules and seed, their structure and functions. Bot Jahrbüch Syst. 113:255–270.
- Cameron BG, Prakash N. 1994. Variations of the megagametophyte in the Papilionoideae. In: Ferguson IK, Tucker S, editors. Advances in legume systematics 6: structural botany. Royal Botanic Gardens: Kew. p .97–115.
- Chamberlin MA, Horner TH, Palmer RG. 1994. Early endosperm, embryo and ovule development in Glycine max (L.) Merr. Int J Plant Sci. 155:421–439.
- Chehregani A, Majd A. 1992. Studies on developmental process in ovules of Soja Glycine max L. plants and effects of certain toxins and environmental pollutants. Acta Horticult. 319:431–436.
- Chehregani A, Mohsenzadeh F, Tanaomi N. 2011. Comparative study of gametophyte development in the some species of the genus Onobrychis: Systematic significance of gametophyte futures. Biologia. 66(2):229–237.
- Chehregani A, Tanaomi N. 2010. Ovule ontogenesis and megagametophyte development in Onobrychis schahuensis Bornm. (Fabaceae). Turk J Bot. 34:241–248.
- Chehregani A, Tanaomi N, Ranjbar M. 2008. Pollen and anther Development in Onobrychis schahuensis Bornm. (Fabaceae). International Journal of Botany. 4(2):241–244.
- Cionini PG. 1987. The suspensor and its role in embryo development in Phaseolus (Papilionaceae): a review. Atti Soc Toscana Sci Natur Pisa. 94:151–161.
- De-Paula OC, Oliveira DMT. 2012. Seed ontogeny of Chamaecrista and its systematic implications in Cassiinae (Leguminosae, Caesalpinioideae). Plant Syst Evol. 298(9):1659–1669.
- Deshpande PK, Bhasin RK. 1976. A contribution to the life history of Zornia diphylla Pers. J Ind Bot Soc. 55:115–124.
- Dnyansagar VR. 1957. Embryological studies in the Leguminosae. V. Prosopis spicigera and Desmanthus virgatus. Bot Gaz. 118 (3):180–186.
- Duan L, Jun Wen J, Yang X, Liu PL, Arslan E, Ertuğrul K, Chang ZY. 2015. Phylogeny of Hedysarum and tribe Hedysareae (Leguminosae: Papilionoideae) inferred from sequence data of ITS, matK, trnL-F and psbA-trnH. Taxon. 64 (1):49–64.
- Faigo´n-Soverna A, Galati B, Hoc P. 2003. Study of ovule and megagametophyte development in four species of subtribe Phaseolinae (Leguminosae). Acta Biol Cracov Ser Bot. 45:57–67.
- Feng X, Zhao Z, Tian Z, Xu S, Luo Y, Cai Z, Wang Y, Yang J, Wang Z, Weng L, Chen J, Zheng L, Zheng L, Guo X, Luo J, Sato S, Tabata S, Ma W, Cao X, Hu X, Sun C, Luo D. 2006. Control of petal shape and floral zygomorphy in Lotus japonicas. Proceedings of the National Academy of Siences. 103(13):4970–4975.
- Fisher DB. 1968. Protein staining of ribboned epon sections for light microscopy. Histochemie. 16 (1):92–96.
- Gahan PB. 1984. Plant histochemistry and cytochemistry. London: Academic Press.
- Galati BG, Rosenfeldt S, Toum GM. 2006. Embryological studies in Lotus glaber (Fabaceae). Ann Bot Fenn. 43:97–106.
- Ghassempour H, Majd A. 2012. Ovule ontogenesis and megagametophyte development in Onobrychis sintenissii Bormn.(Leguminosae–Papilionoideae). J Appl Biol Sci. 6(1):51–54.
- Gordon EM, McCandless EL. 1973. Ultrastructure and histochemistry of Chondrus crispus Stackhouse. In: Harvey MJ, Mclachlan J, editors. Chondrus crispus. Halifax: Nova Scotian Institute of Science; p. 111–133.
- Güneş F. 2013. Seed characteristics and testa textures of Pratensis, Orobon, Lathyrus, Orobastrum and Cicercula sections from Lathyrus (Fabaceae) in Turkey. Plant Syst Evol. 299:1935–1953.
- Harris WM. 1984. On the development of osteosclereids in seed coats of Pisum sativum L. New Phytol. 98:135–141.
- Harris WM. 1987. Comparative ultrastructure of developing seed coats of hard-seeded and soft-seeded varieties of soybean, Glycine max (L.) Merr. Bot Gaze. 148:324–331.
- Hashemi Z, Rezanejad F. 2013. Morphological and developmental study of Alhagi pseudoalhagi (M. B.) Desv. flower and anatomical features. Journal of. Plant Biology. 15(5):31–43.
- Hesamzadeh Hejazi SM, Ziaei Nasab M. 2010. Cytotaxonomy of some Onobrychis (Fabaceae) species and populations in Iran. Caryologia. 63 (1):18–31.
- Hindmarsh GJ. 1964. Gametophyte development in Trifolium pratense L. Aust J Bot. 12:1–14.
- Johansen DA. 1940. Plant microtechniques. 2nd ed. New York (NY): McGraw Hill.
- Johansson M, Walles B. 1994. Functional anatomy of the ovule in broad bean (Vicia faba L.): Ultrastructural seed development and nutrient pathways. Ann Bot. 74:233–244.
- Johri BM, Ambegaokar KB, Srivastava PS. 1992. Comparative embryology of Angiosperms. vol 1. New York (NY): Springer-Verlag.
- Karamian R, Ranjbar M, Hadadi A. 2012. Chromosome number reports in five Onobrychis species (O. sect. Onobrychis, Fabaceae) in Iran. J Cell Mol Res. 3(2):81–92.
- Kazimierski T, Kazimierski EM. 1979. Structure of embryo sac, fertilization and development of embryo in Swedish clover (Trifolium hybridum L.) plants with reduced leaves. Acta Soc Bot Polon. 48:365–375.
- Lersten NR. 1983. Suspensors in Leguminosae. Bot Rev. 49:233–257.
- Lewke Bandara N, Papini A, Mostl S, Brown T, Smith LMJ. 2013. A phylogenetic analysis of genus Onobrychis and its relationships within the tribe Hedysareae (Fabaceae). Turk J Bot. 37:981–992.
- Lim AL, Prakash N. 1994. Embryology and seed development in the winged bean. Psophocarpus tetragonolobus. Garden Bull. 46 (2):79–92.
- Liu ZW, Xiao DX, Zhang L, Lian FQ, Tu SP. 2003. Microsporogenesis and development of male gametophyte in Lycoris radiate Herb. Acta Agric Univ Jiangxiensis. 28:234–238.
- Lock JM. 2005. Tribe Hedysareae. In: Lewis G, Schrire B, Mackinder B, Lock M, editors. Legumes of the World. Kew: Royal Botanical Gardens; p. 489–495.
- Mabberley DJ. 1990. The plant book, a portable dictionary of the higher plants, reprinted with corrections. Cambridge: Cambridge University Press.
- Manning JC, Van Staden J. 1985. The development and ultrastructure of the testa and tracheid bar in Erythrina lysistemon Hutch. (Leguminosae: Papilionoideae). Protoplasma. 129:157–167.
- Martin JN. 1914. Comparative morphology of some Leguminosae. Bot Gaz. 58 (2):154–167.
- Mitchell JP. 1975. Megasporogenesis and microsporogenesis in Vicia faba. Can J Bot. 53:2804–2812.
- Moço MCC, Mariath JEA. 2003. Ovule ontogenesis and megasporogenesis in Adesmia latifolia (Spreng.) Vog. (Leguminosae–Papilionoideae). Rev Brasil Bot. 26:495–502.
- Muneratto JC, Souza LA. 2013. Fruit (pericarp and seed) ontogeny of Sida species. Gayana Bot. 70(1):44–56.
- O’Brien TP, Feder N, Mccully ME. 1964. Polychromatic staining of plant cell wall by toluidine blue. Protoplasma. 59 (2):39–52.
- Pal N. 1960. Development of the seed of Milletia ovalifolia. Bot Gaz. 122:130–137.
- Pantulu JV. 1945. Studies in the Caesalpinioideae. I. A contribution to the embryology of the genus Cassia. J Indian Bot Soc. 24:10–24.
- Pavlova DK, Manova VI. 2000. Pollen morphology of the genera Onobrychis and Hedysarum (Hedysareae, Fabaceae) in Bulgaria. Annales Botanici Fennici. 37(3):207–217.
- Ranjbar M, Hajmoradi F, Karamian R. 2012. An overview on cytogenetics of the genus Onobrychis (Fabaceae) with special reference to O. sect. Hymenobrychis from Iran. Caryologia. 65 (3):187–198.
- Ranjbar M, Karamian R, Hadadi A. 2010. Cytosystematics of three Onobrychis species (Fabaceae) in Iran. Caryologia. 63 (3):237–249.
- Rechinger KH. 1984. Onobrychis. In: Rechinger KH, editor. Flora Iranica. Graz, Austria: Akademische Druck- und Verlagsantalt. 157:387–464.
- Rembert Junior DH. 1966. Megasporogenesis in Laburnum anagyroides Medic. a case of bisporic development in Leguminosae. Trans Kentucky Acad Sci. 27:47–50.
- Rembert Junior DH. 1967. Development of the ovule and megagametophyte in Wisteria sinensis. Bot Gaz. 128:223–229.
- Rembert Junior DH. 1969a. Comparative megasporogenesis in Caesalpiniaceae. Bot Gaz. 130:47–52.
- Rembert Junior DH. 1969b. Comparative megasporogenesis in Papilionaceae. Am J Bot. 56:584–591.
- Rembert Junior DH. 1971. Phylogenetic significance of megaspore tetrad patterns in Leguminales. Phytomorphology. 21:317–416.
- Rembert Junior DH. 1977. Contribution to ovule ontogeny in Glycine max. Phytomorphology. 27:368–370.
- Rezanejad F. 2006. Zygotic Embryogenesis in Spartium junceum L. (Fabaceae): Development of embryo and suspensor. Int J Bot. 2(2):113–16.
- Rezanejad F. 2007. The effect of air pollution on Microsporogenesis in Spartium Junceum L. (Fabaceae). Turk J Bot. 31:183–191.
- Riahi M, Zarre S. 2009. Seed development in Astragalus cemerinus and A. ruscifolius (Fabaceae), and its systematic implications. Acta Biol Cracov. 51:111–117.
- Riahi M, Zarre S, Chehregani A, Shahsavan-Behboudi B. 2003. Seed development in two species of medifixed hairy Astragalus (Fabaceae). Flora. 198:211–219.
- Rodriguez-Pontes M. 2007. Development of megagametophyte, embryo, and seed in Senna corymbosa (Lam.) H.S. Irwin & Barneby (Leguminosae – Caesalpinioideae). Bot J Linn Soc. 153(2):169–179.
- Rodriguez-Pontes M. 2008. Seed formation in two species of Adesmia (Fabaceae): co-occurrence of micropylar and lateral endosperm haustoria in legumes and its taxonomic value. Bot J Linn Soc. 158:602–612.
- Salinas-Gamboa R, Johnson SD, Sánchez-León N, Koltunow AMG, Vielle-Calzada J-P. 2016. New observations on gametogenic development and reproductive experimental tools to support seed yield improvement in cowpea [Vigna unguiculata (L.) Walp.]. Plant Reprod.doi:10.1007/s00497-015-0273-3.
- Schischkin BK, Bobrov EG. 1972. Flora of USRR. Vol. 13. Jerusalem: Israel Program for Scientific Translations; p. 244–281.
- Smith BW. 1956. Arachis hypogea: normal megasporogenesis and syngamia with occasional single fertilization. Am J Bot. 43:81–89.
- Suzuki K, Takeda H, Tsukaguchi T, Egawa Y. 2001. Ultastructural study on degeneration of tapetum in anther of Snap bean (Phaseolus vulgaris L.) under heat stress. Sexual Plant Reproduction. 13:293–299.
- Teixera SP, Forni–Martins ER, Ranga NT. 2002. Development and cytology of pollen in Dahlstedtia Malme (Leguminosae: Papilionoideae). Botanical Journal of the Linnean Society. 138(4):461–471.
- Vardar F. 2013. Developmental and cytochemical features of female gametophyte in endemic Lathyrus undulatus (Fabaceae). Int J Agric Biol. 15 (1):135–139.
- Varela RO, Albornoz PL. 2013. Morpho-anatomy, imbibition, viability and germination of the seed of Anadenanthera colubrina var. cebil (Fabaceae). Rev Biol Trop. 61(3):1109–1118.
- Wilson CA. 2001. Floral stages, ovule development, and ovule and fruit success in Iris tenax, focusing on var. Gormanii, a taxon with low seed set. American Journal of Botany. 88(2):2221–2231.
- Yeung EC, Clutter ME. 1978. Embryogeny of Phaseolus coccineus: growth and microanatomy. Protoplasma. 94:19–40.
- Zulkarnain Z. 2005. Embryology of Swainsona formosa (Fabaceae): anther and ovule development. Hayati J Biosci. 12 (1):11–16.