Abstract
The histological structure of the anthers and developmental processes of the male and female gametophytes in Ocimum basilicum L. were studied for the first time. To study developmental stages of the flower, young flowers and buds at different developmental stages were embedded in paraffin, sliced with a microtome and analyzed using a photomicroscope. It was observed that sporogenous meristem is formed as a result of cellular divisions in tunica and corpus regions. The initial ring (IR) activities remarkably decreased. Successive divisions in the peripheral and surface regions of sporogenous meristem results in the stamens primordia. Petals were grown from the peripheral parts of the region. Stamens were tetrasporangiate and cytokinesis in microsporocyte is simultaneous. The tetrads were also tetrahedral and the tapetum is of the secretory type. The mature pollen grain was three-celled and composed of a vegetative cell and two sperms. The ovary in O. basilicum was observed to be hypogynous, quadri-locular with several ovules in each loculus. The ovules were anatropous, unitegmic, and tenuinucellate. Finally, the embryo sac development belonged to the Polygonum type.
Keywords:
1. Introduction
Ocimum basilicum L., popularly known as sweet basil, is an annual aromatic plant native to tropical and warm regions and is scattered throughout the six continents of the world (Joshi Citation2014). The genus Ocimum (Lamiaceae) consists of about 30 species scattered in the tropical and subtropical regions of the world with some species cultivated in temperate areas (CitationPaton 1992). Svecova and Neugebauerova (Citation2010) reported a study on 34 cultivars of basil (Ocimum basilicum L.) and their morphological, economic, and biochemical characteristics using standardized descriptors.
The essential oil in O. basilicum is mainly composed of monoterpenes, sesquiterpenes, and phenyl propanoids (Raina et al. Citation2013). These secondary metabolites play a role in chemical defenses of the plant against abiotic and biotic stress, such as infection caused by viruses (Ramegowda and Senthil-Kumar Citation2015). The oil content and ornamental value of a wide range of species and cultivars of O. basilicum from various different sources was evaluated using standardized descriptors (Joshi Citation2014).
A distinctive morphological feature of this genus is the rectangular cross-section of the stem and opposite leaves. The flowers are strongly zygomorphic with two distinct lobes. There are four stamens and those located at the anterior lobe are pseudostamens. Anterior stamens were located on corolla and the quadric-locular ovary is hypogenous (Paton Citation1992). The pollen morphology in Lamiaceae was studied by Moon and Hong (Citation2003). They reported that the pollen is mostly medium or sometimes small in size. The exine is bireticulate with unbranched collumellae and a continuous, lamellated endexine (Moon and Hong Citation2003). Waterman (Citation1960) studied Labiatae pollen grains ranging from 25–35 μm in length; these are prolate to sub-spheroidal. They are reticulate, and either tricolpate or hexacolpate.
Morphological and systematic analysis of O. basilicum has long been an important issue (Raj Citation1983) and many researchers have been interested in transgenic studies on this genus (Oram and Kirk Citation1992). The diversity of chemical compounds in the plant has also been studied (Krüger et al. Citation2002). Majd et al. (Citation2014) studied and compared the essential oil of two Origanum species: Origanum majorana L. and O. vulgare L., to determine their composition. They showed that general differentiation between the two species was related to the amount of component groups and their most important essential oil content. Among species of Ocimum, O. basilicum is usually considered as the most important species from an economic point of view. The essential oil extracted from O. basilicum has shown antioxidant and antibacterial properties (Bentham Citation1848; Javanmardi et al. Citation2003; Sirousmehr et al. Citation2014). The extracts contain aromatic substances with a wide range of biological applications and properties: insect repellent, nematocide, fungicide, and antioxidant (Cao et al. Citation2008; Javanmardi et al. Citation2003; Juliani and Simon Citation2002).
Several researchers have studied the development of male and female gametophytes of the Lamiaceae family. Daskalova and Genova (Citation1996) and Daskalova (Citation2004) reported most of the established embryological features: tetrasporangiate and four-layered anthers, tapetum of the secretory type, normal meiosis of the simultaneous type, and three-celled hexacolpate pollen are typical of the species of Lamiaceae studied so far (Daskalova Citation2004; Daskalova and Genova Citation1996). Daskalova (Citation2005) have shown that each anther is constituted of four layers and each undergoes further development until their distinct morphological differentiation during the development of the male gametophyte. Finally, prior to splitting of the anther, only two layers remained: a fibrous endothecium and epidermis. Biochemical and morphological properties of this plant have also been studied (Svecova and Neugebauerova Citation2010). Mohammed and Tesfaye (Citation2015) have carried out the analysis of molecular variance for the accessions studied and showed that the highest proportion of genetic variation was attributed to within populations rather than among populations. This confirms that there is a high level of gene flow and low level of genetic differentiation. Mačukanović-Jocić et al. (Citation2007) used SEM (scanning electron microscope) investigation, and serial sectioning demonstrated that the floral nectaries are slightly convex and asymmetrically four-lobed, with three large lobes facing the lower corolla lip and the smallest facing the upper corolla lip in Ocimum. They studied floral nectaries of O. basilicum using light, fluorescence and scanning electron microscopy. Each nectary forms an asymmetrical four-lobed disc at the base of the outer surface of the ovary. The three large lobes were found to be functional, whereas the smallest lobe is lacking in the modified stomata. The nectary epidermis of three functional lobes is covered with a very thin cuticle and contains many modified stomata involved in the exudation process. They are diffusely distributed and lie in the same plane as the epidermal cells. The secretory tissue is composed of small cells with thin walls, relatively large nuclei, dense granular cytoplasm and small vacuoles (Mačukanović-Jocić et al. Citation2007).
Since O. basilicum is of pharmaceutical importance and its compounds and essential oils are widely used in food and perfume industries, the present study examines micro-sporogenesis, micro-gametogenesis, macro-sporogenesis and macro-gametogenesis in O. basilicum. Based on our studies of the literature, this is the first study reporting on the sporogenesis and gametophyte development of O. basilicum.
2. Materials and methods
Basil seeds were obtained from Pakan Bazr Institute, Isfahan (Iran). Plastic pots of 18 cm height and 20 cm diameter were used for sowing the seeds during two successive summers. The flowers and buds were harvested at different developmental stages, fixed in FAA70 (formalin, glacial acetic acid and 70% ethanol, 5:5:90 v/v), and then stored in ethanol 70%. Specimens were dehydrated by passing them through solutions of increasing ethanol concentration. After embedding the samples in paraffin wax, they were sliced (7–10 μm) with a Micro DC 4055 microtome (Cut 4060 Co., Mainz, Germany). Staining was carried out with hematoxylin and eosin according to the protocol suggested by Yeung (Citation1984). Several slices were studied for each developmental stage using a light microscope (Nikon, Tokyo, Japan) equipped with a digital camera. At least 20 specimens were studied for each developmental stage and the best were taken for photomicrographs (Yeung Citation1984).
3. Results
3.1. Generative meristem and flowering
Microscopic observation of generative meristem showed that sporogenous meristem is formed as a result of cellular divisions in tunica and corpus regions (Figure (a)). The initial ring (IR) activities remarkably decreased and also there was a reduction in the size of this region (Figure (a)). The remaining part of this region turned into primordia of sepals after successive divisions (P.Se in Figure (a)). Petal primordia were grown from the peripheral parts of this region (P.Pe in Figure (b)). Since inflorescence in basil is in the form of verticillaster, a floret is observed in each shoot where each of the florets is developing into a fully grown flower. The lower florets (Fl.) were at more advanced stages of development (Figure (c)). As this figure shows, generative meristem lengthens as a result of cellular divisions and its cells increase in volume, vacuolating later.
Figure 1. Developmental stages of reproductive meristem in basil stained with hematoxylin-eosin (×10). (a) Reproductive meristem at initial formation stage: the lump of sporogenous meristem is noticeable and receptacle meristem is active at the bottom. Procambial strands were also seen. (b) This is a more developed stage of reproductive meristem. Two lumps emerge on sporogenous meristem forming petals primordia at the edges while turning into ovary primordium in the middle. (c) Verticillaster inflorescence. (d) Stamens, sepals, and petals primordia are clearly observed; however, ovary primordium is still flat. (e) Components of the flower are gradually emerging as cell division continues. (f) Pistil primordium is seen at the center. (g, h) A very young bud. (i, j) A bud with large mature anther and the ovary containing ovule. (j) Fully grown flower and wide open anther. Abbreviations: An: anther; Br: bract; Fl.: floret; IR: initial ring; O: ovule; Ov: ovary; P.o: primordium ovule; P.Pe: primordium petal; P.Pi: primordium pistil; P.S.: procambium strand; P.Se: primordium sepal; P.St: primordium stamen; Pe: petal; R.M.: receptacle meristem; Se.: sepal; Sp.M.: sporogenous meristem; Sti: stigma; Sty: style.
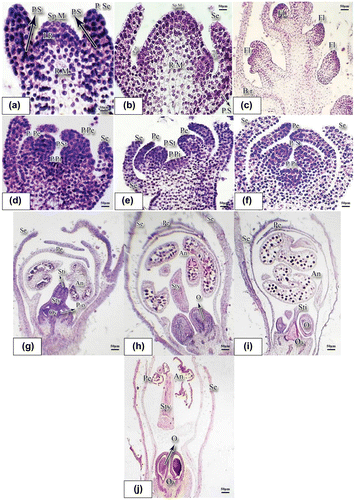
Successive divisions in the peripheral and surface regions of sporogenous meristem results in the stamens primordia (P.St in Figure (d)). After successive divisions in the extreme peripheral regions of the sporogenous meristem, primordia of petals (P.Pe) were founded while the central region remains as a lump for generation of pistil primordium (P.Pi in Figure (d)). Sepals were developed in the peripheral regions as a result of divisions in the remaining parts of the initial ring (Figure (d)). Petals were connected to primordial of stamens (Figure (d)), but were separated at later stages (Figure (e), (f)). At late developmental stages of generative meristem, it produces pistil primordium (P.Pi.) at the central part of the sporogenous meristem (Figure (e), (f)). It also paves the way for the emergence and development of pistil primordium as a result of cellular divisions at central and also deeper regions. Emergence and gradual development of the flower components were shown in Figure (g–j). As the cell divisions progress, components of the flower formed (Figure (g–j)).
Figure 2. Developmental stages of stamens and pollen grain in basil stained with hematoxylin-eosin. (a) Longitudinal incision of a young anther with the microspore mother cells inside (×40). (b) Microspore mother cells; the arrow shows tapetum binucleate cells. The top layer cells have progressed towards the anther sac and they were marked with a circle (×100). (c) Longitudinal incision of the anther at dyad microspore stage (×40). (d) Four layers composing the anther wall: epiderm, endothecium, middle layer and the tapetum; tetrad is clearly noticeable (×40). (e) Tetrahedral tetrad (×40). (f) Young microspore produced through meiosis and released from the tetrad; the two nuclei were noticeable (×100). Abbreviations: En: endothecium; Epi: epidermis; Ex: exine; In: intine; Mi: middle layer; M.M.C.: microspore mother cell; S.W.: special wall; Ta.: tapetum.
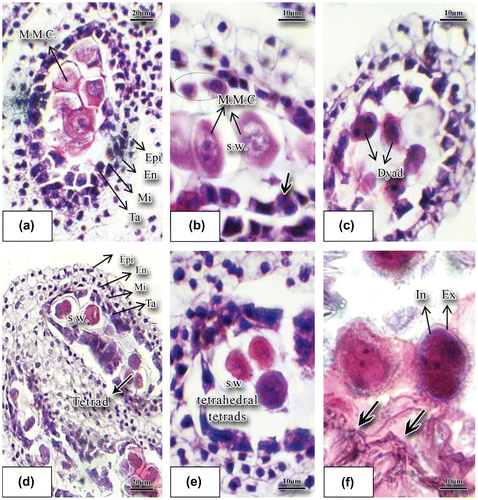
The intensity of cellular divisions gradually increases from the outer to the inner ring which is evident from staining and volume of the cell nucleus (Figure (f)). As this figure showed, the cells dividing to form pistil primordium were deeper in color at the deeper layers, suggesting more intensive cellular division at this region to form pistil and its components.
As the cells divide, microspore mother cells were produced in anther. Style, ovary, and primordia of ovules were formed in the gynoecium. In buds (Figure (g–i)), as developmental stages progress and components of flower were formed, ovary becomes bulky, bilobate style and stigma become visible, ovules are formed inside the ovary and tetrasporangiate anthers are clearly observed while stamens are not dehiscent yet (Figure (g)). In Figure (j), while sepals were open and petals have surrounded flower components, stamens are too bulky and maturing pollen grains are noticeable. Ovaries contain immature ovules and the style is visible as a long column in the middle part of the bud. Petals in Figure (j) are away from each other and the internal parts of the flower are clearly seen, the anther is complete, the mature pollens are released from the anther and the development of flower components is complete (Figure (i), (j)).
3.2. Microsporogenesis and development of pollen grains
The stamen in basil develops very early; when the lump of the ovary primordium is still not clear, the primordium of stamen is noticeable as a mass of meristemic cells. There are four stamens with connected anthers. Tetrasporangiate anthers contain four pollen sacs (Figure (a)) and at the early stages of development of anthers several rows of archeosporial cells were differentiated beneath the epidermis of the anther. Each archeospore cell divides into two cells, a peripheral cell and a sporogenous cell. The peripheral cell generates layers of anther wall and the sporogenous cell generates microspore mother cells (MMC). At this stage microspore mother cells were well differentiated and large in size with high density of cytoplasm. Each microspore mother cell increases in size and forms a special wall of callosic nature (Figure (b–d)). This callosic wall shows that meiosis division is imminent. Meiosis causes the formation of tetrahedral tetrads that are surrounded with a special callosic wall (Figure (e)). The young anther wall in this sample is composed of four layers, namely: epidermis, endothecium layer, middle layer, and the innermost tapetum layer (secretory) (Ta in Figure (a)). The middle layer in sweet basil is composed of narrow cells. The middle layer consisting of small-sized cells is not ephemeral and remains vital up to the end of homeotypic division during meiosis in the MMC (Figure (a)). Tapetum cells make a distinct layer and surround microspore mother cells. Layers of the anther wall, particularly the tapetum layer, become distinct in the proximity of microspore mother cells. Initially, the tapetum is secretory (Figure (f)) consisting of a one-nucleate, large, polygonal or rectangular cell. Occasionally, the tapetum cells are morphologically unequal. During anther ontogenesis and after subsequent mitoses they become binucleate (thick arrow in Figure (b)). Usually, the cells of the inner tapetum (towards the periphery of the anther wall) are different in size, shape, and speed of their development as compared to those of the outer integument towards the cavity of the anther locule. After the formation of dyad in the young anthers (Figure (c)), finally meiosis divisions lead to tetrahedral cells (Figure (d), (e)). The callosic layer degenerates. The cells forming tetrads separate and release microspores which do not have any vacuole yet and have dense cytoplasm and regular form (Figure (f)). As per the figure, the microspore nucleus immediately starts dividing and the young microspore becomes binucleated. The pollen sac contains developing microspores. Formation of exine and intine and degeneration of tapetum layer are visible (thick arrows in Figure (f)). The tapetum layer is of the secretory type at this stage (thick arrows in Figure (f)). Exine and intine layers in the young microspores are noticeable (Figure (f)). The two nuclei that compose microspores are morphologically quite distinct and have different functions (Figure (b)). The generative nucleus (GN) has tightly packed DNA content and is darker while the vegetative nucleus (VN) has less dense DNA content and is lighter in color (Figure (b)). The large vegetative cell and a small generative nucleus result in the formation of a mature pollen grain. The mature pollen grain in this species was composed of a vegetative cell and two sperms (thick arrows in (Figure (d)). Tapetum layer cells almost degenerate at binuclear pollen grain stage. Sculpturing of mature pollen grains is reticulated with horizontal lines (Figure (c)).
Figure 3. Mature pollen grain and anther stained with hematoxylin-eosin. (a) Cross section of the mature anther (×4). (b) The generative and vegetative nuclei have distanced from each other; the longitudinal slice of the anther shows the binucleate pollen grain (×100). (c) Wide open anther inside which pollen grains have exine ornament (×40). (d) Mature pollen grain contains a large vegetative and generative cell (×100); the generative cell has two sperms (arrows) (×100); the mature pollen grain is surrounded by exine and intine. Abbreviations: An: anther; Con: connective; En: endothecium; Ex: exine; Fi: filament; Sty: style; G.N.: generative nucleus; In: intine); Po: pollen grain; Sty: style; V.N.: vegetative nucleus.
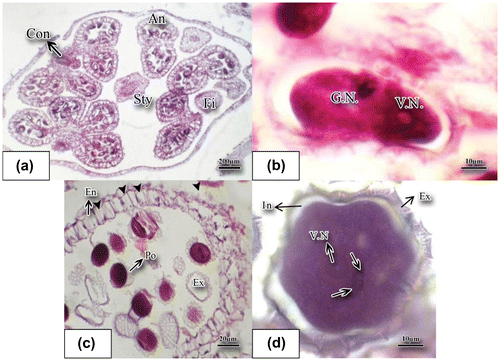
Figure 4. Development of ovule and megasporogenesis in O. basilicum stained with hematoxylin-eosin. (a) Longitudinal slice of a very young ovule; archeospore cells are noticeable (×100). (b) The young ovule which is anatropous at this stage (×40). (c) Longitudinal slice of the young ovule producing megaspore mother cell is observable in this figure (×100). (d) Four nucleate embryo sac (×40). (e) Binucleate embryo sac (×40). Abbreviations: Ar.: archeospore cell; E.N.: endothelium; F.: funicule; E.S.: embryo sac; M.M.C.: megaspore mother cell; Mi: micropyle; N: nucellus; T: tegument.
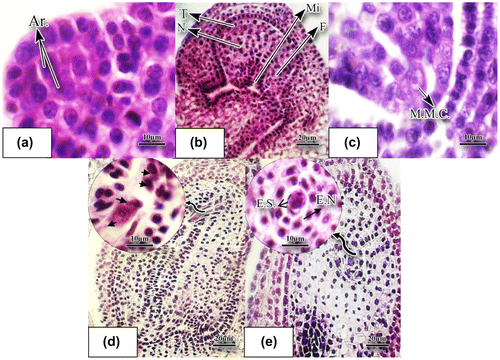
The microspore cells released from the callosic coat were surrounded by the exine wall. Another pecto-cellulose wall called the intine is formed near the cytoplasm which is thicker in the grooves. Released pollen grains in this plant are nearly spherical and their maturation is complete with formation of the exine sculpturing and small spikes (Figure (d)). At this stage, the remaining layer of anther is the endothecium and the other layers fade away (Figure (c)). Upon tearing up the endothecium, mature pollen grains were released. The fibrous thickness of endothecium was shown with black arrowhead (Figure (c)).
3.3. Development of gynoecium and ovary
The gynoecium in O. basilicum consists of two carpels, a long style, and bilobated stigma. The ovules are anatropous, unitegumic, and tenuinucellate (Figures (j), (b)). The ovular primordium was initiated by periclinal cell division of the ovary wall (Figure (g)). After development of stamens primordia, the meristem divides in order to form primordium of gynoecium. The primordium of gynoecium divides rapidly after formation of the stamens primordia. During the stages when microspore mother cells are developing inside the anther, primordia of ovules which were composed of six to seven layers were gradually formed through periclinal divisions of ovary wall. Archeospore cells were quite distinguished inside primordia of ovules from the other nucellus cells because of their large volume, dense cytoplasm and voluminous nucleolus (Figure (a)).
Figure 5. Stages of conception and formation of proembryo in basil stained with hematoxylin-eosin. (a) Mature embryo sac (×40). (b) Longitudinal slice of ovule; the oosphere is being conceived inside the embryo sac; hypostasis cells are above (×10). (c) The oosphere is being conceived; male gamete is located in the vicinity of the oosphere (black arrow shows the male gamete) (×100). (d) The two cells at the base are synergids, while the cell above is being fertilized by a sperm (arrowhead). The cell on the top of the triangle is the oosphere cell. The albumen mother cell divides and generates the nuclear albumen (×40). (e) Zygote cell and the nearby albumen mother cell (×100). (f) Conceived embryo sac; proembryo is located on the one row suspensor nuclear albumen. Divisions of albumen nuclei which resulted in the nuclear albumen are noticeable (×40). Abbreviations: A.M.C.: albumen mother cell; Ant: antipodal cells; C.C.: central cell; Hyp: hypostasis; Oo: oospher; P.em: proembryo; Su: suspensor; Syn: synergid; Z.C.: zygote cell.
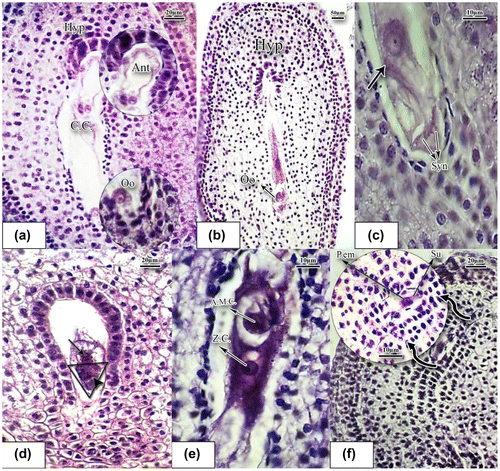
3.4. Development of female gametophyte
The female gametophyte develops over two main phases of megasporogenesis and megagametogenesis. Megasporogenesis involves stages of meiosis divisions. The archesporial cells below the nucellus epidermis form parietal cells and sporogenous cells (Figure (a)). Successive division of primordium of ovule gradually forms the ovule, which is anatropous at this stage and is tunicate (Figure (b)). A sporogenous cell then differentiates into a megasporocyte (Figure (c)). The megasporocyte is characterized by a large nucleus and dense cytoplasm. As the figure shows, the megaspore mother cell in basil is located directly under the ovular epidermis. Meiosis of the megasporocyte produces a linear tetrad of megaspores (Figure (d)). Three out of four cells produced through meiosis degenerate and the remaining cell is a functional megaspore cell (Figure(d)). The megaspore mother cell produces megaspores as a result of meiosis. The functional megaspore also undergoes three successive mitotic divisions without cytokinesis at the first mitotic division, producing two new haploid nuclei making a binucleated embryo sac (Figure (e)). In the course of development of the megaspore, the female gametophyte, or embryo sac, is generated. Each nucleus is relatively voluminous and basophilic. The second mitotic division also produces four haploid nuclei and results in an embryo sac with four nuclei. Finally, the third mitosis results in the embryo sac with eight nuclei, which is in accordance with the Polygonum type of embryo sac. As the embryo sac enlarges length-wise, vacuoles are formed within the eight nuclei and as a result three nuclei remain at the micropyle end while three nuclei reside at the chalaza end and the other two remain at the center (Figure (a)). Thus, it consists of seven cells: two synergid cells and one egg cell (oosphere) comprising the egg apparatus, three antipodal cells, and one central cell that contains two nuclei. Formation of the embryo sac and increase in its volume results in occupation of a large proportion of the mature ovule. The peripheral tissues of nucellus and its base gradually decompose and degenerate and the embryo sac proceeds towards the micropyle. Endothelium cells, because of an increase in the nucleolus volume, were clearly noticed around the embryo sac and particularly around the antipodes. In the ovule, usually after the 2- or 4-nucleated embryo sac stage, a hypostase forms in its chalazal region (Figure (a), (b)). There are three antipode cells in the shape of a crescent at the chalazal pole which are connected to the two central cells through the cytoplasm appendage. The oosphere cell is easily seen at the micropyle pole (Figure (a)). There are two polar (central) nuclei at the center of the embryo sac (Figure (a)). The mature embryo sac is depicted in Figure (b). Thus, there is a cellularization process in the embryo sac resulting in the formation of the mature embryo sac. Cellularization of the embryo sac occurs simultaneously with the migration of nuclei. The three cells in the vicinity of the micropyle make up the egg apparatus (Figure (b), (c)). The antipodal cells were degenerated immediately at prefertilization stages (Figure (e)). The egg apparatus is composed of two triangular synergid cells, one with a bulky nucleolus while the other is degenerating. The pollen tube releases its gametes into the embryo sac (Figure (c)). Immediately after fertilization, in the triangle, three cells can be observed (Figure (d)). The two cells at the base are synergids, while the cell above is being fertilized by a sperm (arrowhead). The cell on the top of the triangle is the oosphere cell. The albumen mother cell divides and generates nuclear albumen. One of the sperms combines with the egg cell and forms the zygote cell. The other gamete combines with the central (polar) cell and form the triploid albumen mother cell (Figure (c–e)) which is a very large cell with high chromosome content remaining in the vicinity of the zygote cell (Figure (e)). Antipodal and synergid cells were degenerated at this stage. A proembryo is formed which is located on the suspensor, which is composed of a row of cells (Figure (f)).
4. Discussion
The generative meristem is formed as a result of differentiation of vegetative meristem. Since the inflorescence in basil is in the form of a verticillaster, a floret is observed in each shoot where each of the florets is developing into a fully grown flower. These results were in accordance with the findings reported in Ziziphus jujuba L. (Rhamnaceae)(Jafari Marandi and Niknam Citation2012).
O. basilicum has four stamens and each appears as a cellular mass on the receptacle. The anthers are tetrasporangiate and developed centrifugally according to the dicotyledonous-type (Davis Citation1962; Kai-vu et al. Citation1997). The tapetum layer is of secretory type which is in accordance with the findings of prior research surrounding the other members of Lamiaceae (Jiménez et al. Citation1995; Kai-vu et al. Citation1997; Maheswari Citation1950). A secretory type tapetum was also reported for Rosmarinus officinalis, which is one of Lamiaceae plants (Johri et al. Citation1992). Jiménez et al. (Citation1995) proposed to use “tapetum maturation” instead of the misunderstandable “tapetum degeneration”. The tapetum cells also start dividing at the earlier stages of the microspore mother cell meiosis divisions, and binucleated tapetum cells were observed which confirms the findings reported by Daskalova (Citation2004), Chehregani and Sedaghat (Citation2009), and Kai-vu et al. (Citation1997) on the Lamiaceae family. Meiosis results in dyads and tetrads in each microsporocyte. The tetrads in basil were tetrahedral, similar to the observations made by Kai-vu et al. (Citation1997) and Daskalova (Citation2004). Kartal (Citation2015) studied microsporogenesis and microgametogenesis in the endangered species Fritillaria stribrnyi (Liliaceae), and reported that meiotic division resulted in the formation of isobilateral types of tetrad (Kartal Citation2015).The mature pollen grains contain a vegetative and a generative cell at the earlier stage. The generative cell produces two sperms in the later stage. Tri-nucleate pollen grains in Lamiaceae were reported by Erdtman (Citation1945), Daskalova (Citation2004a), and (Wei et al. Citation2010). After the formation of a large central vacuole, the microspore’s nucleus undergoes the first mitosis, forming the generative cell and the vegetative cell (Jiménez et al. Citation1995). The elongated generative cell undergoes the second mitosis which results in two lenticular sperm cells. The pollen tube carries sperm cells into the ovule so pollen tube growth is one of the key steps in the fertilization process (Batygina Citation2002).
The findings of the present study showed that like the other members of the Lamiaceae family, the ovary is superior with axial placentation. The ovule is unitegumic, anatropous, and tenuinucellate. This confirms the findings of Daskalova (Citation2002), Kamellina and Dzevaltovsky (Citation1987), Kai-vu et al. (Citation1997), Wu and Cao (Citation2011), and Rad and Hajisadehian (Citation2014).
Daskalova (Citation2005) reported that the flowers in Nepeta (Lamiaceae) mostly have four normally developed locules of the pistil and a normally situated and developed style with bifid stigma. In this species, the stigma was also bifid (Daskalova Citation2005). The ovules in this species are anatropous, unitegumic, and tenuinucellate, which is in accordance with the results about other members of Lamiaceae (Daskalova Citation2005). Archeospore cells inside ovule primordia were quite distinguished from the other nucellus cells because of their large volume, dense cytoplasm, and voluminous nucleolus. Two archeospore cells formed in the plant under the epidermis layer of the ovule; this was also reported by Daskalova (Citation2002). In this primordium, an archeosporial cell produces a megaspore mother cell which undergoes meiosis, forming a linear or T-shaped tetrad. The micropylar cell is a functional megaspore that survives and will function in megagametophyte development. Analysis of the megasporogenesis of the plant showed that the tetrad is linear; this was also reported by Daskalova (Citation2002), Chehregani and Sedaghat (Citation2009) in Brassicaceae, and Kai-vu et al. (Citation1997) in the Lamiaceae family, observing that three cells in the tetrad degenerated and only the cell at the micropyllar pole remained, which is in fact the initial cell of the embryo sac (Chehregani and Sedaghat Citation2009).
The degeneration process is time consuming, as already reported by Daskalova (Citation2002) and also Matthews et al. (Citation1984). The embryo sac mother cell eventually generates the embryo sac with eight nuclei. The presence of three antipode cells is a particular feature of the Polygonum type that was observed in the studied plant species. The antipodal cells degenerated immediately in prefertilization stages. This was also reported for Arabidopsis thaliana (Jürgens et al. Citation1991). The Polygonum type development of embryo sac is the most common type and was observed in about 70% of flowering plants(Tanaomi et al. Citation2016). The antipodal cells were arranged alongside while their bottoms stretched considerably, touching the chalazal pole of the embryo sac and confirming the findings of Kamellina and Dzevaltovsky(Citation1987) and Daskalova (Citation2002). A distinguishing feature of the species is the presence of pollen-directing tissue. The peripheral tissue, which is developed from the primary archeosporal cells, differentiates at the micropyle region of the nucellus. The layers of this tissue vary in numbers (between 1 and 20). There is a strand in the sample composed of long cells stretching between micropyle and ovary. The pollen-directing tissue transfers the pollen tube to the oosphere. Another feature of the sample was the presence of hypostasis which is an organized tissue at the base of the nucellus and integument. In tenuinucelate ovules, like those of the Lamiaceae family, hypostasis is located immediately below the embryo sac. It is believed that there is a relationship between the length of hypostasis and the pattern of nucellus, teguments, and the embryo sac. In the ovule, usually after the 2-, 4-nucleate embryo sac stage, a hypostasis forms in its chalaza region, as in most Brassicaceae taxa (Prasad Citation1977; Shamrov Citation2002; Vijayabaghavan and Prabhakar Citation1981). Figueiredo and Köhler (Citation2016) reported that during female gametogenesis the maternal tissues tightly regulate megagametophyte formation and the interplay between the sporophyte and the fertilization products, embryo and endosperm, has major implications in the formation of a viable seed (Figueiredo and Köhler Citation2016).
Disclosure statement
No potential conflict of interest was reported by the authors.
Acknowledgements
The authors acknowledge the insight from their teacher, Professor Majd, and comments from the reviewer, Dr Chehregani, who meticulously read the research report and provided invaluable advice to improve the quality of the report. They also acknowledge Islamic Azad Islamic University laboratory for use of equipment.
References
- Batygina, T. 2002. Embryology of flowering plants Terminology and concepts Vol 1: generative organs of flower. Enfield, USA.: Science Publishers Inc.
- Bentham G. 1848. Labiatae in prodomus systematic naturalis regni vegetabilis pars XII. Paris: Treuttel and wurtz.
- Cao L, Xia N, Deng Y. 2008. Embryology of Handeliodendron bodinieri (Sapindaceae) and its systematic value: development of male and female gametophytes. Plant Syst Evol. 274(1):17–23. 10.1007/s00606-008-0024-0
- Chehregani A, Sedaghat M. 2009. Pollen grain and ovule development in Lepidium vesicarium (Brassicaceae). Int J Agriculture & Biology. 11(5):1560–8530.
- Daskalova T. 2002. Macrosporogenesis and development of the female gametophyte in Salvia pratensis L. Phytol Balanica. 8(2):205–209.
- Daskalova T. 2004a. On some specificities of seed formation in Salvia nemorosa (Lamiaceae). Phytologia Balcanica. 10(1):79–84.
- Daskalova T. 2004. Histological structure of the microsporangia, microsporogenesis and development of the male gametophyte in Nepeta cataria (Lamiaceae). Phytologia Balcanica. 10(2–3):241–246.
- Daskalova T. 2005. Polygamy in some species of Nepeta (Lamiaceae). Phytolog Balc. 11(1):79–98.
- Daskalova T, Genova E. 1996. Histological structure of the anthers and microsporogenesis in Hyssopus officinalis L. ssp. aristatus (Godr.) Briq. (Lamiaceae). Bot Jahrb Syst. 118(3):297–302.
- Davis GL. 1962. Embryological studies in the compositae, I. Sporogenesis, Gametogenesis, and Embryogeny in Cotula australis (Less.) Hook. F. Aust J Bot. 10(1):1–12. 10.1071/BT9620001
- Erdtman G. 1945. Pollen morphology and plant taxonomy. IV. Labiatae. Verbenaceae and Avicenniaceae. Svensk Botanisk Tidskrift. 39:279–285.
- Figueiredo DD, Köhler C. 2016. Bridging the generation gap: communication between maternal sporophyte, female gametophyte and fertilization products. Curr Opin Plant Biol. 29:16–20. 10.1016/j.pbi.2015.10.008
- Jafari Marandi, S, Niknam, F. 2012. Pollen and Anther Development in Ziziphus jujuba L.(Rhamnaceae). Adv Environ Biol, 6(8), 2339–2343.
- Javanmardi J, Stushnoff C, Locke E, Vivanco J. 2003. Antioxidant activity and total phenolic content of Iranian Ocimum accessions. Food Chem. 83(4):547–550. 10.1016/S0308-8146(03)00151-1
- Jiménez JLU, Fernández PH, Schlag MG, Hesse M. 1995. Pollen and tapetum development in male fertile Rosmarinus officinalis L. (Lamiaceae). Grana. 34(5):305–316. 10.1080/00173139509429064
- Johri, BM, Ambegaokar, KB, Srivastava, PS. 1992. The background comparative embryology of angiosperms. Berlin Heidelberg: Springer; p. 1–2.
- Joshi, RK. 2014. Chemical composition and antimicrobial activity of the essential oil of Ocimum basilicum L.(sweet basil) from Western Ghats of North West Karnataka, India. Anc Sci Life, 33(3):151–156.
- Juliani, H, Simon, J. 2002. Antioxidant activity of basil. Trends in New Crops and New Uses. 575–579.
- Jürgens G, Mayer U, Berleth T, Miséra S. 1991. Genetic analysis of pattern formation in the Arabidopsis embryo. Development. 113(Supplement 1):27–38.
- Kai-vu P, Wen Jie W, Shi-liang Z. 1997. Embryological study on Mosla chinensis (Lamiaceae). Acta Bot Sinica. 39(2):111–116.
- Kamellina O, Dzevaltovsky A. 1987. Lamiaceae. In: Batygina M, Yakovlev TB, editors. Comparative embryology of flowering plants. Leningrad (In Russian): Nauka; p. 225–236.
- Kartal C. 2015. Microsporogenesis, microgametogenesis and in vitro pollen germination in the endangered species Fritillaria stribrnyi (Liliaceae). Caryologia. 68(1):36–43. 10.1080/00087114.2014.998947
- Krüger H, Wetzel SB, Zeiger B. 2002. The chemical variability of Ocimum species. J Herbs Spices Med Plants. 9(4):335–344. 10.1300/J044v09n04_11
- Mačukanović-Jocić M, Rančić D, Dajić Stevanović ZD. 2007. Floral nectaries of basil (Ocimum basilicum): morphology, anatomy and possible mode of secretion. South African J Bot. 73(4):636–641. 10.1016/j.sajb.2007.06.008
- Maheswari JK. 1950. An intoduction to the embryology of angiosperms. New York, NY: McGraw-Hill.
- Majd A, Mehrafarin A, Jonoubi P. 2014. Qualitative and quantitative assessment of the essential oils of Origanum vulgare and Origanum majorana in Iran. J Med Plants. 2(50):163–171.
- Matthews MA, Volkenburgh EV, Boyer, JS 1984. Acclimation of leaf growth to low water potentials in sunflower. Plant, Cell Environ. 7(3):199–206.
- Mohammed S, Tesfaye K. 2015. Morphological and molecular characterization of Lepidium sativum population collected from Ethiopia. Afr J Plant Sci. 9(4):215–222.
- Moon, H-K, Hong, S-P. 2003. Pollen morphology of the genus Lycopus (Lamiaceae). Ann Bot Fenn. 40:191–198.
- Oram, R, Kirk, J. 1992. Breeding Indian mustard for Australian conditions. In: Hutchinson KJ, Vickery PJ, editors. Proceedings of the Australian Agronomy Conference. New South Wales: Australian Society of Agronomy. p. 467–470.
- Paton A. 1992. A synopsis of Ocimum L. (Labiatae) in Africa. Kew Bull. 47(3):403–435. 10.2307/4110571
- Prasad K. 1977. The development and structure of basal body in the ovule and seed of certain species of Cruciferae. Bot Jahrb. 98(2):266–272.
- Rad AC, Hajisadehian S. 2014. Microsporogenesis, megasporogenesis and gametophyte development in Senecio glaucus L. Thaiszia J Bot. 24(2):89–100.
- Raina AP, Kumar A, Dutta M. 2013. Chemical characterization of aroma compounds in essential oil isolated from “Holy Basil”(Ocimum tenuiflorum L.) grown in India. Genet Resour Crop Ev. 60(5):1727–1735. 10.1007/s10722-013-9981-4
- Raj B. 1983. A contribution to the pollen morphology of Verbenaceae. Rev. Palaeobot. Palynol. 39(3–4):343–422.
- Ramegowda V, Senthil-Kumar M. 2015. The interactive effects of simultaneous biotic and abiotic stresses on plants: Mechanistic understanding from drought and pathogen combination. J Plant Physiol. 176:47–54. 10.1016/j.jplph.2014.11.008
- Shamrov I. 2002. Ovule and seed morphogenesis in Capsella bursa-pastoris (Brassicaceae) in connection with peculiar mode of endothelium formation. Bot Zh. 87(2):1–18.
- Sirousmehr A, Arbabi J, Asgharipour MR. 2014. Effect of drought stress levels and organic manures on yield, essential oil content and some morphological characteristics of sweet basil (Ocimum basilicum). Adv Environ Biol. 8(4):880–886.
- Svecova E, Neugebauerova J. 2010. A study of 34 cultivars of basil (Ocimum L.) and their morphological, economic and biochemical characteristics, using standardized descriptors. Acta Univ Sapientiae, Alimentaria. 3:118–135.
- Tanaomi N, Jonoubi P, Chehregani Rad A, Majd A, Ranjbar M. 2016. Embryology of Onobrychis persica Sirj. and Rech. f. (Fabaceae) and its systematic implications. Caryologia. 69(3):1–11.
- Vijayabaghavan M, Prabhakar K. 1981. Ontogenetical and histochemical studies on chalazal proliferating tissue in Iberis amara and Alyssum maritimum. Beitrage zur Biologie der Pflanzen. 56(1):7–17.
- Waterman AH. 1960. Pollen grain studies of the Labiatae of Michigan. Webbia. 15(2):399–415. 10.1080/00837792.1960.10669704
- Wei Q, Li F, Liu R, Hu L, Dong X, Gao Y, Hu B. 2010. Microsporogenesis and male gametophyte development in Coleus blumei Benth. J Northeast Agric Univ. 2010(12):48–52.
- Wu Y, Cao Y. 2011. Megasporogenesis and female gametophyte development in Salvia miltiorrhiza Bge. J Qingdao Agric Univ (Nat Sci). 2011(2):133–137.
- Yeung, E. 1984. Histological and histochemical staining procedures. In: Vasil, IK, editor. Culture and Somatic Cell Genetics of Plants, Vol. 1. Orlando, Florida: Academics Press; p. 689–697.