Abstract
Meiotic chromosomal behavior of five recommended and released Philippine varieties of sugarcane and their parents, namely VMC 95-09 (Q 90 × Unidentified), PSR 97-45 (VMC 74-292 × CP 72-1312), PSR 00-343 (VMC 84-947 × Unidentified), PSR 00-161 (VMC 86-550 × VMC 87-599), and PSR 00-34 (VMC 84-194 × PHIL 89-43) were characterized and analyzed. Samples were collected from the tassel source of Philippine Sugar Research Institute Foundation Inc. (PHILSURIN) located in Negros Occidental, Philippines. Gametic number for each variety was determined at diakinesis and at least 50 cells per meiotic stage was analyzed. All varieties exhibited chromosome mosaicism at diakinesis with n = 40–60. Chromosome clumping was observed at diakinesis of Q 90 (68%), CP 72-1312 (35%), and PSR 97-45 (22%) pollen mother cells. High frequency of bivalent formation (68–100%) was observed, both in parents and hybrids, indicating that chromosome pairing is autosyndetic. Irregularities at meiosis were also observed at high frequencies both in parents and hybrids. Multiple metaphase I plate, non-congression, and laggards were observed at meiosis I and meiosis II. Chromosome contribution of parents to each hybrid was determined based on gametic chromosome number of the parents and their meiotic behavior. Results of this study can be used in identifying best hybrids that can be utilized in an inter or intra-species hybridization to produce better performing sugarcane varieties.
Introduction
The sugarcane industry started in the Philippines long before Spanish colonization and continued to prosper especially during American occupation. In 1902–1913 trade agreements were established between the USA and the Philippines which allowed export of sugar products to the USA. This led to the construction of over 47 mills and an increase of 502% in sugar production from 206,000 tons in 1912–1913 to 1,565,405 tons in 1933–1934 (SRA Citation2012).
As of 2006, it is estimated that ₱24.91 billion of Philippine national agricultural production was contributed by the sugarcane industry. The industry generates around 600,000 jobs directly in sugarcane farming and an additional five million workers are indirectly dependent on sugar production for their employment (Investment Opportunity Sugarcane Plantation Citation2011). The Philippine government is planning to increase sugarcane area, farm productivity, and sugar yield as response to the ASEAN (Association of South East Asian Nations) Free Trade Area (AFTA) agreement. In this program, the tariff on imported sugar from competing ASEAN countries was reduced from 38% in 2011 to 5% in 2015 (Investment Opportunity Sugarcane Plantation Citation2011; Sugarcane Industry Road Map (2011–2016)Citation 2012). With implementation of AFTA, there is a need for research institutions to improve current Philippine sugarcane varieties in terms of yield, resistance, stress tolerance, and sugar content. These measures will ensure the good performance of Philippine sugar in the highly competitive market of the ASEAN countries.
Sugarcanes are highly polyploid plants as a result of hybridization between at least three or four Saccharum species. Because of this, sugarcane cultivars are found to be genetically complex and cannot be identified by single gene traits (Heinz Citation1991).
In Citation1924, Bremer first identified the chromosome number of S. officinarum, noble canes, to be 2n = 80, x = 10. This was further supported by studies by Dutt and Rao (Citation1933), Price (Citation1960), and Jagathesan et al. (Citation1970). Results of their studies showed that S. officinarum clones in global collections have the same chromosome number of 2n = 80. Thus, only sugarcane clones with high sucrose content and a chromosome number of 2n = 80 are considered to be typical S. officinarum species. Other clones with high sucrose content but with aneuploid chromosome numbers are considered as hybrids or sometimes referred to as atypical S. officinarum clones.
Modern sugarcane cultivars that are commercially used have a chromosome number of 2n = 100–130. These hybrids are extremely polyploid and are products of repetitive hybridization between domesticated S. officinarum and S. spontaneum species. Studies by D’Hont et al. (1996) and Piperidis and D’Hont (Citation2001) revealed the genome composition of these hybrids. It is made up of 70–80% S. officinarum, 10–20% S. spontaneum, and 5–17% recombinant chromosomes (Alwala and Kimbeng Citation2010).
In the Philippines, several studies have been conducted to understand chromosomal behavior of sugarcane cultivars that exist in the country. Divinagracia and Ramirez (Citation1976) studied the cytology of indigenous S. officinarum cultivars in the Philippines. They established four groups based on the chromosome number of the species surveyed. Group I has a chromosome number of 2n = 80 and is commonly known as Negros Purple and Luzon White. Group II sugarcanes are Cebu Purple and Zamboanga White. This group has a chromosome number of 2n = 120. Palawan White comprises Group III with a chromosome number of 2n = 128 while Pampanga Red and Hilongos are classified as Group IV, with 2n = 136. From these data, Negros Purple and Luzon White are the Philippine cultivars considered to belong to the noble canes, or typical S. officinarum.
A cytogenetic research on the hybrids of cultivated sugarcane (S. officinarum Caña Azul; 2n = 102 to 106) and talahib (S. spontaneum SES 84A; 2n = 96) was done by Engle et al. (Citation1979). They were able to identify three hybrid groups with the following chromosome numbers: 2n = 63, 2n = 88, and 2n = 100–108. Hybrids that have low chromosome number have morphological characteristics more similar to the S. spontaneum parent, while hybrids with high chromosome number are morphologically similar to the S. officinarum parent.
Engle, Escote, et al. (Citation1980) did another cytogenetic study of the hybrid derived from the cross between Hawaiian commercial sugarcane variety (2n = 112) and S. spontaneum (2n = 80) from Iloilo, Philippines. Hybrids were found to have the expected chromosome number of 2n = 96 and morphological characteristics were intermediate between the parents. In the same year, Engle, Sedano, et al. (Citation1980) did a cytogenetic study on the hybrids derived from open-pollinated S. officinarum and S. spontaneum F1 hybrids. Results showed that parents with high chromosome number produced offspring that have higher chromosome number than offspring that came from parents with low chromosome number. Morphological analysis revealed the same results as in Engle (Citation1979) where hybrids with high chromosome number have characteristics similar to S. officinarum, while hybrids with low chromosome number are more similar to S. spontaneum.
As discussed in previous paragraphs, most of the cytogenetic studies in Philippine sugarcane varieties were conducted in late 1970s to early 1980s. Thus, there are no cytogenetic data for the important sugarcane varieties that were released after these years. Knowledge on cytogenetic behavior of new recommended sugarcane varieties is important for more effective breeding programs. Detailed information on the meiotic behavior of plant species is important as this process is involved in producing fertile gametes, which is an important consideration in intra and inter-species hybridization programs. Through this, the chromosome number, chromosome behavior, as well as cytogenetic relationships between closely related varieties or cultivars can be determined. These can then be further used to identify pathways that lead to the transfer of desirable characteristics from a closely related species to an improved sugarcane cultivar. The present study is very timely as the sugar industry in the Philippines is being revived for food, both locally and internationally, and for other product opportunities like biofuel production.
This study aimed to determine the meiotic behavior of sugarcane hybrids and their respective parents; and to analyze the chromosomal contribution of the respective parents to each of the five recommended and released Philippine sugarcane hybrids.
Materials and methods
Sample collection
Microsporocytes of the five recommended and released Philippine sugarcane hybrids and their parents were collected from PHILSURIN tassel source located in Negros Occidental, Philippines. These varieties were maintained and asexually propagated by PHILSURIN. Time of microsporocyte collection was from 9:00 am to 12:00 pm. Samples were immediately preserved in freshly prepared Farmer’s solution (3:1 95% ethanol:glacial acetic acid) for cell fixation. All sugarcane varieties used in this study are presented in Table .
Table 1. Parents of five PHILSURIN recommended and released varieties namely: VMC 95-09, PSR 97-45, PSR 00-343, PSR 00-161, and PSR 00-34.
Slide preparation and documentation
After 48 h in fixative, samples were transferred in 70% ethanol and were stored at 4°C. Slides were prepared using the acetocarmine squash technique. At least five florets per variety were analyzed. These florets were then obtained from one to two flowers in each sugarcane variety. Cover slips were pressed by the thumb to flatten cells and spread the chromosomes. Cells were destained using 45% acetic acid and staining was enhanced by allowing slides to be gently heated over an alcohol lamp. The chromosome/cytoplasm contrast was monitored until a good distinction of chromosomes was attained. Slides were then sealed using the paraffin-balsam mixture. At least 50 meiotic cells were observed for each meiosis I and meiosis II stage. Counting of bivalents and gametic chromosome number determination was done at diakinesis using the oil immersion objective (OIO, 1000×). Photomicrographs were taken using Zeiss Axioskop Microscope (Carl Zeiss Jena GmbH, Jena, Germany) and Canon EOS 550D (Canon Inc., Tokyo, Japan) Camera.
Results
VMC 95-09 (Q90 × Unidentified)
Meiosis I
Q90 (female parent). Cytogenetic analysis showed that this S. officinarum variety has cells with gametic chromosome number of n = 40–53, and 78% of cells have n = 43–49. The gamete chromosome number with the highest frequency was n = 44 (14%). Chromosome clumping was observed in this sugarcane variety and can be associated with multivalent formation. Normal bivalent formation was also observed but in relatively low frequencies. A total of 25 chromosomal configurations were recorded and formation of one to two quadrivalents, one trivalent, and one to six univalents were seen. All chromosomal configurations, the equivalent gametic chromosome number, and frequencies observed in Q 90 are presented in Table .
Table 2. Chromosome configurations at diakinesis observed in Saccharum officinarum L. var. Q90 and VMC 95-09.
Q90 was observed to have a high frequency of irregular metaphase I behavior, with 86.49%. These irregularities were observed as some chromosomes lag behind in aligning at the equatorial plate. Non-congression of chromosomes and the presence of multiple metaphase plates were also observed in this sugarcane variety. Laggards were also recorded in anaphase and telophase I. There were three to nine laggards observed in anaphase I, but only one to six lagging chromosomes in telophase I (Table and Figure ).
Table 3. Chromosome behavior at metaphase I, anaphase I, and telophase I of five recommended and released Philippine varieties of Saccharum officinarum L. and their parentals.
Figure 1. Meiosis I cells of Saccharum officinarum L. (OIO, 1000×). (a–d) var. Q90: (a) diakinesis with 49II; (b) non-congression at metaphase I; (c) anaphase I with laggards; (d) telophase I with laggards. (e–h) var. VMC 95-09: (e) diakinesis with 46II; (f) metaphase I; (g) anaphase I with laggards; (h) telophase I with laggards.
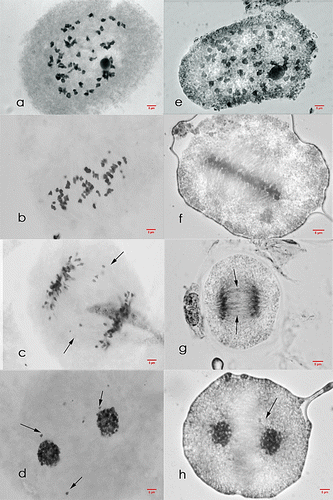
VMC 95-09 (hybrid). The hybrid showed diakinesis cells with gametic chromosomal range of n = 40–52 with n = 46 as the chromosome number with the highest frequency of 37.5%. Nine chromosomal configurations were observed in diakinesis and all configurations were showing normal bivalent formation (Table ).
Unlike its female parent, VMC 95-09 has a high frequency of normal metaphase I, but laggards and non-congressional alignment of chromosomes at metaphase plate were still observed. Though metaphase I is mostly normal, all anaphase I cells observed have three to five laggards while telophase I has one to four laggards (Table and Figure ).
Meiosis II
Q90 (female parent). At prophase II, 54.29% of cells have one to three laggards. Since the number of laggards in prophase II was observed to be less than the number of laggards present in telophase I, then most laggards in the previous stage were able to catch up with other chromosomes and were included in the nucleus of the two daughter cells produced after meiosis I.
Observations at metaphase II revealed that 49.10% of cells have laggards and non-congression of chromosomes. Anaphase II cells were observed to have two to seven laggards while 52% of telophase II cells were found to have two laggards. However, when tetrads were examined, normal tetrad cells occurred at high frequency (94.23%). Meiosis II chromosome behavior is presented in Table and Figure .
Table 4. Chromosome behavior meiosis II of five recommended and released Philippine varieties of Saccharum officinarum L. and their parentals.
Figure 2. Meiosis II cells of Saccharum officinarum L. (OIO, 1000×). (a–e) var. Q90: (a) prophase II with laggards; (b) metaphase II with laggards; (c) anaphase II with laggards; (d) telophase II with laggards; (e) tetrads with laggards. (f–j) var. VMC 95-09: (f) prophase II with laggards; (g) normal metaphase II; (h) normal anaphase II; (i) telophase II with laggards; (j) normal tetrad cells.
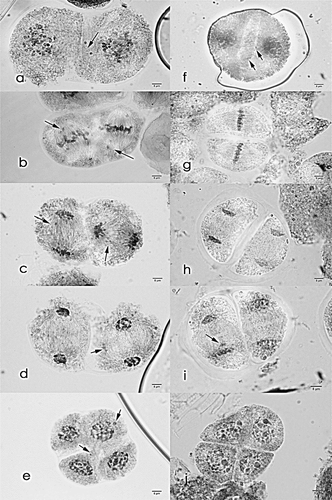
VMC 95-09 (hybrid). Most of the laggards observed in anaphase I and telophase I were included in the nuclei of the dyads produced after meiosis I. However, prophase II cells with one to two laggards have higher frequency than normal prophase II cells (Table ). Like metaphase I, metaphase II cells have high frequency of normal cells despite high occurrence of laggards in prophase II. This is possible as laggards observed in prophase II can still be included in the aligning chromosomes at metaphase II as they fall near or within the range of the equatorial plate (Escote Citation1978). A high frequency of laggards was observed in anaphase II but most of the laggards managed to catch up with other chromosomes as laggard frequency decreased in telophase II. Further examination in the final meiotic II stage showed that all lagging chromosomes might be included in the nucleus of the tetrad formed, as all cells observed are 100% normal (Table ). Photomicrographs of the different meiosis II stages are presented in Figure .
PSR 97-45 (VMC 74-292 × CP 72-1312)
Meiosis I
VMC 74-292 (female parent). This variety showed 11 chromosome configurations at diakinesis with n = 53 as the highest gametic chromosome number and n = 45 as the lowest gametic chromosome number (Table ). The gametic chromosome number of n = 47 was observed to be the most prevalent (24.14%) while 93.11% of cells have n = 47–53. Eight out of 11 chromosomal configurations showed normal bivalent formation while the remaining three configurations showed univalent formation.
Table 5. Chromosome configurations at diakinesis observed in Saccharum officinarum L. var. VMC 74-292, CP 72-1312, and PSR 97-45.
Table and Figure present the meiosis I chromosome behavior of VMC 74-292. It can be seen that this variety has a high frequency of abnormal metaphase I cells, with 86.72%. Lagging chromosomes, non-congression, and multiple metaphase plate are the abnormalities observed in metaphase I. All anaphase I cells were observed to have six to 15 laggards. Frequency of laggards as well the number of chromosomes lagging behind was found to decrease in telophase I.
Figure 3. Meiosis I cells of Saccharum officinarum L. (OIO, 1000×). (a–d) var. VMC 74-292: (a) chromosome stickiness at diakinesis; (b) multipolar metaphase I plate; (c) anaphase I with laggards; (d) telophase I with laggards. (e–h) var. CP 72-1312: (e) diakinesis with 47II + 2I; (f) metaphase I; (g) anaphase I with laggards; (h) telophase I with laggards. (i–l) var. PSR 97-45: (i) diakinesis with 41II + 4I; (j) non-congression at metaphase I; (k) anaphase I with laggards; (l) telophase I with laggards.
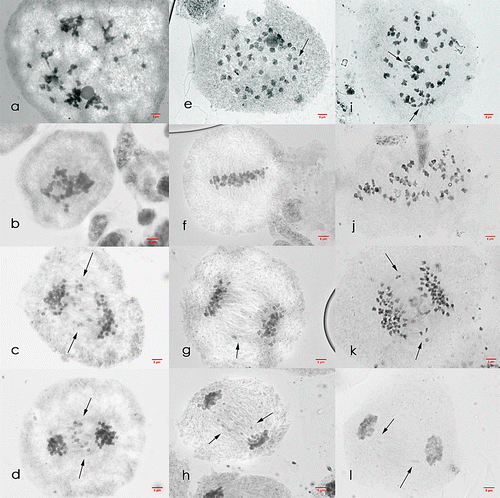
CP 72-1312 (male parent). CP 72-1312 is a hybrid produced from the cross between CP 65-357 and CP 56-63. Its ancestry can be traced back from CP 29-320, CP 38-34, S. barberi, and Ashy Mauritius (Engle et al. Citation2012). A total of 19.24% of cells have a gametic chromosome number of n = 48, the highest frequency observed among chromosome mosaics in this variety, and 84.62% have n = 41–48. A total of 24 chromosomal configurations were observed in diakinesis and chromosomal clumping was seen. Clumping of chromosomes can be associated to multivalent formations and persistent chromatin threads that connect two or more distant bivalents in the cytoplasm (Table ).
There were equal numbers of metaphase I cells with normal and abnormal chromosome behavior (Table ). Half (50%) of metaphase I cells were seen to have laggards, non-congressional alignment of chromosomes at the equatorial plate, and presence of multiple metaphase plate. Cells at anaphase I with three to eight laggards were observed to occur at 88.24% while 57.63% of telophase I were seen to have one to nine lagging chromosomes during chromosomal migration towards the opposite poles of the cell (Table ). Figure presents the photomicrographs of CP 72-1312 cells at diakinesis, metaphase I, anaphase I, and telophase I.
PSR 97-45 (hybrid). The hybrid has a majority of cells with gametic chromosome number of n = 49 and n = 51 with frequencies both at 14% (Table ). Cells with n = 51 have three configurations: 51II, 49II + 4I, and 2III + 47II + 2I. On the other hand, there were also three configurations that led to n = 49 and these were 49II, 48II + 2I, and 47II + 4I with 49II as the configuration with the highest frequency among the three, 6%. The gametic chromosomal range of n = 43–51 was observed at 78% of diakinesis cells.
Metaphase I cells of PSR 97-45 have high irregular behavior with 75.90% of cells having laggards, non-congressional alignment of chromosomes, and multiple metaphase plate formation (Table ). Cells with laggards at anaphase I have equal frequency with normal anaphase I cells. The number of laggards ranges from one to 12 and these were seen to be late disjoining bivalents. Telophase I cells with laggards exist in relatively low frequency (31.71%) with only one to three lagging chromosome observed (Table and Figure ).
Meiosis II
VMC 74-292 (female parent). A total of 48.72% of VMC 74-292 prophase II cells contain laggards but much fewer than in telophase I. This is an indication that some of the lagging chromosomes were able to be included in the nuclei of the two daughter cells.
Abnormal behavior of chromosomes at metaphase II, anaphase II, and telophase II were observed to occur at very high frequencies (Table ). However, when tetrad cells were observed, normal cells are greater in frequency than cells with laggards. This shows that even if most cells in telophase II had laggards, these chromosomes were still able to catch up with other migrating chromosomes, thus forming normal tetrad cells. Cells of VMC 74-292 undergoing meiosis II are presented in Figure .
Figure 4. Meiosis II cells of Saccharum officinarum L. (OIO, 1000×). (a–e) var. VMC 74-292: (a) prophase II with laggards; (b) metaphase II with laggards; (c) anaphase II with laggards; (d) telophase II with laggards; (e) normal tetrad cells. (f–j) var. CP 72-1312: (f) prophase II with laggards and chromosomes caught at the cell; (g) metaphase II with laggards; (h) anaphase II with laggards; (i) telophase II with laggards; (j) tetrads with laggards. (k–o) var. PSR 97-45: (k) normal prophase II; (l) normal metaphase II; (m) anaphase II with laggards; (n) telophase II with laggards; (o) normal tetrad cells.
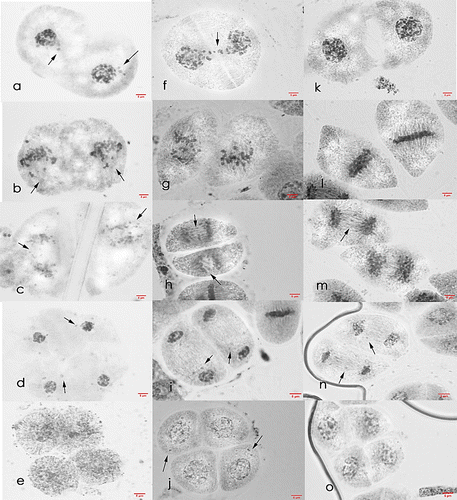
CP 72-1312 (male parent). One to three laggards were observed at prophase II with a frequency of 45.95% (Table ). Metaphase II had cells that are mostly normal (61.76%), but most cells at anaphase II have two to five laggards. The frequency of cells with laggards at telophase II is almost equal to the frequency of anaphase II cells with laggards: 75% and 79.17%, respectively. With this high frequency of laggards at previous stages, almost half (47.06%) of tetrads formed have one to three laggards (Figure ).
PSR 97-45 (hybrid). Prophase II cells were observed to be mostly normal with a frequency of 61.22%. Cells with laggards were observed to have one to two lagging chromosomes. Though most prophase II cells are normal, metaphase II cells have a high frequency of irregular behavior. Almost 61% of cells are found to exhibit laggards, double plate formation, and non-congressional alignment of chromosomes. Anaphase II cells mostly have three to five laggards while almost half, 47.27%, of telophase II cells have one to four laggards. When tetrads were viewed under the microscope, 92.98% of cells have normal tetrad formation (Table and Figure ).
PSR 00-343 (VMC 84-947 × Unidentified)
Meiosis I
VMC 84-947 (female parent). This variety is produced from a cross between POJ 2725, a sugarcane variety released in 1925, with an unidentified male parent (Engle et al. Citation2012). Cytogenetic analysis at diakinesis showed gametic chromosomal range of n = 40–53. Cells with n = 49 and n = 43 exist in equal frequencies (12.73%) and have the highest frequency among the varying chromosome numbers observed (Table ). Most cells exhibited normal bivalent formation with a total of 21 chromosomal configurations observed.
Table 6. Chromosome configurations at diakinesis observed in Saccharum officinarum L. var. VMC 84-947 and PSR 00-343.
Most metaphase I cells of VMC 84-947 exhibited irregular meiotic behavior like multiple metaphase plate formation, presence of lagging chromosomes, and non-congressional alignment of chromosomes at the equatorial plate. The majority of cells at anaphase I (78.95%) were observed to have one to eight laggards and this high frequency of laggard occurrence was also found in telophase I. At telophase I, 70.69% of cells were found to have one to five lagging chromosomes (Table and Figure ).
Figure 5. Meiosis I cells of Saccharum officinarum L. (OIO, 1000×). (a–d) var. VMC 84-947: (a) diakinesis with 49II; (b) metaphase I; (c) anaphase I with laggards; (d) telophase I with laggards. (e–h) var. PSR 00-343: (e) diakinesis with 45II; (f) non-congressional alignment and metaphase I with tripolar spindle; (g) anaphase I with laggards; (h) telophase I with laggards.
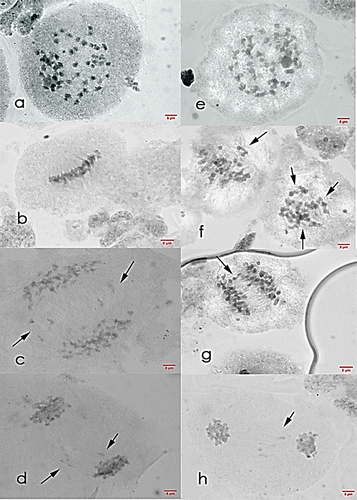
PSR 00-343 (hybrid). The hybrid showed cells with gametic chromosomal range of n = 40–53, with n = 40 as the gametic chromosome number with highest frequency of 16.00% (Table ). Cells with n = 40 exhibited only one chromosomal configuration of 40II. Normal bivalent formation was prevalent in PSR 00-343 with only 8.00% of cells were observed to have univalents. Since all univalents seen were found to be parallel or close to each other, it is possible that they were formed due to early disjunction of the chromosome pairs.
In Table , it can be seen that the hybrid had high frequency of irregular metaphase I behavior (73.86%) and abnormalities such as laggard formation, non-congressional alignment of chromosomes and multiple metaphase plate were observed in PSR 00-343 meiotic cells. Segregation of chromosomes was also observed to have a high frequency of abnormal behavior. More than half (58.33%) of anaphase I cells were observed to have two to five laggards while 59.46% of telophase I cells have one to four laggards (Figure ).
Meiosis II
VMC 84-947 (female parent). Though high laggard frequency was observed at ana-telophase I stage, 60.71% of cells at prophase II exhibited normal meiotic behavior. However, irregular chromosome behavior was found to exist in high frequencies at metaphase II (60.25%), anaphase II (68.18%), and telophase II (66.67%). This shows that abnormal meiotic behavior observed in the preceding meiosis II stages are not due to the existence of non-aligned chromosomes observed in prophase II alone. Tetrads formed were also examined and in spite of high abnormal meiotic behavior frequency in metaphase II, anaphase II, and telophase II, most tetrads that were produced by VMC 84-947 do not have laggards (Table and Figure ).
Figure 6. Meiosis II cells of Saccharum officinarum L. (OIO, 1000×): (a–e) var. VMC 84-947: (a) prophase II; (b) non-congressional alignment at metaphase II; (c) anaphase II with laggards; (d) telophase II with laggards; (e) normal tetrad cells. (f–j) var. PSR 00-343: (f) prophase II with laggards and chromosomes caught at the cell plate; (g) metaphase II with laggards; (h) anaphase II with laggards; (i) telophase II with laggards; (j) normal tetrad cells.
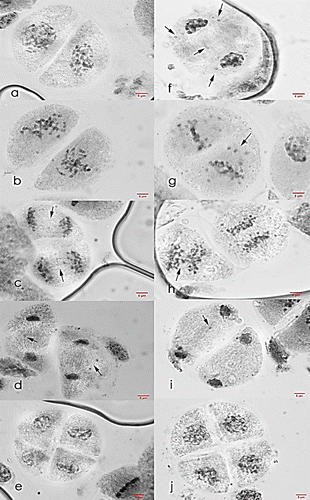
PSR 00-343 (hybrid). A total of 44% of cells at prophase II were seen to have laggards but in lower numbers than those observed in telophase I. Metaphase II had high irregular meiotic frequency of 89.28%. All anaphase II cells were observed to have four to six laggards while 58.70% of telophase II cells have one to four laggards. Tetrad analysis showed that lagging chromosomes at anaphase and telophase II were able to catch up with other segregating chromosomes as 90% of cells were observed to be normal and only 10% have at most one lagging chromosome (Table ). Representative meiosis II photomicrographs are presented in Figure .
PSR 00-161 (VMC 86-550 × VMC 87-599)
Meiosis I
VMC 86-550 (female parent). This is a sugarcane variety that was released in 1995 and was produced through a cross between PHIL 56-226 with an unidentified male parent (Engle et al. Citation2012). Its diakinesis cells were observed to have 14 chromosomal configurations, with n = 60 as the highest gametic chromosome number and n = 47 the lowest. The majority of cells (88.21%) were observed to have n = 50–56 and n = 51 as the gametic chromosome number with highest frequency of 20.59% (Table ).
Table 7. Chromosome configurations at diakinesis observed in Saccharum officinarum L. var. VMC 86-550, VMC 87-599, and PSR 00-161.
A high frequency of irregular meiotic behavior was observed in metaphase I with 40.94% of cells showed lagging chromosomes, 18.79% had non-congressional alignment, and 23.49% exhibited multiple metaphase plate. Like metaphase I, most cells in anaphase I were observed to have lagging chromosomes while almost half, 48% of cells in telophase I was observed to have one to 10 laggards (Table and Figure ).
Figure 7. Meiosis I cells of Saccharum officinarum L. (OIO, 1000×): (a–d) var. VMC 86-550: (a) diakinesis with 51II; (b) non-congression at metaphase I; (c) anaphase I with laggards; (d) telophase I with laggards. (e–h) var. VMC 87-599: (e) diakinesis with 45II; (f) non-congression at metaphase I; (g) anaphase I with laggards; (h) telophase I with laggards. (i–l) var. PSR 00-161: (i) diakinesis with 45II; (j) non-congression at metaphase I; (k) anaphase I with laggards; (l) telophase I with laggards.
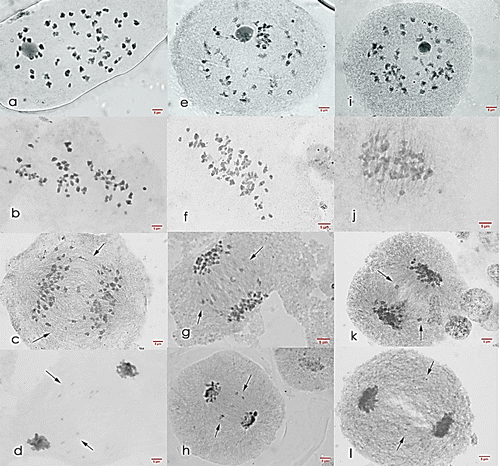
VMC 87-599 (male parent). Cells at diakinesis stage showed a gametic chromosome number of n = 40–57, with 19.60% of cells having n = 45, the highest frequency observed. Three configurations were observed that led to n = 45 and these are: 45II (15.68%), 44II + 2I (1.96%), and 43II + 4I (1.96%). VMC 87-599 is a hybrid between VMC 76-505 and PHIL 55-220 (Engle et al. Citation2012). Table shows the chromosome behavior of VMC 87-599 at diakinesis.
Like VMC 86-550, VMC 87-599 have high abnormal metaphase I frequency. Cells with laggards, non-congressional alignment of chromosomes, and formation of multiple metaphase plate was found to be evident in this stage of this sugarcane variety. All anaphase I cells were observed to have one to nine laggards while 76.27% of telophase I cells have one to six lagging chromosomes (Table and Figure ).
PSR 00-161 (hybrid). The hybrid was observed to have cells with gametic range of n = 40–58, with 68% of cells have n = 44–50. The chromosome number with highest frequency was n = 45 (18%), with two configurations: 45II (16%) and 43II + 4I (2%). Other frequencies and configurations observed are presented in Table .
It can be seen in Table that like its parents, PSR 00-161 showed high frequency of abnormal metaphase I cells. Presence of laggards, multiple metaphase plate, and cells with tripolar spindle were observed in this sugarcane hybrid. All anaphase I cells showed irregular meiotic behavior while 72.22% of telophase I cells had one to five lagging chromosomes (Table and Figure ).
Meiosis II
VMC 86-550 (female parent). Normal cells and cells with laggards at prophase II were found to have equal frequencies. One to four laggards were observed in 50% of prophase II cells. Metaphase II cells have almost equal frequencies when normal cells and cells with irregular chromosome behavior were compared. The same trend was observed in anaphase II with one to six chromosomes observed to be lagging behind as most chromosomes migrate towards opposite poles. At telophase II, the majority of cells (56.60%) were observed to have one to four laggards. However, lagging chromosomes were observed to catch up at the end of meiosis II as 86.27% of tetrads are found to be normal (Table and Figure ).
Figure 8. Meiosis II cells of Saccharum officinarum L. (OIO, 1000×). (a–e) var. VMC 86-550: (a) prophase II with laggards and chromosome caught at the cell plate; (b) metaphase II; (c) anaphase II with laggards; (d) telophase II with laggards; (e) normal tetrad cells. (f–j) var. VMC 87-599: (f) prophase II with laggards; (g) metaphase II with laggards; (h) anaphase II with laggards; (i) telophase II with laggards; (j) tetrads with laggards.
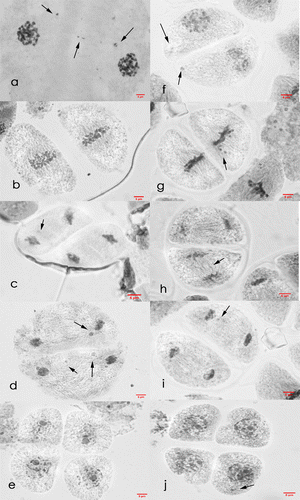
VMC 87-599 (male parent). Most of the lagging chromosomes in meiosis I were observed to catch up with the rest of the migrating chromosomes as most cells in prophase II (55.32%) were normal, and when laggards are present in cell, they were observed to be fewer in number than the laggards present in telophase I. However, most cells at metaphase II, anaphase II, and telophase II were found to have irregular meiotic behavior. More than half (55.42%) of metaphase II cells showed laggard formation and non-congressional alignment of chromosomes, 76.47% of anaphase II cells were observed to have two to five laggards while 56.76% of cells at telophase II have one to four laggards. Most lagging chromosomes observed at telophase II fail to catch up with other migrating chromosomes as 64.81% of tetrads formed were found to have one to three non-aligned chromosomes in the main nucleus of the daughter cells (Table and Figure ).
PSR 00-161 (hybrid). No meiosis II cells were observed in PSR 00-161. It is possible that flowers collected are young and at early stages of meiosis. Sample collection was done on 4–7 November which is the hybridization season at PHILSURIN station in Victorias, Negros. PSR 00-161 is a sugarcane variety known to exhibit flowering during hybridization season when planted in high land. Most sugarcane varieties included in this study were collected at the tassel source of PHILSURIN which is in a highland location. However, PSR 00-161 samples were collected from the germplasm collection which is situated in a lowland area, near PHILSURIN station. PSR 00-161 is known to exhibit late flowering behavior when planted in low land or flower induction maybe absent depending on the climate and soil condition of the area.
PSR 00-34 (VMC 84-194 × PHIL 89-43)
Meiosis I
VMC 84-194 (female parent). This variety showed 15 chromosomal configurations at diakinesis with n = 58 as the highest gametic chromosome number at 10% occurrence and n = 43 as the lowest chromosome number that has a frequency of 2% (Table ). Cells with n = 53 had the highest frequency among all chromosome numbers observed with 18%. All cells were observed to exhibit normal bivalent formation with 76% of diakinesis cells were found to have a chromosomal range of n = 50–58.
Table 8. Chromosome configurations at diakinesis observed in Saccharum officinarum L. var. VMC 84-194, PHIL 89-43, and PSR 00-34.
Though all cells showed normal bivalent formation at diakinesis, 69.37% of cells at metaphase I were found to have irregular meiotic behavior such as lagging chromosomes and presence of multiple metaphase plate. At anaphase I, all cells were found to have one to nine laggards and this high laggard frequency was still observed in telophase I as 80% of cells at the this stage have one to seven lagging chromosomes (Table and Figure ).
Figure 9. Meiosis I cells of Saccharum officinarum L. (OIO, 1000×): (a–d) var. VMC 84-194: (a) binucleated cell with 53II; (b) non-congression at metaphase I; (c) anaphase I with laggards; (d) telophase I with laggards. (e–h) var. PHIL 89-43: (e) diakinesis with 45II; (f) metaphase I with laggards; (g) anaphase I with laggards; (h) telophase I with laggards. (i–l) var. PSR 00-34: (i) diakinesis with 50II; (j) metaphase I with tripolar spindle; (k) anaphase I with laggards; (l) telophase I with laggards.
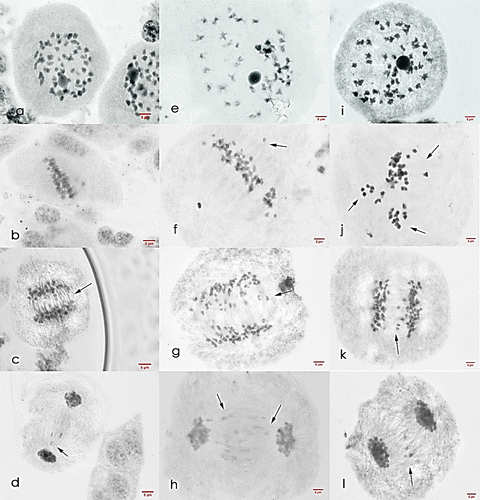
PHIL 89-43 (male parent). This sugarcane is a hybrid produced from the cross between Q 102 and PHIL 71-15 (Engle et al. Citation2012). Its diakinesis cells were found to have a gametic chromosome number of n = 41–57. The majority of PHIL 89-43 (86.78%) cells have a chromosome number of n = 41–49 and cells with n = 45 had the highest frequency of 18.87%, which showed one chromosomal configuration of 45II (Table ).
Most cells of PHIL 89-43 at metaphase I were observed to have irregular meiotic behavior, specifically non-synchronous alignment of chromosomes at the equatorial plate and the presence of a multiple metaphase plate. Most anaphase I cells (98%) were found to have six to 15 lagging chromosomes while telophase I cells also showed high laggard frequency. One to nine laggards were observed in 89.33% of PHIL 89-43 telophase I cells (Table and Figure ).
PSR 00-34 (hybrid). Diakinesis cells of the hybrid, when analyzed, showed 15 chromosomal configurations and a gametic chromosomal range of 2n = 40–58. Cells with n = 50 had the highest frequency of 17.31%. Most cells exhibited normal bivalent formation but the formation of two to six univalents was also observed (Table ).
Table shows that metaphase I of the hybrid exhibited high frequencies of irregular meiotic behavior, with 83.93% of its cells observed to have laggards and non-congressional alignment of chromosomes at the metaphase plate. All anaphase I cells of the hybrid were observed to have two to 11 lagging chromosomes. High frequencies of laggard occurrence were also observed in telophase I cells as 77.78% of cells were found to have at least one to five laggards (Figure ).
Meiosis II
VMC 84-194 (female parent). Most laggards that were observed in telophase I caught up with other migrating chromosomes, as the majority of cells at prophase II were found to be normal (Table ). A high frequency of cells with normal meiotic behavior was also observed in metaphase II but 75% of cells at anaphase II have one to three laggards. However, lagging chromosomes at anaphase II were able to catch up, as 63.64% of cells at telophase II were found to have normal chromosome migration. The majority of tetrads produced (94%) at the end of meiosis II were observed to be normal. Figure shows the representative photomicrographs for each stage of meiosis II.
Figure 10. Meiosis II cells of Saccharum officinarum L. (OIO, 1000×): (a–e) var. VMC 84-194: (a) prophase II with laggards; (b) metaphase II; (c) anaphase II with laggards; (d) telophase II; (e) normal tetrad cells. (f–j) var. PHIL 89-43: (f) prophase II with laggards; (g) non-congression at metaphase II; (h) anaphase II; (i) telophase II with laggards; (j) tetrads with laggards and chromosomes caught at the cell plate. (k–o) var. PSR 00-34: (k) prophase II with laggards; (l) metaphase II with laggards; (m) anaphase II with laggards; (n) telophase II with laggard; (o) normal tetrad cells.
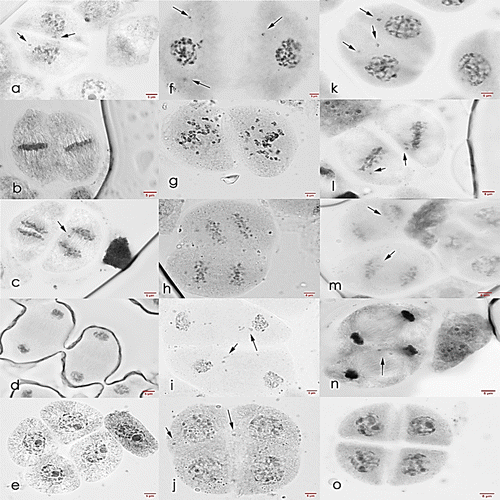
PHIL 89-43 (male parent). Most laggards at telophase I failed to catch up with other segregating chromosomes, as 54.29% of prophase II cells were seen to have one to five non-aligned chromosomes. Most cells at metaphase II, anaphase II, and telophase II also showed high frequencies of irregular meiotic behavior, but the majority of tetrads formed were observed to be normal (Table and Figure ).
PSR 00-34 (hybrid). In Table it can be seen that most laggards in telophase I did not catch up with other segregating chromosomes, as 66.67% of prophase II cells were observed to have one to three non-aligned chromosomes. Like its parents, PSR 00-34 exhibited high frequencies of irregular meiotic behavior at metaphase II, anaphase II, and telophase II. When the products of meiosis II were observed, only 11.54% of tetrads have at most one lagging chromosome. Figure presents the photomicrograph of PSR 00-34 cells at meiosis II.
Pollen fertility
Pollen fertility data were provided by PHILSURIN and were obtained through the I2KI test. Table shows the fertility ratings being used in PHILSURIN while Table shows the pollen fertility data of all sugarcane varieties examined in this study.
Table 9. Pollen fertility ratings used in Philippine Sugar Research Institute (PHILSURIN).
Table 10. Pollen fertility in some recommended and released Philippine varieties of sugarcane (Saccharum officinarum L.).
Discussion
Chromosome transmission
Chromosome transmission such as n+n, 2n+n, n+2n, and 2n+2n are reported in sugarcane (Huang et al. Citation2015). Escote (Citation1978) reported n+n transmission in the hybrids of S. officinarum and S. spontaneum. Melloni et al. (Citation2014) also reported the same type of chromosome transmission in the hybrids derived from the cross between RB855465 (2n = 100–130), a commercial sugarcane variety, and S. spontaneum (GlagaH; 2n = 112). Moreover, a molecular cytogenetic study by D’Hont et al. (Citation1996) on the modern sugarcane varieties (F1 of S. officinarum and S. spontaneum) reported n+n transmission instead of the 2n+n that was usually described in early cytogenetic studies of sugarcane. However, in Citation2010, Piperidis et al. performed genomic in situ hybridization in modern sugarcane varieties that were derived from the cross between S. officinarum and S. spontaneum, and reported the occurrence of 2n+n transmission in the back crosses of these two species. In this study, chromosome transmission in all hybrids of interest was found to be n+n, though some hybrids have pollen mother cells that arise from the fertilization of aneuploid gametes. Varieties with unidentified male parents were assumed to also have n+n transmission, as indicated by their chromosome number and observed meiotic behavior. If there was 2n+n transmission in varieties with unidentified male parents, the chromosome numbers of VMC 95-09 and PSR 00-343 must be in the range of 2n = 120–200. However, this high chromosome number was not observed as VMC 95-09 has a chromosomal range of 2n = 80–104 (n = 40–52) while PSR 00-343 has 2n = 80–106 (n = 40–53).
VMC 95-09 (Q 90 × Unidentified)
Given the chromosome number observed in the hybrid, VMC 95-09 (2n = 80–104; n = 40–52), and chromosome number of the female parent, Q 90 (2n = 80–106; n = 40–53), the male parent could have a chromosome number 2n = 80–102, and transmitted n = 40–51 of its chromosomes to VMC 95-09. Moreover, normal bivalent formation was observed in VMC 95-09 and this implies autosyndetic pairing.
PSR 97-45 (VMC 74-292 × CP 72-1312)
The female parent, VMC 74-292, was observed to have a gametic range of n = 45–53, while CP 72-1312, the male parent, had n = 40–54. When these gametes combine during fertilization, it should result in the range 2n = 85–107. PSR 97-45 has a chromosomal range of 2n = 80–110 (n = 40–55), which indicated that it had cells with fewer or more chromosomes than the expected chromosomal range of the hybrid. This observation can be attributed to the formation of aneuploid gametes in the parents as both male and female parents were observed to have high frequency of abnormal meiotic behavior. Lagging chromosomes were observed in both VMC 74-292 and CP 72-1312, which were mostly late disjoining bivalents. Some of these laggards were eventually caught in the cell plate and lost by the cell. On the other hand, irregular chromosome alignment at meiosis II may lead to non-disjunction of sister chromatids and result to the formation of a nucleus with an additional chromosome set (Andreuzza and Siddiqi Citation2008). This phenomenon was particularly observed in male meiocytes as proper choromosomal alignment at meiosis II and proper spindle positioning is essential for correct chromosome segregation and ploidy reduction (Storme and Geelen Citation2011). Though there is a possibility that hybrids with odd chromosome numbers can be formed, no PSR 97-45 diakinesis cell was observed to have such behavior. Plants are known to have higher tolerance to changes in chromosome number, thus many plants are observed to be polyploid, e.g. S. officinarum. Polyploid plants are known to have irregular meiosis unless chromosomes exhibit normal pairing and behave like diploids (Fletcher et al. Citation2012). Thus, it is more favorable in plants to have an even chromosome number, so regular bivalent formation can proceed. Polyploid plants with odd numbers are known to be sterile because of genomic imbalance and the chromosomes cannot be divided into equal sets at the reduction stage of meiosis (Fletcher et al. Citation2012).
Univalents and clumped chromosomes were found to be present in the hybrid. Univalents in PSR 97-45 existed in pairs and were usually parallel to each other (Figure (i)). This may indicate that univalents seen were actually due to early disjunction of chromosome pairs instead of complete failure in bivalent formation. On the other hand, the clumped chromosomes in the hybrid may be the chromosomes from the male parent, CP 72-1312, as this behavior was observed in this variety at relatively high frequency (35%) rather than intergenomic association. With these findings, it can be suggested that chromosome pairing in PSR 97-45 is autosyndetic because of high frequency of bivalent formation.
PSR 00-343 (VMC 84-947 × Unidentified)
VMC 84-947, which served as the female parent, had a gametic range of n = 40–53 with 83.64% of its cells with n = 40–49. If there is n+n transmission between the two parents, the male parent of PSR 00-343 could have a gametic chromosome number of n = 40–45, given that 74.51% of PSR 00-343 diakinesis cells showed a chromosome number of 2n = 80–94 (n = 40–47). Since the female parent’s chromosome number also existed in this range, it was possible that gametes with a chromosome number of n = 50–53 could have been fertilized by the male parent and given rise to hybrid cells with a chromosome number of 2n = 90–100 (n = 45–50). Other chromosome numbers observed in the hybrid, 2n = 102–106 (n = 51–53), could have been products of fertilization of aneuploid gametes as irregular meiotic behavior was observed in the female parent.
Normal bivalent formation was prevalent in PSR 00-343 with only 9.8% of cells observed to have univalents. Since all univalents seen were found to be parallel or close to each other, it is possible that they were formed due to early disjunction of the chromosome pairs.
PSR 00-161 (VMC 86-550 × VMC 87-599)
VMC 86-550, which served as the female parent, had a gametic range of n = 47–60, while VMC 87-599, the male parent, had 2n = 40–57. If there was an equal chromosome contribution between the parents, the hybrid, PSR 00-161, should have a chromosome number of 2n = 87–117. PSR 00-161 showed diakinesis cells with chromosome number of 2n = 80–116 (n = 40–58). Like in PSR 97-45, hybrid cells with higher or lower chromosome number than the expected range were observed in PSR 00-161. This observation may be due to the fertilization of aneuploid gametes from both parents, as VMC 86-550 and VMC 87-599 were seen to have irregular meiosis. This erratic meiotic behavior may have given rise to aneuploid gametes or gametes with different chromosome number.
Moreover, no odd chromosome number was observed in PSR 00-161, as in PSR 97-45, as plants with odd chromosome numbers are known to be sterile. The majority of cells in PSR 00-161 exhibited normal bivalent formation and few univalents were observed.
PSR 00-34 (VMC 84-194 × PHIL 89-43).
The female parent, VMC 84-194, had diakinetic cells with n = 43–58, while the male parent, PHIL 89-43, had n = 41–57. When haploid cells are combined, it should give rise to a variety with a chromosome number of 2n = 84–115. Since PSR 00-34 showed cells with 2n = 80–116 (n = 40–58), there is a high possibility that aneuploid gametes from both parents were formed, as high frequencies of irregular meiotic behavior were observed both in VMC 84-194 and PHIL 89-43. Irregular chromosome alignment was observed in meiosis I and meiosis II in both parents, which had led to formation of daughter cells with additional or reduced chromosome sets in their nuclei (Andreuzza and Siddiqi Citation2008).
Meiotic behavior
All sugarcane varieties observed in the study were found to exhibit high frequencies of abnormality in meiotic behavior. According to Shu et al. (Citation2012), chromosomal aberrations are mainly due to mutations such as duplication, deletion, inversion, translocation and other phenomena that may have a large, little or no consequence in the phenotype of an organism. Moreover, abnormalities in chromosome segregation are also significant in producing chromosomal aberrations such as genomic instability and aneuploidy.
Multipolar metaphase plate formation was found to be present in all sugarcane varieties used in the study. This observation indicated abnormality in the spindle of the cell (Escote Citation1978). Vasek in Citation1962 reported this phenomenon in Clarkia exilis, a small herbaceous plant found in the western part of North America. This abnormality observed in the herb resulted in abnormal telophase chromosome segregation, thus resulted in micronuclei formation and reduced pollen fertility. Segregation data in the F2 and F3 progenies of C. exilis showed that this abnormality was caused by a recessive gene. Its persistence in the natural population may be due to its ability to produce partial sterility and may be associated with a relatively strong selective advantage (Escote Citation1978). If abnormal spindles have convergent poles, normal, fertile gametes can be formed. If poles are divergent, chromosome elimination may occur as poles with fewer chromosomes will not proceed to other meiotic stages.
Laggard occurrence was another chromosomal abnormality observed in metaphase I, anaphase I, telophase I, and in meiosis II of all the varieties studied. Escote (Citation1978) enumerated three factors that could result in the occurrence of laggards in sugarcanes: (1) due to non-synchronous separation of chromosomes because of non-congression or late congression of chromosomes at the metaphase plate; (2) due to late disjunction of bivalents and univalent; and (3) due to the presence of multiple spindles at metaphase. Moreover, since chromosomes were observed to behave as univalents or multivalents in diakinesis of pollen mother cells of some sugarcane varieties, segregation of these chromosome arrangements may also be the cause for laggard formation in the subsequent stages of meiosis.
Some lagging chromosomes seen in the sugarcane varieties observed were eventually lost by the cell as they were caught in the cell plate during cytokinesis, while some were observed as non-aligned chromosomes in prophase II and tetrad cells which may eventually give rise to the formation of micronuclei in meiotic daughter cells. Early cytological studies (Kasha and Kao Citation1970) showed that rapid preferential uniparental chromosome elimination in plants was due to micronuclei formation in mitosis of early hybrids of closely related species. Chromosomes to be eliminated often exhibit non-congression at metaphase and existed as laggards in anaphase (Laurie and Bennet Citation1989). In Citation2005, Gernand et al. identified how chromosomes in the micronucleus were eliminated. After micronucleus formation, enclosed chromosomes underwent heterochromatinization and DNA fragmentations that resulted in the disintegration of the micronucleus. In meiosis, the fate of micronuclei can be diverse. Cells with micronuclei may remain at the tetrad stage or may give rise to microcytes in some plant species (Mendes-Bonato et al. Citation2007). It may also be a disadvantage as during the course of an individual development, pollen grains that contain micronuclei may not be able to develop competitively compared to cells with normal pollen behavior, especially if the genes essential for gamete survival are found in the micronucleus and not on the main nucleus (Elliott Citation1958). Though cells with non-aligned chromosomes at tetrad stage were observed in the sugarcane varieties studied, normal tetrad cells occurred at higher frequencies, which indicated that pollens can develop competitively with each other. However, there is a high probability that the number of chromosomes in each tetrad cell is not the same, thus forming aneuploid cells or cells with different chromosome numbers as a result of irregular meiotic behavior observed in all sugarcane varieties.
Pollen fertility
Among the five hybrids observed, PSR 00-34 and VMC 95-09 had the lowest pollen fertility of 0–9% and 30–39%, respectively. The other three hybrids, PSR 97-45, PSR 00-161, and PSR 00-34, are considered to be semi-fertile, having pollen fertility values of 70–89%. These three varieties can be used as male parents in hybridization programs and if used as female parents, emasculation should be done first.
The observed reduced pollen fertility in the hybrids and their parents appear to be a reflection of their irregular meiotic behavior. However, it is important to note that other environmental factors can also affect pollen fertility that may have contributed to the differences in pollen fertility of all sugarcane varieties studied. Some of these factors include diurnal temperatures (Clements and Awada Citation1967; Adejuwon Citation1988), rainfall amount and distribution (Olaoye Citation1996), decline in day length (Abou-salama Citation1990), and sub-optimal photoperiod (Berding and Hurney Citation2005) that is often accompanied by moisture stress (Kwajaffa and Olaoye Citation2014) and usually observed in tropical countries like Philippines.
Conclusion
The hybrids and their parents showed variable meiotic behavior. Chromosomal configurations at diakinesis mostly showed normal bivalent formation which indicated autosyndetic pairing, between sugarcane chromosomes both in hybrids and parents, rather than allosyndetic manner.
Chromosome transmission in the studied varieties was observed to be n+n as indicated by the chromosomal range observed in the hybrids, 2n = 80–120. The variability observed in the chromosome numbers and meiotic behavior of the modern sugarcane varieties used in the study is high and might reflect genetic variability, since these varieties are grown in more or less uniform environmental conditions. Results gathered can be useful in identifying and choosing best hybrids to use based on their known morphological behavior, tolerance to different environmental stresses, and cytological characteristics, particularly in meiosis. Meiosis is an important process in plants as this determines fertility which is a standard tool in all hybrid improvement programs.
Disclosure statement
No potential conflict of interest was reported by the authors.
Acknowledgement
We are very grateful to the Philippine Sugar Research Institute Foundation Inc. (PHILSURIN). The sugarcane samples used in this study were all collected from their tassel source. This research would not be possible without the help and assistance of Research Station Manager Lyn Luzaran and Ms. Grazie Oquias.
References
- Abou-Salama AM. 1990. Sugarcane pollen viability and seed setting as affected by daylength decline rates and relative humidity [dissertation]. [LSU historical dissertation and theses]. Louisiana: Louisiana State University.
- Adejuwon SA. 1988. An assessment of the patterns of rainfall fluctuations between 1922 and 1985 in Nigeria [ dissertation]. Ile-Ife: Obafemi Awolowo University.
- Alwala S, Kimbeng CA. 2010. Molecular genetic linkage mapping in Saccharum: strategies, resources and achievements. In: Henry RJ, Kole C, editors. Genetics, genomics and breeding of sugarcane ( 2010, August 15). USA: CRC Press. p. 69–96, 300 p.
- Andreuzza S., Siddiqi L. 2008. Spindle positioning, meiotic nonreduction, and polyploidy in plants. PLOS Genet 4 [Internet]. Available from: https://www.ncbi.nlm.nih.gov/pmc/articles/PMC2582683/
- Berding N, Hurney AP. 2005. Flowering and lodging, physiological-based traits affecting cane and sugar cane yield: what do we know of their control mechanisms and how do we manage them? Field Crops Res. 92(2/3):261–275.
- Bremer G. 1924. The cytology of sugarcane. A cytological investigation of some cultivated kinds and their parents. Genetica (The Hague). 6:497–525.
- Clements HF, Awada M. 1967. Experiments on the artificial induction of flowering in sugarcane. Proc Int Soc Sugarcane Technol. 12:795–812.
- D’hont A, Grivet L, Feldmann P, Rao S, Berding N, Glaszmann JC. 1996. Characterisation of the double genome structure of modern sugarcane cultivars (Saccharum spp.) by molecular cytogenetics. Mol Gen Genet. 250(4):405–413.
- Divinagracia NS, Ramirez DA. 1976. The morphology and cytology of Saccharum officinarum indigenous to the Philippines. Philipp J Crop Sci. 1(1):1–25.
- Dutt NL, Rao KS. 1933. Observations on the cytology of sugarcane. Indian J Agric Sci. 3:37–56.
- Elliott FC. 1958. Plant breeding and cytogenetics. New York (NY): McGraw-Hill Boo Company Inc; 395 p.
- Engle LM, Escote LJ, Dela Cruz FS, Sedano MR. 1980. The cytology and morphology of hybrids derived from Hawaiian commercial variety of sugarcane and Saccharum spontaneum from Iloilo. Philipp J Crop Sci. 5(3):95–99.
- Engle LM, Fabillar LL, Lumbres GV, Sedano MR. 1979. The cytogenetics of hybrids between a cultivated sugarcane and Talahib. Philipp J Crop Sci. 4(2/3):63–71.
- Engle LM, Luzaran RT, Villariez HP, Untal CH. 2012 Aug. The genealogy of PHILSURIN recommended and released sugarcane varieties. Paper Presented at: 2012 PHILSUTECH annual convention. Waterfront Hotel; Cebu City, Philippines.
- Engle LM, Sedano MR, Dela Cruz FS. 1980. The cytology and morphology of hybrids derived from open-pollinated Saccahrum officinarum and S. spontaneum F1 hybrids. Philipp J Crop Sci. 5(4):130–133.
- Escote LJ. 1978. Cytological and morphological study of Saccharum spontaneum L. and related genera in the Philippines and Saccharum officinarum and Saccharum spontaneum hybrids [ Unpublished MS thesis (Genetics)]. Los Baños: University of the Philippines; p. 154 [in English].
- Fletcher H, Hickey I, Winter P. 2012. Instant notes in genetics. 3rd ed. New York: Taylor and Francis Group; p. 384.
- Gernand D, Rutten T, Varshney A, Rubotsova M, Prodanovic S, Brüß C, Kumlehn J, Matzk F, Houben A. 2005. Uniparental chromosomes elimination at mitosis and interphase in wheat and pearl millet crosses involves micronucleus formation, progressive, heterochromatinization, and DNA fragmentation. Plant Cell. 17(9):2431–2438. doi:10.1105/tpc.105.034249.
- Heinz, DJ. 1991. Sugarcane cytogenetics. In: Tsuchiya T, Gupta PK, editors. Chromosome engineering in plants: genetics, breeding, evolution part B (Vol. 2, Part 2). Amsterdam: Elsevier Science. p. 279–292, 647 p.
- Huang Y, Wu J, Wang P, Lin Y, Fu C, Deng Z, Wang Q, Li Q, Chen R, Zhang M. 2015. Characterization of chromosome inheritance of the intergeneric BC2 and BC3 progeny between Saccharum spp. and Erianthus arundinaceus. [Internet]. Available from: https://journals.plos.org/plosone/article?id=10.1371/journal.pone.0133722#pone.0133722.ref021
- Investment Opportunity Sugarcane Plantation. 2011. [Internet]. Available from: https://www.philexport.ph/c/document_library/get_file?uuid=8f3ab5f7-ae8a-48f0-804b-9d2f47419a6b&groupId=127524
- Jagathesan D, Sreenivasan TV, Ratnambal MJ. 1970. Chromosome numbers in noble sugarcane. Indian J Heredity. 2:89–96.
- Kasha KJ, Kao KN. 1970. High frequency of haploid production in barley (Hordeum vulagre L.). Nature. 225:874–875.10.1038/225874a0
- Kwajaffa AM, Olaoye G. 2014. Flowering behavior, pollen fertility, and relationship of flowering with cane yield and sucrose accumulation among sugarcane germplasm accessions in a savanna ecology in Nigeria. Int J Curr Agric Res 3(12):104–108 [Internet]. Available from: https://wrpjournals.com/sites/default/files/issues-pdf/1067.pdf
- Laurie DA, Bennett MD. 1989. The timing of chromosome elimination in hexaploid wheat x maize crosses. Genome. 32:953–961.10.1139/g89-537
- Melloni MNG, Melloni MLG, Rossini L, Creste S, Xavier MA, Landell MGA, Heslop-Harrson JS, Schwarzacher T, Bailey JP. 2014. Sugarcane and characterization of Saccaharum hybrids by molecular cytogenetics with Natalia Melloni. [Internet]. Available from: https://molcyt.org/2014/08/05/sugar-cane-and-characterization-of-saccharum-hybrids-by-molecular-cytogenetics-with-natalia-melloni/
- Mendes-Bonato AB, Pagliarini MS, Valle CB. 2007. Meiotic arrest compromises pollen fertility in an interspecific hybrid between Bracharia ruziziensis x Bracharia decumbens (Poaceae:paniceae). Braz Arch Boil Technol. 50:831–837 [Internet]. Available from: https://www.scielo.br/scielo.php?script=sci_arttext&pid=S1516-89132007000500011 10.1590/S1516-89132007000500011
- Olaoye G. 1996. Studies on flowering in sugarcane in savanna ecology in Nigeria. I. Relationship between pollen fertility and seed set. Niger J Genet XI: 60–65.
- Piperidis G, D’hont A. 2001. Chromosome composition analysis of various Saccharum interspecific hybrids by genomic in situ hybridization GISH. Proc Int Soc Technol. 24:565–566.
- Piperidis G, Piperidis N, D’hont, A. 2010. Molecular cytogenetic investigation of chromosome composition and transmission in sugarcane. Mol Genet Genomics. 284(1):65–73. doi:10.1007/s00438-010-0546-3
- Price S. 1960. Cytological studies in Saccahrum and allied genera. VI. Chromosome numbers in S. officinarum and other noble sugarcanes. Hawaii Plant Rec. 56:183–194.
- Shu QY, Forster BP, Nakagawa H. 2012. Plant mutation breeding and biotechnology. New York (NY): CAB International and FAO; p. 608.10.1079/9781780640853.0000
- Storme ND, Geelen D. 2011. The Arabidopsis mutant Jason produces unreduced first division restitution male gametes through parallel/fused spindle mechanism in meiosis II. Plant Physiol 155:1403–1415 [Internet]. Available from: https://www.plantphysiol.org/content/155/3/1403.full 10.1104/pp.110.170415
- Sugar Regulatory Administration. 2012. History. [Internet]. Available from: https://www.sra.gov.ph/about-us/history/
- Sugarcane Industry Road Map (2011–2016). 2012. [Internet]. Available from: https://www.da.gov.ph/index.php/2012-03-27-12-03-56/2012-04-13-12-40-39
- Vasek FC. 1962. “Multiple spindle” – a meiotic irregularity in Clarkia exilis. Am J Bot. 49(5):536–539.10.2307/2439426