Abstract
The developmental events in the periplasmic space and cytoplasm of microspores of Chamaedorea microspadix during sporoderm ontogeny have been traced in detail from cytokinesis and early tetrad stage to the formation of intine in mature two-celled pollen grains. Several pre-pattern structures as seen in the periplasmic space of early microspores (dark-contrasted globules, ribbon-like striped structures, strings of beads, spherical units, radially oriented columns of spherical units, rod-like units), can easily be read as different types of micelles (spherical, cylindrical micelles, so-called segmented worms) and their intermediate forms (strings, perforated filaments, columns of spherical micelles). Funnel-like procolumellae, arranged as complexes of bent units, appear next at the middle tetrad stage. These “funnels” possibly correspond to double gyroid phase, typical for a diblock copolymer system, adapted to constraints of the narrow periplasmic space. The foot layer and few endexine lamellae with central white lines appear as laminate (“neat”) micelles, with typical gaps between the laminae; they emerge through intermediate forms of micelles as strings of spherical micelles and/or perforated laminae. Strings are probably a preferential intermediate form of micelle formation in the course of exine development in Chamaedorea: they emerge at every ontogenetic step and participate in the appearance of every exine layer. The first (channelled) layer of the two-layered intine beneath the aperture appears probably on the base of cylindrical micelles. The sequence of the sporoderm layer formation can be understood as a sequence of self-assembling mesophases (and their intermediate forms) in the colloidal system of the microspore periplasmic space, unfolding through the ontogenetic time.
The ideas of Thompson (Citation1917) on the universal importance of physical and chemical interactions for pattern formation in nature paved a new way of thinking for biologists/palynologists, which was adopted by Wodehouse (Citation1935) and Heslop-Harrison (Citation1968, Citation1971a , Citation b , Citation1972), and worked out by Gerasimova-Navashina (Citation1973), Sheldon and Dickinson (Citation1983), Dickinson and Sheldon (Citation1986), Gabarayeva (Citation1990, Citation1993), Van Uffelen (Citation1991), Hemsley et al. (Citation1992, Citation1994, Citation1996, Citation1998, Citation2000, Citation2004), Collinson et al. (Citation1993), Scott (Citation1994), Southworth and Jernstedt (Citation1995), Gabarayeva and Hemsley (Citation2006), Hemsley and Gabarayeva (Citation2007) and Blackmore et al. (Citation2007). As seen from this list, the initial idea has increasing adherents, and each author introduces new ideas and develops previous ones.
The cellular process of microsporogenesis in monocotyledons, focused on the type of cytokinesis, was illustrated and analysed by Furness and Rudall (Citation1999). Numerous intraspecific variations in aperture pattern were shown for Arecaceae (Harley & Baker, Citation2001; Harley, Citation2004) – the phenomenon, shown for many other species and regarded as one of nature's regularities (Pozhidaev, Citation1998, Citation2000, Citation2002). Molecular phylogeny of the genus Chamaedorea was examined by Thomas et al. (Citation2006). Pollen morphology, descriptions, comparison with recent palm representatives, their systematics and evolutionary relationships of Chamaedorea were studied and reviewed by Harley (Citation1990) and Ferguson and Harley (Citation1993). In Chamaedorea microspadix Burret, cytokinesis is simultaneous and achieved by cell plates growing centripetally from the edges of each of the cleavage planes; the resulting tetrads range from tetragonal to symmetric or asymmetric tetrahedral with occasional rhomboidal tetrads (Nadot et al., Citation2006). Pollen grains are monosulcate and occasionally trichotomosulcate.
The investigations of sporoderm ontogeny are particularly helpful with respect to identification and mode of establishment of different layers, especially if the endexine is thin and poorly discernible in the mature pollen wall. However, no developmental studies on Chamaedorea sporoderm have been undertaken until now. The mode of the exine pattern emergence in C. microspadix, operating during development in response to subtle variations of the conditions within the plasma membrane glycocalyx, is the main point of interest in this paper, the more so that all our previous ontogenetic studies have dealt with dicotyledons (e.g., Gabarayeva, Citation1986, Citation1987, Citation1991b, Citation1995; Gabarayeva & El-Ghazaly, Citation1997; Gabarayeva & Grigorjeva, Citation2003; Gabarayeva et al., Citation2009a, b).
Materials and methods
Flower buds of Chamaedorea microspadix were collected from the greenhouses of Komarov Botanical Institute, St. Petersburg, for six years to obtain all the main developmental stages for study. Stamens were fixed in 3% glutaraldehyde and 2.5% sucrose in 0.1 M cacodylate buffer with the addition of lanthanum nitrate (1%) for better preservation of the plasma membrane glycocalyx, at pH 7.4, 20°C, 24 h. After post-fixation in 2% osmium tetroxide (20°C, 4 h) and acetone dehydration, the samples were embedded in a mixture of Epon and Araldite. Ultrathin sections were contrasted with a saturated solution of uranyl acetate in ethanol and 0.2% lead citrate. Sections were examined with a Hitachi H-600 transmission electron microscope.
Fresh mature pollen grains for scanning electron microscopy (SEM) were mounted on stubs, coated with gold/palladium and examined with a JEOL JSM-6390 instrument.
To reveal the chemical composition of the substance associated with double-membrane organelles, and the substance of intermediate micelle form (strings) in the cytoplasm of the microspores, which differed from normal developmental pathways, the enzymes pronase E (Calbiochem, Streptomyces griesus B grade) and lipase (Sigma Chemical Co., Type I – Wheat germ) were applied (Dashek et al., Citation1971; Belitser et al., Citation1982).
Results
Pre-tetrad stages
Before meiosis, sporogenous cells are connected with cytomictic channels predestined to synchronise the beginning of meiosis (A). The walls of adjacent cells are separated by a middle plate. The cytoplasm contains cisternae of ER, mitochondria, hydrolytic vacuoles (cytosomes), active dictyosomes and large nuclei. At the end of meiosis, four microspores of a tetrad pass through cytokinesis and deposition of callose between the cells, with cell plates growing centripetally from the edges (B). The microspore cytoplasm is full of Golgi vesicles, tubular ER, large plastids with plastoglobules and lamellar systems, double-membrane organelles with dark-contrasted inclusions (which can be the second generation of small plastids or dedifferentiated mitochondria), osmiophilic filaments in the cytoplasm (B) and small vacuoles.
Figure 1. Pre-tetrad stages in Chamaedorea microspadix. A. Sporogenous cells are connected with cytomictic channels (arrows). The walls (cw) of adjacent cells are separated by a middle plate (mp). The cytoplasm of sporogenous cells (csc) contains cisternae of ER (er), mitochondria (m), and hydrolytic vacuoles (cytosomes, cs). B. The end of cytokinesis. Deposition of callose (c) between the cells (arrows). Golgi vesicles (gv), tubular ER (er), large plastids (p) with plastoglobules (pg) and lamellar systems (lam), double-membrane organelles (dm) with dark-contrasted inclusions (probably other generation of small plastids or dedifferentiated mitochondria), osmiophilic filaments in the cytoplasm (arrowheads) are recognisable in the cytoplasm. Scale bars – 0.5 μm.
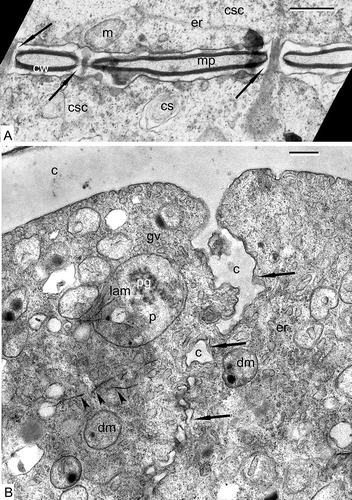
Tetrad stages
Young tetrad stage
On the completion of meiosis and cytokinesis, the four young microspores encompassed within the callose envelope do not show any signs of the cell surface glycocalyx on the plasma membrane. However, soon dark-contrasted lipoid globules appear on the cell surface (A), and the cytoplasm includes a large nucleus, ER cisternae, double-membrane organelles with dark-contrasted inclusions, large plastids with osmiophilic globules, lipid droplets and small vacuoles. Somewhat later, a layer of the glycocalyx appears on the plasma membrane as a result of the activity of many dictyosomes, located in the cortical microspore cytoplasm (B). This layer is far from being homogeneous. It consists of spherical and close-to-spherical elements on the surface (C) and ribbon-like striped underlining structures, parallel to the plasma membrane (C).
Figure 2. Early tetrad stages in Chamaedorea microspadix microspores. A. Dark-contrasted lipoid globules appear on the cell surface (arrowheads), which we interpret as spherical micelles. B. A layer of the glycocalyx (g) appears on the plasma membrane as a result of activity of many Golgi stacks (gs) in the cortical microspore cytoplasm. C. The glycocalyx consists of spherical and close-to-spherical elements (= spherical micelles, arrowheads) and of ribbon-like striped structures (worm-like micelles, white arrows). D, E. Later, the glycocalyx layer (g) looks as if it is comprised of spherical units with a dark core and light halo (spherical micelles, arrowheads). F. Some of these roundish units (spherical micelles) become arranged into radially oriented columns (transition to cylindrical micelles, arrowheads); many Golgi vesicles (gv) are recognisable in the peripheral cytoplasm. G. At this next ontogenetic step, radially oriented rod-like units are well pronounced inside the glycocalyx (cylindrical micelles, arrowheads) together with small chains of vesicles alike strings of beads (strings, a transitional form of micelles, arrows). Legend to all figures: c – callose, dm – double-membrane organelle with inclusion, er – endoplasmic reticulum, g – glycocalyx (layer), mc – microspore cytoplasm, n – nucleus, nu – nucleolus, pl – plasma membrane. Scale bars – 0.3 μm (A), 1.0 μm (B), 0.25 μm (C–G).
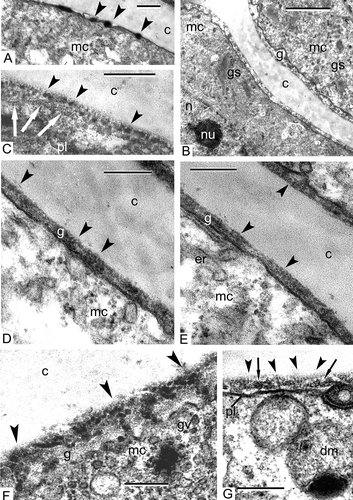
At a later stage, the glycocalyx layer consists entirely of spherical units with a dark core and a light halo (D, E). This changes when some of these roundish units become arranged into radially oriented columns (F). The peripheral cytoplasm is full of Golgi vesicles, which deliver glycoproteins to the glycocalyx. At the next ontogenetic step, radially oriented rod-like units are well pronounced structures inside the glycocalyx (G), together with small chains of vesicles resembling strings of beads (G).
Middle tetrad stage
A survey of a tetrad at the middle tetrad stage (A) shows many organelles and inclusions in the cytoplasm: large plastids with spherical plastoglobules and clusters of fine-fibrillar osmiophilic substances; double-membrane organelles (arrows indicate the second generation of smaller plastids or non-differentiated mitochondria without any signs of cristae, with strongly osmiophilic inclusions) and many vacuoles, some with a fibrillar substance, probably lysosomes; and lipid globules, often in association with the plasma membrane. Double-membrane organelles with peripherally disposed osmiophilic granules are, as will be shown below, a persistent and typical character of Chamaedorea microspadix microspores. They seem to play an important role in pollen wall biosynthesis. At the distal poles of the microspores, in the position of future apertures, the plasma membrane invaginates. This results in the appearance of a cavity at these points and the impending accumulation of the glycocalyx material on the plasma membrane surface (A). Thin initial columellae are seen through the glycocalyx layer. At high magnifications, these columellae show a specific form: their outlines on sections resemble the letter “Y” (B, C). Another new structure is the initial foot layer, which is still discontinuous and evidently formed by strings of small vesicles (B, D). The detailed examination of the initial columellae reveals a funnel-like three-dimensional form. It becomes clear that every columella consists of several bent units which are close to each other proximally, in the vicinity of the plasmalemma (D) but separate from each other in the fan-like manner distally (D, E). The borders of every two neighbouring “funnels” connect over a period of time, forming the reticulate tectum. The borders of funnel-like columellae, consisting of several bent rod-units, are discernible in slightly more thick sections, which, although some sharpness of focus is lost, give more three-dimensional information. Every rod has a dark core and light outlines (E), but in some columellae, these subunits acquire the opposite contrast and show a light core and dark outlines (F–H). It is quite probable that the initial sporopollenin (SP) accumulation has already begun at this stage.
Figure 3. Middle tetrad stage in Chamaedorea microspadix microspores. A. A survey of a tetrad at the middle tetrad stage. Large plastids with single spherical plastoglobule and clusters of fine-fibrillar osmiophilic substance (p), second generation of smaller plastids (or non-differentiated mitochondria without any signs of cristae) with osmiophilic inclusions (arrows), lipid globules (lg) and lysosomes (ls) are reconisable in the cytoplasm. At distal poles of the microspores, where future apertures appear, the plasma membrane invaginations form cavities impeding the accumulation of the glycocalyx (asterisks). Thin initial columellae are seen through the glycocalyx layer. B, C. At higher magnification, these columellae show the form of the letter “Y” (so-called gyroid phase of micelles, arrows). The initial foot layer (which is still discontinuous) is formed by a chain of tiny vesicles (by strings, arrowheads in B). D. The detailed examination of the columellae reveals their funnel-like form: every columella consists of several rod-like units (cylindrical micelles), which are close to each other in the vicinity of the plasmalemma (arrows) and separate from each other on the fan-like manner distally (arrowheads). E. The borders of funnel-like columellae consist of several bent rods (every rod-micelle has a dark core and light outlines – black and white arrows). F–H. The details of rod-micelles, comprising the columellae, are discernible in slightly thicker sections. Note the contrast reversal of rods-micelles: now they have a light core and dark outlines (E, F – black and white arrows, G – arrows, H – white arrows). This seems to correspond to the reverse of normal micelles to inside-out micelles. Several ring-like units in H (spherical micelles, white arrows) are components of the rod (cylindrical micelle, black arrow). Legend to all figures: c – callose, fl – foot layer, g – glycocalyx, lg – lipid globules, mc – microspore cytoplasm, pl – plasma membrane, v – vacuole. Scale bars – 1.0 μm (A), 0.25 μm (B–F), 0.1 μm (G, H).
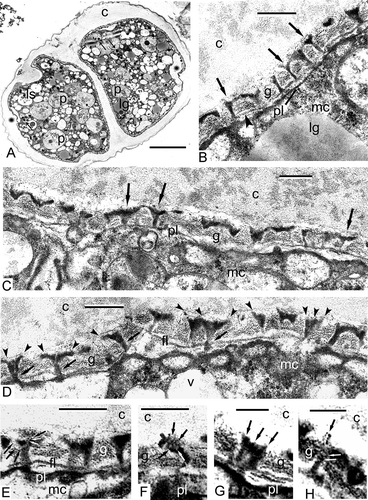
Late tetrad stage
At this stage the accumulation of SP is intensified revealing the pattern of the primexine with its columellae, tectum and foot layer (A, B). Where sectioned obliquely (A, to the right, 4C), the primexine shows a reticulate pattern. In the area of the future aperture (A, left upper part), most of the ectexine is absent. Microspores contain a large nucleus with a nucleolus, large plastids with typical plastoglobules, double-membrane organelles with dark-contrasted inclusions, active dictyosomes pinching off vesicles, and small vacuoles (A). The foot layer becomes thicker, but is still segmented (D).
Figure 4. Late tetrad stage in Chamaedorea microspadix microspores. A, B. At this stage, the accumulation of sporopollenin is intensified revealing the pattern of the primexine with its columellae (co), tectum (t) and the foot layer (fl). Where sectioned obliquely (A, asterisk), the primexine shows a reticulate pattern. In the area of the future aperture (A, arrow), most of the ectexine is absent. Microspores contain a large nucleus (n) with a nucleolus (nu), large plastids (p) with typical plastoglobules, double-membrane organelles (dm) with dark-contrasted inclusions, active dictyosomes (Golgi stacks, gs) pinching off Golgi vesicles (gv), obliquely sectioned exine shows a reticulate pattern (rp). D. The foot layer becomes thicker (arrow). Legend to all figures: c – callose, co – columellae, er – endoplasmic reticulum, g – glycocalyx, mc – microspore cytoplasm, pl – plasma membrane, t – tectum. Scale bars – 1 μm (A), 0.5 μm (B–D).
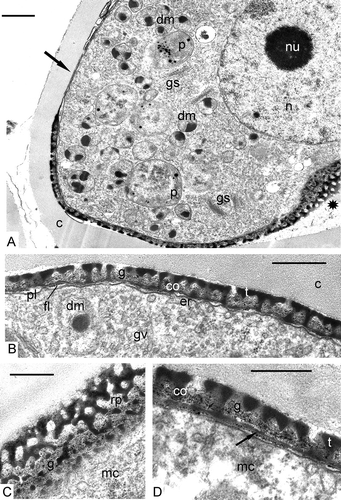
Somewhat later, the first endexine lamella appears as a very thin layer (A). Soon it becomes more pronounced (B) and the next lamellae appear discontinued as a string of tiny vesicles or perforated lamina in the process of endexine formation (B). After additional SP accumulation a typical “white line” is recognisable within the first endexine lamella (C), while the second lamella is still in the process of formation (C).
Figure 5. Late tetrad stage in progress in Chamaedorea microspadix microspores. A. The first endexine lamella has appeared (arrows). The cytoplasm of a microspore contains large plastids (p), double-membrane organelles with dark-contrasted inclusions (dm), Golgi stacks (gs), lysosomes (ls), lipid globules (lg), cisternae of endoplasmic reticulum (er), and vacuoles (v). B. The first endexine lamella becomes more pronounced (arrowheads), and next lamellae appear in the process of endexine formation (arrow). C. After additional sporopollenin accumulation, a typical “white line” is recognisable within the first endexine lamella (white arrow), while the second lamella is still in the process of formation (arrowhead). c – callose, co – columella, fl – foot layer, gv – Golgi vesicles, mc – microspore cytoplasm, pl – plasma membrane, t – tectum. Scale bars – 0.25 μm.
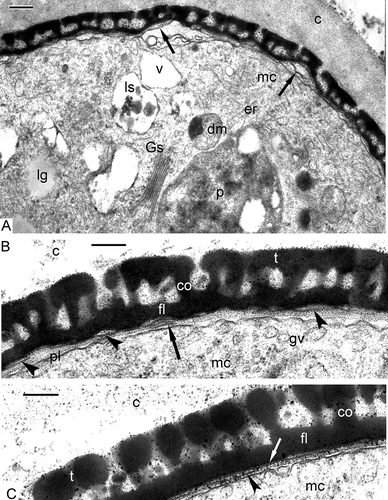
Interestingly, at the late tetrad stage, some microspores were observed in tetrads with a deviating type of development. They had a much denser cytoplasm (A, to the left) in comparison to normal ones (A, to the right) and a dense nucleus. Many double-membrane organelles with dark-contrasted inclusions are seen in both normal and deviating microspores (A). At higher magnification (×50 000), the cytoplasm of such “dark” microspores appears to be packed with dark-contrasted thread-like inclusions in association with small roundish particles. These look like strings of beads, or just strings – a form of micelles (B). At this magnification, the dark-contrasted inclusions that are associated with double-membrane organelles (probably smaller plastids), are deposited not directly inside these organelles, but are separated either by double-membranes and located inside deep invaginations of these organelles, or are within the luminae of two membranes (B). Dark inclusions are often in close association with lipid globules (B), and strings of the cytoplasm often cross the border of lipid globules, as if they have pierced them (B, D). Crystal-like formations are observed inside double-membrane organelles, where they form asterisk-shaped or needle-shaped structures (C, D). At the same late tetrad stage, microspores with atypical massive exines appear; their occurrence is approximately the same as that of atypical microspores at the previous tetrad stage: several are found in each ultrathin section. They have double-membrane organelles with partly degraded stroma, which are probably lysosomes: several steps of clarification of the contents of these organelles as well as single-membrane organelles that are typical for lysosome formation are observed (E). Dark-contrasted strings in the cytoplasm of such microspores appear less discernible (E). To try to reveal the chemical composition of the strings (at least approximately) in the cytoplasm and the composition of dark inclusions in double-membrane organelles, we have undertaken enzymatic histochemical tests with pronase E and lipase. Treatment of sections with a 1% solution of pronase E at 20°C for 22 h has led to the disappearance of strings in deviating microspores with dark cytoplasm (lower microspore in A). Treatment with a 1% solution of pronase E at 37°C for 4 h has not revealed “strings” in the cytoplasm, but they are preserved inside double-membrane organelles (B). The dark contrasted inclusions in double-membrane organelles have lost some of their contrast, but still persist (B). Treatment of sections with 1% solution of lipase at 37°C for 4 h have shown similar results: strings are preserved within double-membrane organelles but have disappeared from the cytoplasm (C).
Figure 6. Deviating microspores at the late tetrad stage in Chamaedorea microspadix. A. Some microspores, observed in tetrads, deviate from the normal development and have a much denser cytoplasm (on the left: am – atypical microspore) in comparison to normal ones (on the right: tm – typical microspore). They also have a much denser atypical nucleus (an). Many double-membrane organelles with dark-contrasted inclusions are seen in both normal and deviating microspores (white and black arrows). B. This higher magnification shows that the cytoplasm of such microspores is packed with dark-contrasted thread-like inclusions in association with small roundish particles, so-named “strings of beads” (arrows). In some places, these strings cross the restricting membranes of organelles (double arrowhead). A dark-contrasted substance associated with double-membrane organelles (plastids or dedifferentiated mitochondria) is separated from the body of these organelles by a double membrane (arrowheads) and is located inside invaginations of double membrane. C, D. Needle-shaped or star-shaped formations are observed in the stroma of double-membrane organelles (arrows). E. At the same stage, microspores with untypical massive exine are seen. Double-membrane organelles with associated dark-contrasted inclusions are converted into lysosomes (ls). They first lose the inner membrane and then gradually lose their dark inclusions. Strings in the cytoplasm are less discernible (arrowheads). Legend to all figures: aex – atypical exine, c – callose, dm – double-membrane organelles, ex – exine, gs – Golgi stack, lg – lipid globule, mc – microspore cytoplasm, n – nucleus, v – vacuole. Scale bars – 0.5 μm.
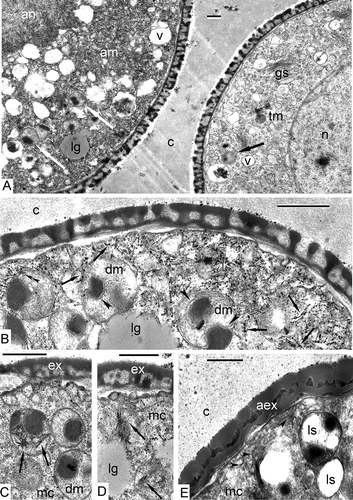
Figure 7. Cytochemical reactions. A, B. Treatment of the section with 1% solution of pronase E: A. Treatment at 20 °C for 22h. Two microspores of a tetrad, one typical (upper one: tm) and one atypical, with dark cytoplasm (lower one: am) have no “strings” in the cytoplasm; B. Treatment at 37 °C for 4h. Deviating microspore (am). No “strings” are recognisable in the cytoplasm, but they are preserved inside double-membrane organelles (arrows). C. Treatment of the section with 1% solution of lipase at 37 °C for 4h. “Strings” are well preserved inside the double-membrane organelles (arrows), but disappeared from the cytoplasm. Legend to all figures: c – callose, dm – double membrane organelle, ex – exine, lg – lipid globule, ls – lysosome, p – plastid, v – vacuole. Scale bars – 0.5 μm.
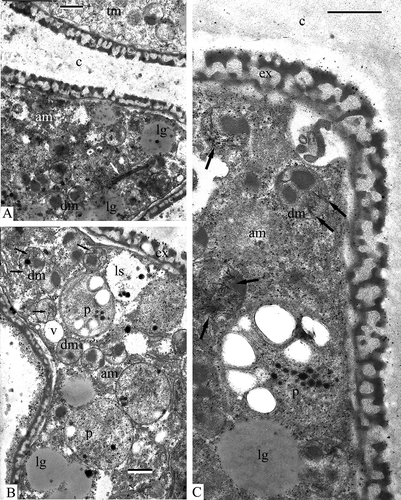
Normal late tetrad microspores from the disintegrating tetrad (A) have a quite well-formed ectexine, a very thin discontinuous endexine, which is still in the process of formation, cytoplasm with many typical plastids and double-membrane organelles with dark-contrasted inclusions of different form, many active Golgi stacks and their vesicles, vacuoles, multivesicular bodies, ER cisternae. The callose “jacket” of the tetrads is disintegrating (A).
Figure 8. The end of the tetrad period and the beginning of the post-tetrad period in Chamaedorea microspadix. A. Late tetrad microspore from the tetrad with disintegrating callose (dc) has a quite well-formed exine (ex) with very thin and a poorly pronounced endexine, cytoplasm with plastids (p), double-membrane organelles (dm) with dark-contrasted inclusions, many active Golgi stacks (gs) and their vesicles, vacuoles (v), multivesicular bodies (mvb), and ER cisternae (er). B. Free young microspore, submedial section through the sulcus. The first endexine lamella is well seen by its “white line” (arrows), the next endexine lamella is in the process of formation (arrowheads). C. The details of endexine lamella formation. A region of strings, the latter arranged parallelly, is recognisable in the periplasmic space (ps, arrows) between the plasma membrane (pl) and the first endexine lamella (white arrowhead). Outgrowths on the surface of the tectum are long (black arrowheads). Legend to all figures: ect – ectextine, mc – microspore cytoplasm, pl – plasma membrane. Scale bars – 0.5 μm.
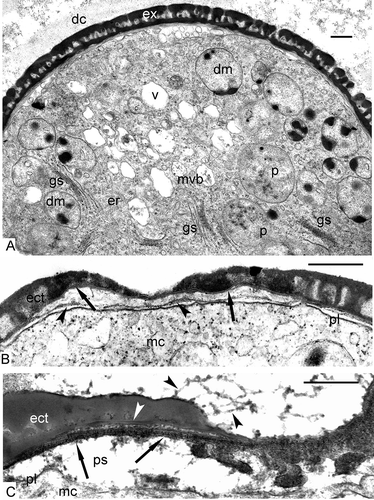
Post-tetrad period: young free microspores
After their release from tetrads, microspores increase in size and all the elements of the exine grow in thickness because of additional accumulation of SP of tapetal origin. In a submedial section through the sulcus, the first (primordial) endexine lamella is well seen by virtue of its “white line” (B), and the next endexine lamella is in the process of formation (B). As a result of this process, some osmiophilic substance accumulates in the periplasmic space between the plasma membrane and the first endexine lamella (C), and a region of strings, disposed in parallel, is observed (C). Another interesting feature is the presence of outgrowths on the surface of the tectum at this stage, some of them are long (C; A, B).
Figure 9. Post-tetrad period in Chamaedorea microspadix. A, B. Free mature microspores. Outgrowths on the surface of the tectum are recognisable (black arrowheads). The endexine is very thin (arrow in A). C. Two-celled pollen grain after completing microspore mitosis (gc – generative cell, vc – vegetative cell). The intine layer is well-seen in the area as distal aperture (s – sulcus). D. A fragment of a pollen grain with intine layer (i) in the aperture, very thin and torn membrane of the aperture (arrowhead) and plenty of nutritive substances as starch in amyloplasts (ap), fat in lipid globules (lg) and other substances in vacuoles (v). Legend to all figures: ect – ectexine, ex – exine, lg – lipid globules, mc – microspore cytoplasm, pl – plasma membrane, vc – vegetative cell. Scale bars – 0.5 μm (A, B), 5.0 μm (C, D).
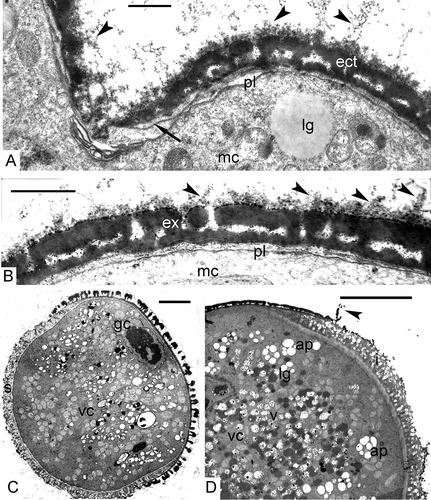
Two-celled pollen grains
After microspore mitosis and the appearance of the generative cell, a layer of intine appears (C, D). The intine consists of two layers: the first is the so-called channelled intine, which is localised in the aperture region only (sulcus in D), and the second, inner layer is fine-fibrillar and covers the whole pollen grain (A). The membrane of the aperture, consisting of the foot layer of the ectexine and a very thin endexine, is usually torn in the course of material preparation (D). The cytoplasm of the vegetative cell is packed with lipid globules, plastids with starch grains (amyloplasts), and vacuoles with nutritive contents (D).
Figure 10. Pollen grains of Chamaedorea microspadix. A. Interaperture region. Weak-contrasted, fine-fibrillar second layer of intine (i 2) continues around the whole pollen grain. A very thin supratectum layer (arrowhead) covers the tectum. B. Unlike the second intine layer, the channelled intine-1 (i 1) stretches only over the aperture region. The channels of intine-1 (arrows) are cylindrical structures in radial sections. C. Half-an-aperture view. Both intine-1 (i 1) and intine-2 (i 2) are discernible, and also the ectexine (ect) and a dark-contrasted thin endexine (arrow). D. When cross-sectioned, the channels of intine-1 (i 1) appear as circular units with fibrillar contents (arrowheads). E‐G. SEM images of mature pollen grains. E. Proximal view. F. Distal view with sulcus. G. The sculpture of pollen grain can be regarded as perforate. Legend to all figures: co – columella, ex – exine, fl – foot layer, mc – microspore cytoplasm, t – tectum. Scale bars – 1.0 μm (A–D, G), 5.0 μm (E, F).
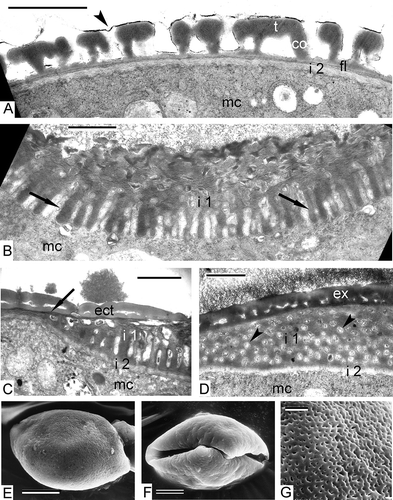
In interaperture regions, the weak-contrasted fine-fibrillar second layer of the intine is well seen (A). It continues around the whole pollen grain. A very thin supratectum layer (A) covers the tectum. Unlike the second intine layer, the channelled intine-1 stretches only over the apertural region. The channels of the intine-1 are cylindrical structures in radial sections (B, C), and when cross-sectioned, appear as circular units with fibrillar contents (D). SEM images of mature pollen grains reveal that the sculpture of pollen grain can be regarded as perforate (E, proximal view, 10F, distal view with sulcus, 10G).
Discussion
The data obtained in this study provide support to our previous hypothesis (Gabarayeva & Hemsley, Citation2006; Hemsley & Gabarayeva, Citation2007). In accordance with the latter, growth of the exine progresses by successive transitory mesophases of a constrained self-assembling micellar system. In such a case, a system passes through a sequence of self-organising pseudophases (=mesophases) such as spherical micelles, cylindrical micelles, layers of hexagonally packed cylindrical micelles, multilayered structures of double layers, separated with gaps (neat micelles), bicontinuous labyrinths, etc. (see & in Hemsley & Gabarayeva, Citation2007). The non-linear nature of self-assembly (when small changes in parameters may have no effect or a great effect; Hemsley et al., Citation1998) causes the abrupt transitions (“switches”) between micellar mesophases. The latter receive their morphological expression as different sporoderm layers.
The underlying cause of the glycocalyx pre-structures
As shown above, the first elements appearing on the plasma membrane of the microspores at the early tetrad stage are dark-contrasted globules (A). Similar globules have been observed at this initial tetrad stage in many species (Dickinson, Citation1976a , Citation b ; Hesse, Citation1985; El-Ghazaly & Jensen, Citation1987; Skvarla & Rowley, Citation1987; Gabarayeva, Citation1991a ; Rowley et al., Citation1992; Takahashi, Citation1993; Gabarayeva & Rowley, Citation1994; Gabarayeva, Citation1995; Gabarayeva & El-Ghazaly, Citation1997; Gabarayeva, Citation2000; Gabarayeva & Grigorjeva, Citation2002, Citation2003; Gabarayeva et al., Citation2009a ). These could be glycoproteins, synthesised and delivered by Golgi stacks on the plasma membrane surface. Taking into consideration that glycoproteins are diphilic, surface active substances (surfactants), the dark globules on the microspore plasma membrane are most probably the first mesophase, self-assembling in the surfactant solution: spherical micelles [for the schemes of self-assembling micellar mesophases, see & from Gabarayeva and Hemsley (Citation2006) and from Hemsley and Gabarayeva (Citation2007)]. Another possibility is that these globules are lipophilic nutritive substances of tapetal origin which also form spherical micelles in a hydrophilic medium.
Ribbon-like striped structures, parallel to the plasma membrane (C), are most probably a kind of micelles, called “long, segmented worms” (Lodge et al., Citation2005). These kinds of micelles occur in experiments with mixed polymers, where they form block copolymers (diblock, triblock, multiblock, etc.). In case of triblock copolymers, strings of spherical micellar “beads” (or just “strings”) or long, segmented “worms” appear. The latter emerge when all three blocks are extremely strongly segregated from one another. This causes very high interfacial tension which encourages flat interfaces (seen as segments of “worms”). Interestingly, in aqueous diblocks, both worm-like micelles and vesicles have been well-documented (Lodge et al., Citation2005). Thus, in the early tetrad period of pollen wall development, when the callose envelope is adjacent to glycoproteins of the forming glycocalyx, and when some lipoid substance is evidently present at the same site, a situation may arise close to that described in experiments of Lodge et al. (Citation2005). The callose (β-1,3-glucane), existing in a water-based medium of thecal fluid, swells and represents a kind of homopolymer colloid. Being a swelling colloid [a gel, see Ball (Citation1994)], the callose envelope may behave actively in respect to the adjacent macromolecules of glycoproteins of the glycocalyx, promoting a structure-forming process at the interface (Gabarayeva et al., Citation2009a ), especially because the third component (some lipoid substance, maybe one of sporopollenin precursors, fatty acids) interferes with the process.
Another possibility may exist when incompatible polymers meet. Hamm et al. (Citation2004) have prepared a trilayer system of three different polymers: amorphous homopolymer layer, liquid crystal (nematic) homopolymer layer, and an amorphous-nematic copolymer at the interface between them. As a result, a lamellar micellar structure appears first in the interfacial copolymer, which, proving unstable, changes towards a “worm-like” layer, which then fuses with both homopolymer blocks providing an interface, resembling a “columellate-tectate exine patterning”. This simulation by Hamm et al. (Citation2004) provides an idea regarding what is observed in the periplasmic space at the combination of callose (amorphous homopolymer), glycocalyx (nematic or smectic liquid crystals) and microemulsions of sporopollenin precursors (Gabarayeva et al., Citation2009a ), resulting in an emergent primexine pattern as interfacial micellar mesophases of three components.
After a short developmental step when worm-like micelles (which persist during development – see below) coexist with dark-contrasted globular micelles, a new step demonstrates a new kind of micellar system in the periplasmic space – a layer of smaller spherical units-micelles, some of them in radial columns (D, E). This process – the transition from a spherical micelle mesophase to a cylindrical mesophase – causes the arrangement of spherical units into columns (F) and then to rod-like units (G). The latter are first very thin (10 nm in diameter) and correspond to substructural units of a tuft in the terminology of Rowley and Flynn (Citation1968) and Rowley (Citation1990), which form a pre-pattern for the future primexine.
Suggested controls of primexine pattern formation
The middle tetrad stage is a crucial point in exine pattern determination. The initial rod-like units appear more prominent. They evidently consist of several cylindrical micelles, which correspond to tufts, arranged into funnel-like complexes (); the distal ends grow laterally and adjoin with each other forming a reticulate tectum. These “funnels” are surprisingly similar to the gyroid phase (G) demonstrating structures formed by a diblock copolymer. Such structures in Chamaedorea periplasmic space are disposed parallel to each other (perpendicularly to the plasma membrane), whereas the scheme (G) shows a different, free distribution of elements throughout the volume of the solution. A possible reason may be that additional constraints are imposed on the colloidal system in the narrow periplasmic space. If some common flexible self-assembling micellar machinery is the basis for both experimental and natural processes during sporoderm ontogeny (which is our belief), then the gyroid mesophase could be the framework for the future columellate-tectate pattern in Chamaedorea and other species with complex, branching columellae in their exines. This would be the reason why such patterns are so widespread in nature. Block polymers are structure-directing materials, which illustrate the remarkable flexibility that self-assembly affords. The design variables include the number and chemical identity of the ingredients and their molecular weight, composition, and architecture. The main mesophases in this scheme () are the same as in a sequence of “simple” typical surfactant solutions (see in Gabarayeva et al., Citation2009a ), with the exception of the double gyroid phase (G) with its branching structure. The latter, however, can also be regarded as a kind of bicontinuous double-labyrinth structure, known for simple water-lipid surfactant systems.
Figure 11. Schematic illustration of the nanostructures formed by an AB diblock copolymer in the bulk. The various phases are: S (spheres), C (hexagonally-packed cylinders), G (double gyroid), and L (lamellae). The white and grey domains represent the A and B blocks, respectively, as the fraction of A in the copolymer increases from left to right (figure taken from Lodge et al., Citation2005, with permission of the Royal Society of Chemistry, London).

An important developmental step is the contrast reversal: rod-micelles initially have a dark core and light outlines (E), but then they have a light core and dark outlines (F) – a phenomenon observed in many species (see a review in Hemsley and Gabarayeva, Citation2007). This seems to correspond to inversion of normal micelles to reverse (turned inside-out) micelles in result of a shift in hydrophilic/hydrophobic balance in the periplasmic space. Reverse of contrast is especially well seen (H), where several ring-like units (flattened spherical micelles) are components of a rod (cylindrical micelle). Inversed micelles with their water-loving interiors are open for transfer of nutritive substances from the thecal fluid toward the developing microspore cytoplasm.
The formation of the initial foot layer demands special consideration. It appears initially in fragments as strings of tiny (about 10 nm in diameter) spherical micelles (B, D, E). Collinson et al. (Citation1993) considered (1) spherical micelles forming “strings of beads” as the gradual transition from spherical to filamentous (cylindrical) micelles, and (2) perforated laminae as a transition to continuous laminae. The authors noted that the gradual transition illustrates the way in which different colloidal systems occur from minor changes in physico-chemical parameters. We have observed the first type of transition in earlier Chamaedorea exine development when spherical micelles have been self-arranged into radial columns at the early tetrad stage (D, E); the second type was observed in foot layer initiation, where a double-stepped transition takes place: from spherical micelles to strings (or worm-like micelles), and from these filamentous forms to laminate ones. Later in development, the initial framework of the foot layer accumulates SP and some fragments of the foot layer (B, D) still bear the striped pattern of segmented worm-like micelles. In many other species, juvenile intermediate forms of micelle mesophases persist in the mature exine. For example, the columellae contain internal foramina and look lace-like in transmission electron microscopy (TEM) in representatives of the helianthoid exine of Compositae (Blackmore et al., Citation2010). At the late tetrad stage, juvenile lamellae of the endexine seem to appear on the base of perforated laminae, because the images of the lamellae are discontinuous, and the initial SP accumulation is uneven (B). At the next ontogenetic moment, in young free microspores, typical endexine lamellae with their central “white lines” appear (B). These, as was suggested earlier (Gabarayeva & Hemsley, Citation2006; Hemsley & Gabarayeva, Citation2007; Gabarayeva et al., Citation2009b ), are the next micellar mesophase (laminate or “neat” micelles), formed of parallel double layers, separated with gaps, seen in TEM as white lines (see also the scheme in L). The formation of a few (two or three) endexine lamellae continues after the release of microspores from tetrads and strings are observed again in the region of the lamella formation (C).
This switch in exine microarchitecture from radially oriented structures (columellae) to tangentially oriented ones (foot layer, endexine lamellae) may also be connected with the appearance of a diffusion barrier for two different types of molecules at the interface of two miscible liquids. It has been shown that upon liquid-to-liquid contact of two aqueous solutions (a polysaccharide and an amphiphile peptide, bearing opposite electrostatic charge), the formation of a diffuse barrier prevents their chaotic mixing, resulting in structures with highly ordered architecture in which nanofibers first align and then re-orient by nearly 90° as the membrane grows (Capito et al., Citation2008). These authors believe that growth of the membrane is driven by a dynamic synergy between osmotic pressure of ions and self-assembly and that hierarchical order assists their greater hydrolytic stability.
We suggest that the hierarchical order of developing exines contributes to their hydrolytic stability and robust mechanical properties, preventing possible “self-disassembling” of the successive micelle mesophases during exine development, when the exine is not yet stabilised by polymerisation of sporopollenin.
One important feature of exine development is its lability and capacity to vary. No strict “rules” exist for the sequence of developmental events. For example, close-to-radially oriented columellae in developing tetrad microspores of Hedycarya arborea J. R. Forst. & G. Forst., or “radial processes”, as named by Sampson (Citation1977, ), which have distinct “white lines” in the centre – a feature, rather typical for tangentially oriented endexine lamellae of many species. The phenomenon was so atypical that Guédès (Citation1982) estimated these radial processes of Hedycarya as “lifted endexine”. Comparable structures with “white lines” were shown in Tambourissa (Rowley & Flynn, Citation1991). A similar phenomenon (“lifted” membranes, oriented perpendicularly to the plasma membrane) was observed as initial structures in columellae development in Lilium (Dickinson & Heslop-Harrison, Citation1968). We believe that radial “white-lined” structures appear in result of inversion of cylindrical micelles (and corresponding stain reversal in material prepared for TEM). Another possibility is that they appear on the base of cable-like “empty” filaments – an intermediate form of cylindrical and laminate (neat) micelles, the latter in this case re-oriented about 90° relatively to its usual tangential position.
It is striking how different pollen and spore wall structures in the scheme of Guédès [1982, – (1) granules, (2) strings of granules, (3) columns of granules, (4) columellae, (5) “empty” rod-like columellae, (6) “empty” radially oriented columellae with dilatations (looking as if they consist of granules), (7) lamellae with centred white lines] fits all the main mesophases of micelles and their transitive forms: (1) spherical micelles, (2) strings of spherical micelles, (3) columns of spherical micelles, (4) normal cylindrical micelles, (5) reverse (inversed) cylindrical micelles, (6) transitional form between reverse spherical and cylindrical micelles/strings, and (7) lamellate (neat) micelles after accumulation of SP on them. Another important point is that the same main micelle mesophases (with additional specific transitory ones) have been observed over the sporoderm development in dicotyledons under our previous studies (Gabarayeva et al., Citation2009a , Citation b and references therein) and in the monocotyledon Chamaedorea microspadix.
Microspores with atypical development
Atypical microspores with dark-contrasted cytoplasm attract special attention (). These are packed with strings of some osmiophilic substance. Whatever this substance was, its synthesis was definitely excessive, resulting in some deviation from the normal microspore development. This supposition was supported by the presence of several microspores in the anther loculus with atypical massive exine and remnants of strings in the cytoplasm (E). Such an exine pattern is characteristic of representatives of archaic Magnoliaceae species (Gabarayeva, Citation1986, Citation1987, Citation1991b ) rather than of Chamaedorea. In normally developed Chamaedorea microspores, the exine pattern is more delicate (A).
At the late tetrad stage, the dominating process in the periplasmic space is the accumulation of SP on the primexine framework. Therefore, it seems natural to suggest that some osmiophilic SP precursors were synthesised by some microspore organelles [presumably in Chamaedorea, by those double-membrane organelles (abnormal mitochondria or generation of small plastids?), which also contains strings and star-shaped crystals]. These precursors may be unsaturated fatty acids (Dominguez et al., Citation1999; Ahlers et al., Citation2000) or aromatic monomers, particularly p-coumaric and ferulic acids (De Leeuw et al., Citation2006). Regretfully, there are no specific cytochemical tests to reveal fatty acids or aromatic monomers, so we attempted to discover which class the substance of strings belongs to. The results received after treatment of sections with enzymes pronase E (A, B) and lipase (C) have revealed that the two enzymes have been active. The disappearance of strings from the microspore cytoplasm (A–C) suggests that strings contain a complex of protein and lipid, for instance, some lipoprotein. However, strings within double-membrane organelles and dark inclusions of these organelles persist, just losing in part its contrast (C). The question whether the substance of dark-contrasted inclusion is the same as that of strings remains ambiguous, but location of some strings inside double-membrane organelles provides some evidence for a positive answer. It is also probable that proteins in strings are in association with lipids and represent, for instance, some non-specific lipid transfer protein (nsLTP), which, as was recently observed by Levine (Citation2007), transfers lipids and participates in to different pathways of lipid traffic. The fact that our tests have shown resistance of strings within the domain of double-membrane organelles, but not through the cytoplasm, may have an explanation in that nsLTP have slightly other properties at the location of their synthesis, especially since in some cases nsLTPs are known to be resistant to heat treatment and proteolytic digestion enzymes (Asero et al., Citation2000). Kader (Citation1996) was able to show that nsLTPs are precursors for the secretory pathway and are secreted outside the cell wall as polypeptides. Immunocytochemical localisation of nsLTPs in pollen grains of several species from different taxa indicated that nsLTPs were involved in the traffic of defence proteins (Suárez-Cervera et al., Citation2008). LTPs bind lipids in a hydrophobic pocket and carry them across the aqueous environment of the cytoplasm (Levine, Citation2007).
Wherever the substance of strings was synthesised, one thing is definite: there is hypersynthesis of some protein-lipid complex in deviating microspores, expressed in a massive appearance of string-micelles through the cytoplasm and atypically thick exine.
Exine maturation and intine appearance in the post-tetrad period
During exine maturation in the free microspore period, additional accumulation of SP from the tapetum proceeds in the primexine as usual. An interesting feature of free microspores is the numerous outgrowths on the surface of the tectum, some of them being rather long (C, A, B). These structures could be those that were described as “wicks” by El-Ghazaly et al. (Citation2000) and Rowley and Morbelli (Citation2009) – the continuation of the radial units of the glycocalyx (tufts = cylindrical micelles). The authors consider them to be continuous connections linking microspores with the tapetum and functionally destined for facilitation of delivery of the nutritive substances from the tapetum to the microspores. In the sporangium of Selaginella, where wicks are disposed very regularly and many of them are parallel to each other, they look impressive if the plane of a section coincides with the plane of wicks disposition (Rowley & Morbelli, Citation2009). Such impressive formations were not observed in anthers of seed plants, probably due to the less ordered disposition of wicks.
The first layer of two-layered intine of Chamaedorea microspadix is channelled and located only under the aperture. The images (B–D) are very similar to those of the channelled zone of species with a thin, rudimentary exine and thick intine [as e.g. in Heliconia and Canna (Stone et al., Citation1979; Kress & Stone, Citation1982; Skvarla & Rowley, Citation1986), Strelizia (Hesse & Waha, Citation1983), and Zingiber (Theilade & Theilade, Citation1996)]. Interestingly, the images of the channelled intine of Chamaedorea are similar to the images of cylindrical alveolae of ectexine in the cycads Stangeria and Encephalartos (Gabarayeva & Grigorjeva, Citation2002, Citation2004). The latter probably form on the base of cylindrical micelles (Gabarayeva & Hemsley, Citation2006). An informative study on the development of the central channelled intine layer in Aristea major Andrews (Iridaceae) with cytochemical data in dynamics was undertaken by Suárez-Cervera et al. (Citation2000). The authors demonstrated that the appearance of plasma membrane protrusions and intervention of ER and Golgi vesicles in the development precedes the initiation of the channelled layer. However, later the membranes are not observed along the walls of the channels. Instead, fragments of lamellae are seen inside the channels. Examination of the , and of Suárez-Cervera et al. (Citation2000) enabled us to discern tripartite structure of some lamellae with white line in the centre. Lamellar fragments and vesicles are seen also in the vicinity of the plasma membrane, inside the zone of initiation of the channelled layer (Suárez-Cervera et al., Citation2000, ). The cytochemical data demonstrate a positive reaction for glycoproteins; Suárez-Cervera et al. (Citation2000) emphasise a polygonal (actually hexagonal) distribution of channels, especially well-seen in cross sections. These observations are similar to those described by Heslop-Harrison (Citation1987).
Our suggestion is that channelled intines in different species (including Chamaedorea microspadix and Aristea major) develop on the base of glycoprotein cylindrical micelles. It was shown immuno-cytochemically for other, not channelled types of intine, that glycoprotein/protein ingredients are common components of the intine (Suárez-Cervera et al., Citation2005). Other transitional micelle structures (fragments of tripartite lamellae, strings, vesicles) accompany cylindrical units of the main (for this stage) so-called “middle” mesophase. Cylindrical micelles are capable of conducting water and different substances, soluble and insoluble in water. In the moist environment, they easily undergo gelification and disintegration, as it takes place during pollen tube growth.
A summarising semi-scheme showing the main developmental steps in Chamaedorea microspadix sporoderm development is provided (). The scheme is based on original micrographs and on our interpretation of them through the realm of micelles.
Figure 12. Semi-schematic illustration showing the main developmental steps in Chamaedorea microspadix (strongly clarified original micrographs, with several structural elements, emphasised by Indian ink, left column) and schematic images of micelle mesophases, which, according to our belief, correspond to the observed structural elements (right column). A. Early tetrad stage. A mixture of spherical units and long striped ribbons in the glycocalyx (see C) corresponds to mixture of spherical micelles and so-called segmented worms. B. Early tetrad stage. Many spherical units in the glycocalyx (see D) correspond to spherical micelles. C. Early tetrad stage in progress. Spherical units, arranged first into radially oriented columns and then to rod-like structures (see F), correspond first to columns of spherical micelles, then to cylindrical micelles. D. Middle tetrad stage. Funnel-like procolumella (see E), consisting of several bent rod-units with a dark core and light halo (shown by arrows) corresponds to a cluster of bent normal cylindrical micelles. E. A similar procolumella on the point of contrast change (see F) corresponds to a cluster of bent normal cylindrical micelles on the point of switch to inverse micelles. F. Rod-like unit of a procolumella with a light core and dark halo after contrast reversal (see H) corresponds to bent reverse cylindrical micelle. G. Striped ribbon-like structures and chains of tiny vesicales (as initial foot layer) in the glycocalyx (a fragment of D) correspond to two micelle forms, i.e., “segmented worms” and strings. H. Late tetrad stage. The foot layer after progressive sporopollenin accumulation which bears traces of segmentation (see D) corresponds to initial “segmented worms” and strings, arranged to perforated layer. I. Late tetrad stage in progress. The first white-lined endexine lamella and fragments of new-forming lamellae (see B) correspond to laminate (“neat”) micelles and strings or/and perforated layers. J. The first intine layer with cylindrical units (see ) corresponds to layer of hexagonally packed cylindrical micelles (“middle” micelles).
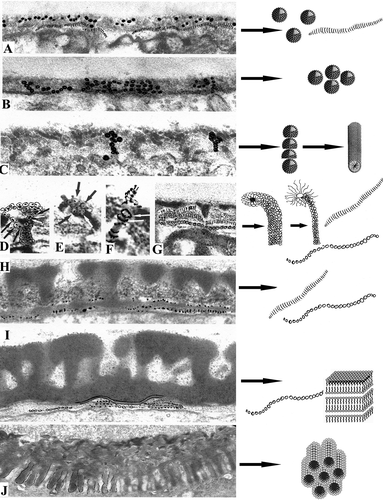
Conclusions
All of the pre-pattern structures of the Chamaedorea microspadix pollen wall at an early tetrad stage (i.e., dark-contrasted globules, ribbon-like striped structures, strings of beads, spherical units, radially oriented columns of spherical units, rod-like units) can be easily read as different types of micelles (spherical, segmented “worms”, cylindrical) and their intermediate forms (strings, perforated filaments that are columns of spherical micelles).
The initial rod-like units of the early tetrad stage switch to funnel-like procolumellae, arranged as complexes of bent rod-like units at the middle tetrad stage. These complexes consist of transformed cylindrical micelles, which separate from each other distally, forming the tectum. These “funnels” could correspond to a double gyroid phase, typical for a diblock copolymer system, adapted to constraints of the narrow periplasmic space. Block polymers illustrate the remarkable flexibility that a self-assembly toolbox affords. The double gyroid phase, with its branching structure, can be also regarded as a kind of bicontinuous double-labyrinth structure, known for simple water (lipid) surfactant systems. It is possible that it is an emergent gyroid phase of the colloidal system (glycoproteins, callose, later the sporopollenin) in the periplasmic space that causes the widespread columellate-tectate pattern of developing exines.
On the other hand, the callose envelope may behave actively in respect to the adjacent macromolecules of glycoprotein (glycocalyx), promoting a structure-forming process at the incompatible polymer interface.
The foot layer and a few endexine lamellae with central white lines appear as laminate (“neat”) micelles, with a typical gap between the laminae. They emerge via an intermediate form that may represent strings of spherical micelles and/or perforated laminae.
These strings are probably a preferential intermediate form of micelle formation in the course of exine development in Chamaedorea. They emerge at every ontogenetic step, participating in the appearance of every exine layer.
The switch in exine microarchitecture from radially oriented structures (columellae) to tangentially oriented ones (foot layer, endexine lamellae) may also be connected with the appearance of a diffusion barrier for two different types of molecules at the interface of two miscible liquids. In this case, growth of the membranes is driven by a dynamic synergy between osmotic pressure of ions and self-assembly.
Numerous double-membrane organelles with dark-contrasted inclusions (a generation of atypical mitochondria or small plastids) are characteristic of Chamaedorea microspadix microspores. Their role remains ambiguous. They are probably involved in the synthesis of strings (a kind of micelles), which overcrowd the cytoplasm of deviating microspores, bringing to their deviation from the normal pathway of development. Cytochemical data give some evidence that hyper-synthesis of some lipoprotein substance (or non-specific lipid transfer protein) leads to the appearance of this transitional form of micelles and atypically massive exine.
The first (channelled) layer of the two-layered intine beneath the aperture seems to appear on the base of cylindrical micelles.
The sequence of sporoderm layer formation can be understood as a sequence of self-assembling mesophases and their intermediate forms in the colloidal system of the microspore periplasmic space, which are unfolding during ontogeny. The non-linear nature of self-assembly brings about the sudden, abrupt character of transitions. An especially abrupt shift is at endexine initiation, when radially-arranged elements of ectexinic domain switch to tangentially-arranged endexinic ones. Taking into consideration that genome-determined composition of the colloidal solution (of the supporting medium, concentrations of dispersed substances and ions) changes gradually, but self-assembling phase transitions are jump-like, the unity of continuity and discreteness once again steps forward as a fundamental and widespread phenomenon in nature.
Supplementary Data
Download Zip (38.3 MB)Acknowledgements
This work was supported by a Russian Foundation for basic research grant (No. 08-04-00498). We are thankful to Bruce Sampson for language corrections. We also thank our engineer Peter Tzinman for assistance with the Hitachi H-600 transmission electron microscope, and Nikolay Arnautov for permission to use material from the Komarov Institute greenhouses.
References
- Ahlers , F. , Bubert , H. , Steuernagel , S. and Wiermann , R. 2000 . The nature of oxygen in sporopollenin from the pollen of Typha angustifolia L . Zeitschrift für Naturforschung , 55 : 129 – 136 .
- Asero , R. , Mistrello , G. , Roncarolo , D. , de Vries , S. C. , Gautier , M. F. , Ciurana , C. L. F. , Verbeek , E. , Mohammadi , T. , Knul-Brettlova , V. , Akkerdaas , J. H. , Bulder , I. , Aalberse , R. C. and Van Ree , R. 2000 . Lipid transfer protein: A pan-allergen in plant derived foods that is highly resistant to pepsin digestion . International Archives of Allergy and Immunology , 122 : 20 – 32 .
- Ball , P. 1994 . Designing the molecular world , Princeton : Princeton University Press .
- Belitser , N. V. , Zaalishvili , G. V. and Sytnianskaja , N. P. 1982 . Ca2+-binding sites and Ca2+-ATPase activity in barley root tip cells . Protoplasma , 111 : 63 – 78 .
- Blackmore , S. , Wortley , A. H. , Skvarla , J. J. , Gabarayeva , N. I. and Rowley , J. R. 2010 . Developmental origins of structural diversity in pollen walls of Compositae . Plant Systematics and Evolution , 284 : 17 – 32 .
- Blackmore , S. , Wortley , A. H. , Skvarla , J. J. and Rowley , J. R. 2007 . Pollen wall development in flowering plants . New Phytologist , 174 : 483 – 498 .
- Capito , R. M. , Azevedo , H. S. , Velichko , Y. S. , Mata , A. and Stupp , S. I. 2008 . Self-assembly of large and small molecules into hierarchically ordered sacs and membranes . Science , 319 : 1812 – 1816 .
- Collinson , M. E. , Hemsley , A. R. and Taylor , W. A. 1993 . Sporopollenin exhibiting colloidal organization in spore walls . Grana , : 31 – 39 .
- Dashek , W. V. , Thomas , H. R. and Rosen , W. G. 1971 . Secretory canal cells . American Journal of Botany , 58 : 909 – 920 .
- De Leeuw , J. W. , Versteegh , G. J. M. and Van Bergen , P. F. 2006 . Biomacromolecules of algae and plants and their fossil analogues . Plant Ecology , 182 : 209 – 233 .
- Dickinson , H. G. 1976a . The deposition of acetolysis-resistant polymers during the formation of pollen . Pollen et Spores , 18 : 321 – 334 .
- Dickinson , H. G. 1976b . “ Common factors in exine deposition ” . In The evolutionary significance of the exine , Edited by: Ferguson , I. K. and Muller , J. 67 – 89 . London : Academic Press .
- Dickinson , H. G. and Heslop-Harrison , J. 1968 . A common mode of deposition of the sporopollenin of sexine and nexine . Nature , 220 : 926 – 927 . (London)
- Dickinson , H. G. and Sheldon , J. M. 1986 . “ The generation of patterning at the plasma membrane of the young microspore of Lilium ” . In Pollen and spores: Form and function , Edited by: Blackmore , S. and Ferguson , I. K. 1 – 18 . London : Academic Press .
- Dominguez , E. , Mercado , J. A. , Quesada , M. A. and Heredia , A. 1999 . Pollen sporopollenin: Degradation and structural elucidation . Sexual Plant Reproduction , 12 : 171 – 178 .
- El-Ghazaly , G. and Jensen , W. A. 1987 . Development of wheat (Triticum aestivum) pollen. II. Histochemical differentiation of wall and Ubisch bodies during development . American Journal of Botany , 74 : 1396 – 1418 .
- El-Ghazaly , G. , Moate , R. , Huysmans , S. , Skvarla , J. and Rowley , J. R. 2000 . “ Selected stages in pollen wall development in Echinodorus, Magnolia, Betula, Rodeletia, Borago and Matricaria ” . In Pollen and spores: Morphology and biology , Edited by: Harley , M. M. , Morton , C. M. and Blackmore , S. 17 – 29 . Kent : Whitstable Printers Ltd, Whitstable .
- Ferguson , I. K. and Harley , M. M. 1993 . The significance of new and recent work on pollen morphology in the Palmae . Kew Bulletin , 48 : 205 – 243 .
- Furness , C. A. and Rudall , P. J. 1999 . Microsporogenesis in monocotyledons . Annales of Botany , 84 : 475 – 499 .
- Gabarayeva , N. I. 1986 . The development of the exine in Michelia fuscata (Magnoliaceae) in connection with the changes in cytoplasmic organelles of microspores and tapetum . Botanicheski Zhurnal (Leningrad) , 71 : 311 – 322 . In Russian, with English summary
- Gabarayeva , N. I. 1987 . Ultrastructure and sporoderm development in Manglietia tenuipes (Magnoliaceae) in the tetrad period: The establishment of primexine in connection with functioning of cytoplasmic organelles . Botanicheski Zhurnal (Leningrad) , 72 : 281 – 290 . In Russian, with English summary
- Gabarayeva , N. I. 1990 . Hypothetical ways of exine structure determination . Botanicheski Zhurnal (Leningrad) , 75 : 1353 – 1362 . In Russian, with English summary
- Gabarayeva , N. I. 1991a . “ Patterns of development in primitive angiosperm pollen ” . In Pollen and spores , Edited by: Blackmore , S. and Barnes , S. H. 257 – 268 . Oxford : Clarendon Press .
- Gabarayeva , N. I. 1991b . The ultrastructure and development of exine and orbicules of Magnolia delavayi (Magnoliaceae) in the tetrad and the beginning of posttetrad periods . Botanicheski Zhurnal (Leningrad) , 76 : 10 – 19 . In Russian, with English summary
- Gabarayeva , N. I. 1993 . Hypothetical ways of exine pattern determination . Grana , 33 ( Suppl. 2 ) : 54 – 59 .
- Gabarayeva , N. I. 1995 . Pollen wall and tapetum development in Anaxagorea brevipes (Annonaceae): Sporoderm substructure, cytoskeleton, sporopollenin precursor particles, and the endexine problem . Review of Palaeobotany and Palynology , 85 : 123 – 152 .
- Gabarayeva , N. I. 2000 . “ Principles and recurrent themes in sporoderm development ” . In Pollen and spores: Morphology and biology , Edited by: Harley , M. M. , Morton , C. M. and Blackmore , S. 1 – 17 . Kent : Whitstable Printers Ltd, Whitstable .
- Gabarayeva , N. I. and El-Ghazaly , G. 1997 . Sporoderm development in Nymphaea mexicana (Nymphaeaceae) . Plant Systematics and Evolution , 204 : 1 – 19 .
- Gabarayeva , N. I. and Grigorjeva , V. V. 2002 . Exine development in Stangeria eriopus (Stangeriaceae): Ultrastructure and substructure, sporopollenin accumulation, the equivocal character of the aperture, and stereology of microspore organelles . Review of Palaeobotany and Palynology , 122 : 185 – 218 .
- Gabarayeva , N. I. and Grigorjeva , V. V. 2003 . Comparative study of the pollen wall development in Illicium floridanum (Illiciaceae) and Schisandra chinensis (Schisandraceae) . Taiwania , 48 : 147 – 167 .
- Gabarayeva , N. I. and Grigorjeva , V. V. 2004 . Exine development in Encephalartos altensteinii (Cycadaceae): Ultrastructure, substructure and the modes of sporopollenin accumulation . Review of Paleobotany and Palynology , 132 : 175 – 193 .
- Gabarayeva , N. , Grigorjeva , V. , Rowley , J. R. and Hemsley , A. R. 2009a . Sporoderm development in Trevesia burckii (Araliaceae). I. Tetrad period: Further evidence for participating of self-assembly processes . Review of Palaeobotany and Palynology , 156 : 211 – 232 .
- Gabarayeva , N. , Grigorjeva , V. , Rowley , J. R. and Hemsley , A. R. 2009b . Sporoderm development in Trevesia burckii (Araliaceae). II. Post-tetrad period: Further evidence for participating of self-assembly processes . Review of Palaeobotany and Palynology , 156 : 233 – 247 .
- Gabarayeva , N. I. and Hemsley , A. R. 2006 . Merging concepts: The role of self-assembly in the development of pollen wall structure . Review of Palaeobotany and Palynology , 138 : 121 – 139 .
- Gabarayeva , N. I. and Rowley , J. R. 1994 . Exine development in Nymphaea colorata (Nymphaeaceae) . Nordic Journal of Botany , 14 : 671 – 691 .
- Gerasimova-Navashina , E. N. 1973 . “ Physicochemical nature of primexine formation of angiosperm pollen grains ” . In Embryology of angiosperms , Edited by: Kovarski , A. 57 – 70 . Kishinev : Shtiintza . In Russian
- Guédès , M. 1982 . Exine stratification, ectexine structure and angiosperm evolution . Grana , 21 : 161 – 170 .
- Hamm , M. , Goldbeck-Wood , G. , Fraaije , J. G. E. M. and Zvelindowsky , A. V. 2004 . Nematic-amorphous polymer interfaces in the presence of a compatibilizer . Journal of Chemical Physics , 121 : 4430 – 4440 .
- Harley , M. M. 1990 . Occurrence of simple, tectate, monosulcate or trichotomosulcate pollen grains within the Palmae . Review of Palaeobotany and Palynology , 63 : 137 – 147 .
- Harley , M. M. 2004 . Triaperturate pollen in the monocotyledons: Configurations and conjectures . Plant Systematics and Evolution , 247 : 75 – 122 .
- Harley , M. M. and Baker , W. J. 2001 . Pollen aperture morphology in Arecaceae: Application with phylogenetic analyses, and a summary of the fossil record of palm-like pollen . Grana , 40 : 45 – 77 .
- Hemsley , A. R. , Collinson , M. E. and Brain , A. P. R. 1992 . Colloidal crystal-like structure of sporopollenin in the megaspore walls of recent Selaginella and similar fossil spores . Botanical Journal of the Linnean Society , 108 : 307 – 320 .
- Hemsley , A. R. , Collinson , M. E. , Kovach , W. L. , Vincent , B. and Williams , T. 1994 . The role of self-assembly in biological systems: Evidence from iridescent colloidal sporopollenin in Selaginella megaspore walls . Philosophical Transactions of the Royal Society of London B , 345 : 163 – 173 .
- Hemsley , A. R. , Collinson , M. E. , Vincent , B. , Griffiths , P. C. and Jenkins , P. D. 2000 . “ Self-assembly of colloidal units in exine development ” . In Pollen and spores: Morphology and biology , Edited by: Harley , M. M. , Morton , C. M. and Blackmore , S. 31 – 44 . Kent : Whitstable Printers Ltd, Whitstable .
- Hemsley , A. R. and Gabarayeva , N. I. 2007 . Exine development: The importance of looking through a colloid chemistry “window” . Plant Systematics and Evolution , 263 : 25 – 49 .
- Hemsley , A. R. , Jenkins , P. D. , Collinson , M. E. and Vincent , B. 1996 . Experimental modelling of exine self-assembly . Botanical Journal of the Linnean Society , 121 : 177 – 187 .
- Hemsley , A. R. , Lewis , J. and Griffiths , P. C. 2004 . Soft and sticky development: Some underlying reasons for microarchitectural pattern convergence . Review of Palaeobotany and Palynology , 130 : 105 – 119 .
- Hemsley , A. R. , Vincent , B. , Collinson , M. E. and Griffiths , P. C. 1998 . Simulated self-assembly of spore exines . Annals of Botany , 82 : 105 – 109 .
- Heslop-Harrison , J. 1968 . The pollen grain wall . Science , 161 : 230 – 237 .
- Heslop-Harrison , J. 1971a . Wall pattern formation in angiosperm microsporogenesis . Symposium of the Society for Experimental Biology , 25 : 277 – 300 .
- Heslop-Harrison , J. 1971b . “ The pollen wall: Structure and development ” . In Pollen development and physiology , Edited by: Heslop-Harrison , J. 75 – 98 . London : Butterworths .
- Heslop-Harrison , J. 1972 . Pattern in plant cell walls: Morphogenesis in miniature . Proceedings of Royal Institute of Great Britain , 45 : 335 – 351 .
- Heslop-Harrison , J. 1987 . Pollen germination and pollen tube growth . International Review of Cytology , 107 : 1 – 78 .
- Hesse , M. 1985 . Hemispherical surface processes of exine and orbicules in Calluna (Ericaceae) . Grana , 24 : 93 – 98 .
- Hesse , M. and Waha , M. 1983 . The fine structure of the pollen wall in Strelizia reginae (Musaceae) . Plant Systematics and Evolution , 141 : 285 – 298 .
- Kader , J. C. 1996 . Lipid transfer proteins in plants . Plant Molecular Biology , 47 : 627 – 654 .
- Kress , W. J. and Stone , D. E. 1982 . Nature of the sporoderm in monocotyledons, with special reference to the pollen grains of Canna and Heliconia . Grana , 21 : 129 – 148 .
- Levine , T. P. 2007 . A lipid transfer protein that transfers lipid . Journal of Cell Biology , 179 : 11 – 13 .
- Lodge , T. P. , Bang , J. , Li , Z. , Hillmyer , M. A. and Talmon , Y. 2005 . Strategies for controlling intra- and intermicellar packing in block copolymer solutions: Illustrating the flexibility of the self-assembly toolbox . Self-organising polymers. Faraday Discussions , 128 : 1 – 12 .
- Nadot , S. , Forchioni , A. , Penet , L. , Sannier , J. and Ressayre , A. 2006 . Links between early pollen development and aperture pattern in monocots . Protoplasma , 228 : 55 – 64 .
- Pozhidaev , A. E. 1998 . Hypothetical way of pollen aperture patterning. 1. Formation of 3-colpate patterns and endoaperture geometry . Review of Palaeobotany and Palynology , 104 : 67 – 83 .
- Pozhidaev , A. E. 2000 . Hypothetical way of pollen aperture patterning. 2. Formation of polycolpate patterns and pseudoaperture geometry . Review of Palaeobotany and Palynology , 109 : 235 – 254 .
- Pozhidaev , A. E. 2002 . Hypothetical way of pollen aperture patterning. 3. A family-based study of Krameriaceae . Review of Palaeobotany and Palynology , 127 : 1 – 23 .
- Rowley , J. R. 1990 . The fundamental structure of the pollen exine . Plant Systematics and Evolution Suppl. , 5 : 13 – 29 .
- Rowley , J. R. and Flynn , J. J. 1968 . Tubular fibrils and the ontogeny of the yellow water lily pollen grain . Cell Biology , 39 : 159
- Rowley , J. R and Flynn , J. J. 1991 . Tambourissa (Monimiaceae). Microspore development in the tetrad period . Annales Sciences Naturelles, Botanique, Paris, 13e Série , 11 : 125 – 147 .
- Rowley , J. R. and Morbelli , M. A. 2009 . Connective structures between tapetal cells and spores in Lycophyta and pollen grains in angiosperms. A review . Review of Palaeobotany and Palynology , 156 : 157 – 164 .
- Rowley , J. R. , Skvarla , J. J. and Pettitt , J. M. 1992 . Pollen wall development in Eucommia ulmoides (Eucommiaceae) . Review of Palaeobotany and Palynology , 70 : 297 – 323 .
- Sampson , F. B. 1977 . Pollen tetrads of Hedycarya arborea J. R. et G. Forst. (Monimiaceae) . Grana , 16 : 61 – 73 .
- Scott , R. J. 1994 . “ Pollen exine – the sporopollenin enigma and the physics of pattern ” . In Molecular and cellular aspects of plant reproduction Society for Experimental Biology Seminar Series 55 , Edited by: Scott , R. J. and Stead , M. A. 49 – 81 . Cambridge : Cambridge University Press .
- Sheldon , J. M. and Dickinson , H. G. 1983 . Determination of patterning in the pollen wall of Lilium henryi . Journal of Cell Science , 63 : 191 – 208 .
- Skvarla , J. J. and Rowley , J. R. 1986 . “ Canna generalis, the conjectured function of intine-like components ” . In Pollen and spores: Form and function , Edited by: Blackmore , S. and Ferguson , I. K. 397 – 399 . London : Academic Press .
- Skvarla , J. J. and Rowley , J. R. 1987 . Ontogeny of pollen in Poinciana (Leguminosae). I. Development of exine template . Review of Palaeobotany and Palynology , 50 : 239 – 311 .
- Southworth , D. and Jernstedt , J. A. 1995 . Pollen exine development precedes microtubule rearrangement in Vigna unguiculata (Fabaceae): A model for pollen wall patterning . Protoplasma , 187 : 79 – 87 .
- Stone , D. E. , Sellers , S. C. and Kress , W. J. 1979 . Ontogeny of exineless pollen in Heliconia, a banana relative . Annals of Missouri Botanical Gardens , 66 : 701 – 730 .
- Suárez-Cervera , M. , Asturias , J. A. , Vega-Maray , A. , Castells , T. , López-Iglesias , C. , Ibarolla , I. , Arilla , M. C. , Gabarayeva , N. and Seone-Camba , J. A. 2005 . The role of allergenic proteins Pla a 1 and Pla a 2 in the germination of Platanus acerifolia pollen grains . Sexual Plant Reproduction , 18 : 101 – 112 .
- Suárez-Cervera , M. , Le Thomas , A. , Goldblatt , P. , Márques , J. and Seone-Camba , J. A. 2000 . “ The channeled intine of Aristea major: Ultrastructural modifications during development, activation and germination ” . In Pollen and spores: Morphology and biology , Edited by: Harley , M. M. , Morton , C. M. and Blackmore , S. 57 – 71 . Kent : Whitstable Printers Ltd, Whitstable .
- Suárez-Cervera , M. , Vega-Maray , A. , Castells , T. , Rodríguez-Rajo , F. J. , Asturias , J. A. , Le Thomas , A. and Seone-Camba , J. A. 2008 . An approach to the knowledge of pollen and allergen diversity through lipid transfer protein localisation in taxonomically distant pollen grains . Grana , 47 : 272 – 284 .
- Takahashi , M. 1993 . Exine initiation and substructure in pollen of Caesalpinia japonica (Leguminosae: Caesalpinioidea) . American Journal of Botany , 80 : 192 – 197 .
- Theilade , I. and Theilade , J. 1996 . Ontogeny of pollen grains in Zingiber spectable (Zingiberacear) . Grana , 35 : 162 – 170 .
- Thomas , M. M. , Garwood , N. C. , Baker , W. J. , Henderson , S. A. , Russell , S. J. , Hodel , D. R. and Bateman , R. M. 2006 . Molecular phylogeny of the palm genus Chamaedorea, based on the low-copy nuclear genes PRK and RPB2 . Molecular Phylogenetics and Evolution , 38 : 398 – 415 .
- Thompson , D. A. 1917 . On growth and form , Cambridge, , UK : Cambridge University Press .
- Van Uffelen , G. A. 1991 . “ The control of spore wall formation ” . In Pollen and spores: Patterns of diversification , Edited by: Blackmore , S. and Barnes , S. H. 89 – 102 . Oxford : Clarendon Press .
- Wodehouse , R. P. 1935 . Pollen grains: Their structure, identification and significance in science and medicine , New York, , USA : McGraw-Hill Co., Inc .