Abstract
The parasitic mite Varroa destructor is a serious threat for western honey bee colonies and beekeepers are compelled to control it to keep their colonies healthy. Yet, by controlling varroa no resistance to the parasite can evolve. As a trial, honey bee colonies have been left untreated in isolated locations to allow development of resistance or tolerance to the mite. These colonies developed an ability to live without control measures against varroa, although the traits responsible for this resistance or tolerance are still unclear. Two of these resistant populations have been studied to test the involvement of specific varroa mite targeted hygienic behaviour varroa sensitive hygiene (VSH) in the acquired resistance. Individual mites were manually introduced into just capped brood cells, after which the brood combs were placed in colonies of the two resistant populations and in control colonies in which varroa had always been controlled. We followed the development of the mites, including possible removals. We found that VSH had increased strongly in one of the selections, up to 40% of the infested cells with mites and pupae were removed, but it had decreased in the other selection, compared to the control colonies. Further we could not conclude from our data that VSH only or preferentially targets reproducing mites, leaving non-reproducing mites undisturbed. The different VSH responses between the two selected resistant honey bee populations lead to conclude that more than one mechanism of resistance may evolve in response to the selection pressure by varroa mites.
El ácaro parásito Varroa destructor es una seria amenaza para las colonias occidentales de abejas melíferas y los apicultores se ven obligados a controlarlo para mantener sus colonias sanas. Sin embargo, al controlar la varroa no puede evolucionar hacia ninguna resistencia al parásito. Como ensayo, se han dejado sin tratar colonias de abejas en lugares aislados para permitir el desarrollo de resistencia o tolerancia al ácaro. Estas colonias desarrollaron una capacidad para vivir sin medidas de control contra la varroa, aunque los rasgos responsables de esta resistencia o tolerancia aún no están claros. Dos de estas poblaciones resistentes han sido estudiadas para probar la implicación de una varroa específica con un comportamiento higiénico específico sensible a varroa (VSH por sus siglas en inglés) en la resistencia adquirida. Los ácaros individuales se introdujeron manualmente en celdas de cría recién operculadas, después de lo cual los cuadros de cría se colocaron en colonias de las dos poblaciones resistentes y en colonias de control en las que la varroa siempre se había controlado. Seguimos el desarrollo de los ácaros, incluyendo posibles retiradas. Se encontró que el carácter VSH había aumentado fuertemente en una de las seleccionadas, hasta el 40% de las celdas infestadas con ácaros y pupas fueron eliminadas, pero había disminuido en la otra seleccionada, en comparación con las colonias de control. Además, no se pudo concluir de nuestros datos que VSH actúe sólo o preferencialmente en ácaros reproductivos, dejando ácaros no reproductores sin ser molestados. Las diferentes respuestas de VSH entre las dos poblaciones de abejas resistentes seleccionadas llevan a concluir que más de un mecanismo de resistencia puede evolucionar en respuesta a la presión de selección por ácaros varroa.
Keywords:
Introduction
The parasitic mite Varroa destructor is thought to be one of the main factors responsible for the annual colony losses of the western honey bee Apis mellifera (Neumann & Carreck, Citation2010; Potts et al., Citation2010). Although the mite does not directly kill the bees, it has significant effects by feeding from the haemolymph of the pupae and the adult bees which can cause problems. When infection occurs at the larval stage, it leads to abnormal organ development and lower body weight of emerging bees (Bowen-Walker & Gunn, Citation2001; van Dooremalen et al., Citation2013). Furthermore, mite infestation weakens bees’ immune defences (Yang & Cox-Foster, Citation2005) and transmits viruses (Boecking & Genersch, Citation2008). Together these effects can damage individual bees and subsequently the whole colony (Boecking & Genersch, Citation2008; van Dooremalen et al., Citation2012; Rosenkranz, Aumeier, & Ziegelmann, Citation2010). The original host of the varroa mite is the Asian honey bee A. cerana. The A. mellifera host is more vulnerable to the parasite than the original A. cerana host, due to the much shorter co-evolutionary history of the host-parasite relation. Despite considerable effort, controlling varroa in managed A. mellifera colonies is still done by chemical treatments against the mites, leading to some cases of acaricide resistance as well as to toxicity towards the bees (Berry, Hood, Pietravalle, & Delaplane, Citation2013). In contrast, few populations of honey bee colonies of A. mellifera left unmanaged show clear signs of varroa resistance and some of them have been used for further breeding (reviewed in Locke, Citation2015).
Resistance against a parasite is defined as the ability of limiting the parasite burden (Raberg, Graham, & Read, Citation2009). Bees are social insects and are therefore more susceptible to disease outbreaks than solitary insects (Fefferman, Traniello, Rosengaus, & Calleri, Citation2007). In response to this increased risk, they have evolved collective defences such as allo-grooming (bees cleaning each other) and hygienic behaviour (Cremer, Armitage, & Schmid-Hempel, Citation2007; Wilson-Rich, Spivak, Fefferman, & Starks, Citation2009). Hygienic behaviour was first described by Rothenbuhler (Citation1964), in the context of American foulbrood disease, as the removal of disease-killed brood from the combs by worker bees. In the case of varroa infestation, the detection and removal of infected brood is achieved at the pupal stage thereby reducing or preventing the mite’s reproduction (Boecking & Drescher, Citation1991; Harbo & Harris, Citation2005).
This behaviour has been named differently: “removal response” (Rath & Drescher, Citation1990), “removal behaviour” (Boecking & Drescher, Citation1991), “varroa-specific hygienic behaviour” (Boecking, Bienefeld, & Drescher, Citation2000), “suppressed mite reproduction” (Harbo & Harris, Citation2005), and “varroa sensitive hygiene” (VSH) (Harris, Citation2007). In this paper, we use the latter term. Bees performing this VSH behaviour are able to recognise olfactory cues released by parasitized brood (Mondet et al., Citation2015; Schoning et al., Citation2012). VSH behaviour contributes to limit the population growth of V. destructor in both A. cerana and A. mellifera colonies (Boecking & Drescher, Citation1991; Rath & Drescher, Citation1990).
In Europe, a naturally resistant population on the island of Gotland, Sweden, has been developed successfully by ceasing mite treatment in infested colonies (Fries, Imdorf, & Rosenkranz, Citation2006). It appears that these colonies can reduce V. destructor population growth without the need of mite treatments. Comparable to the population in Gotland, Tjeerd Blacquière of Wageningen University & Research (unpublished results) started in 2007 and 2008 in the Netherlands to select for surviving colonies in which varroa control was ceased: two populations of honey bee colonies were subjected to cohabitation with varroa. The first one (Amsterdamse Waterleidingduinen, here called the AWD group), was built from a mixture of 70 Dutch hybrid colonies from which 20 were used as a control (population C, treated against the mite twice a year with oxalic acid) and 50 as the starting group to cohabitate with varroa. The second group (Tiengemeten, called the T group) originated from a mixture of Dutch bees and Gotland offspring. Every year, colonies that survived winter, increased in size and produced drones in spring were kept to breed and to produce the following generation (Kruitwagen, van Langevelde, van Dooremalen, & Blacquière, Citation2017). The different groups of colonies were kept in remote areas during mating (T on the island Tiengemeten, AWD in the Amsterdamse Waterleidingduinen, C in Lelystad, all in the Netherlands). The main traits of selection used were the ability of the colonies to grow rapidly (colony growth rate has been determined as a significant predictor of colony success; Meixner, Kryger, & Costa, Citation2015) and to survive winter despite the presence of V. destructor, and then again to develop well in spring.
After significant losses during the first years, the size of the untreated populations became stable and the colonies now have constant low levels of mite infestation, namely varying between 5 and 13% of phoretic mites in broodless conditions (Kruitwagen et al., Citation2017). This stable lowered parasite pressure occurring without treatment led us to consider the populations AWD and T as resistant against V. destructor. However, the mechanisms behind the mite resistance are still unclear. Kruitwagen et al. (Citation2017) investigated grooming behaviour at individual, group and colony level, but they did not find differences between the control population and the two selected populations. Understanding the mechanisms behind resistance to varroa can contribute to the understanding of the host-parasite relationship and could ultimately help to prevent colony losses.
In this study, we tested whether VSH significantly contributes to resistance against V. destructor in the two selected populations. To estimate its contribution to the resistance in these populations, we artificially infested honey bee brood with varroa mites. We hypothesized that (1) VSH would be significantly higher in the resistant populations than in the control and (2) the remaining infested pupae would carry more non-reproductive mites.
Materials and methods
VSH is estimated by calculating the removal rates of parasitised brood, which is sometimes found to correlate with the removal of dead brood, either freeze-killed or pin-killed brood (Boecking & Drescher, Citation1992; Büchler, Berg, & Le Conte, Citation2010; Rinderer, Harris, Hunt, & de Guzman, Citation2010). The methods using dead brood have the advantage of being less time consuming and do not require the capture and introduction of living mites. Yet, the detection of dead brood and the detection of parasitized brood are likely to be triggered by different, highly specific mechanisms (Mondet et al., Citation2016; Schoning et al., Citation2012). For this reason, we chose to use an artificial mite introduction method where we introduced female adult mites in recently capped brood cells and assessed the removal rates.
Honey bee colonies
For this experiment, five colonies of each population under selection (AWD, T) and five control colonies (C), with the queen cohort of 2014, were placed into single boxes of 8 frames and brought to the Grebbedijk apiary, nearby the river Rhine in Wageningen, the Netherlands (51°57′04.0″N, 5°38′07.5″E). The experiment took place from 10th July to 4th September 2015.
Neutral brood
For our artificial mite introduction method, we needed mite-free brood from colonies that were apparently free of varroa resistance traits. Therefore, we used a group of 5 Buckfast colonies with sister queens placed in sets of two storeys of 10 frames each. These colonies had in previous years always been treated against mites, the last treatment had been in December 2014, using Perizin (Coumaphos). The queen was kept in the upper box using a queen excluder. Several times a week, the hives were opened and frames with enough eggs were moved to the lower box to obtain frames with brood of the same stage. From 6 h before mite introductions, these frames were inspected and the cells in the process of being capped were identified and marked on a plastic sheet. This allowed the clear identification of freshly capped cells and only cells capped within the last 6 h were selected for introduction of mites.
Rearing of V. destructor
V. destructor can change its cuticular hydrocarbons in order to mimic its host’s smell (Le Conte et al., Citation2015). Therefore, mites transferred from one colony to another might excrete the smell of the source colony and might be identified faster by hygienic bees than mites that emerged in their own colony. To avoid this bias, all the mites used for the artificial infestation were collected from external mite rearing colonies. Ten colonies of varroa-sensitive honey bees were used exclusively for mite rearing purposes: five “mite shower” colonies (MS) with a high number of phoretic mites, and five companion colonies (CC) with a high number of mites in the reproductive phase (Figure ). These colonies were placed in hives of two storeys of ten frames each, with a queen excluder separating the two storeys. No treatment against V. destructor was applied and emerging drone brood from several sources was added in spring to increase the mite’s population. Queens were always kept in the upper part of the colony. In order to force phoretic mites in the MS to stay on adult bees, frames were exchanged between the MS and CC colonies every seven days. Frames with open brood were moved from the MS to the CC and in return frames with capped brood were moved from the CC to the MS. In all colonies, the brood was placed and kept in the lower storeys to avoid frames with brood in different stages. While mites from the MS were forced to stay phoretic, mites present in the CC were free to enter the brood cells.
Collection of mites
Icing sugar was used to detach mites from adult honey bees. It is known that icing sugar can be easily cleaned by honey bees without harming them and gives satisfactory results: 93% of the mites dislodge from their hosts (Macedo, Wu, & Ellis, Citation2002). About 300 bees were collected from the MS colonies and placed in a jar with a lid of which the centre part was replaced by a 2 mm hardware mesh. A table spoon of powdered sugar was added in the jar through the mesh and the jar was rolled so all the bees were covered with sugar. After 1 or 2 min, the jar was turned upside down over a wide plastic box and shaken (Dietemann et al., Citation2013). The fallen mites were collected and the icing sugar was removed using a moist paintbrush. Mites were placed in a container with a moist tissue to prevent them from desiccating until they were introduced into the brood cells, maximally 2 h later. When introduction had to be delayed due to waiting for the bees to cap brood cells, mites were kept on pupae to allow them to feed.
Introduction of mites into the brood cells
In a total of 30 frames (one in each of the 15 tested colonies, with two replications), 30 cells capped for maximally 6 h were selected for mite introduction. In each cell, an opening was made at the side of the capping using a razor blade (Figure (a)). Mites showing an active behaviour were chosen preferentially and introduced in the cells using a paintbrush (Figure (b)). Cells were closed by pushing the cap down (Figure (c)). To ensure the optimal temperature of the brood during the introduction of the mites, frames were kept outside their colonies as short as possible. In case of cool weather, the introduction operation was regularly paused and frames were put into boxes with worker bees for warming up.
Figure 2. Introducing mites in a honey bee brood cell by first (a) opening of the cells, (b) introducing a Varroa destructor mite, and (c) closing of the cells.
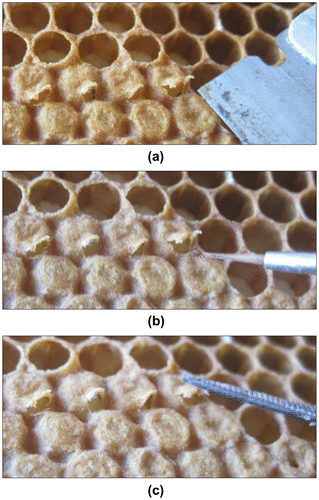
The precise locations of the infested cells were drawn on a transparent sheet placed above the comb. On this sheet, the corners of the frame and the cells with no brood were drawn as points of reference. Frames were then returned into their original Buckfast colonies, where workers sealed the cells properly. During the next day, the success (or acceptance rate) of the manual infestation was assessed and the frames were moved into colonies of the tested populations (T, AWD or C).
Infested frames were taken out of the colonies 10 or 11 days after capping, so each frame spent 9 or 10 days within the tested colony and was removed before emergence of the young bees. Whether the pupae had been removed or not was recorded, and remaining cells were opened to assess whether the mite had reproduced.
Statistical analysis
We considered each cell as an independent sampling unit. We first tested the differences in probability that the pupa was either removed (1) or not removed (0) between the three groups of colonies (T, AWD and C). We used a Generalised Linear Mixed Model (GLMM) with a binary distribution and a logit link function. We had multiple cells per colony, so we selected “Colony” and “Date” as random factor, with scaled identity as the random covariance structure (the best model with the lowest AICc), and “Group” as the fixed factor. The GLMM was followed by the LSD post hoc test to determine the pairwise differences between the groups. Second, we tested the differences in probability that the mite did (1) or did not (0) reproduce in the cells that had not been removed between the groups. Again, we used a GLMM with a binary distribution and a logit link function. The same random and fixed factors were used, and we found that again scaled identity as the random covariance structure provided the best model. The data analysis was performed using the IBM SPSS Statistics software version 23.
Results
Among 800 attempts, 750 cells (241 in the population C, 253 in the population T and 256 in the population AWD) distributed over 27 frames were successfully infested with varroa mites. In total, we found that 218 pupae were removed: 68 in C, 40 in T, and 110 in AWD. We found significant differences in removal probability between the different populations (GLMM, F2,747 = 15.48, p < 0.001). The colonies from AWD had the highest removal probability, whereas the colonies from T had the lowest (Figure ). We also found significant differences in the probability that the varroa mites did not reproduce in the cells that were not removed by the bees between the populations (GLMM, F2,529 = 3.45, p = 0.032). The control and T colonies had the highest probability that remaining mites did not reproduce, whereas the colonies from AWD had the lowest probability (Figure ).
Figure 3. Measured rates in which cells containing a varroa mite were removed by the honey bees of the three populations based on a Generalised Linear Mixed Model with a binary distribution and a logit link function (see text for statistics). C refers to the control population, T to the colonies from Tiengemeten and AWD to Amsterdamse Waterleidingduinen. Letters above bars indicate differences between the populations.
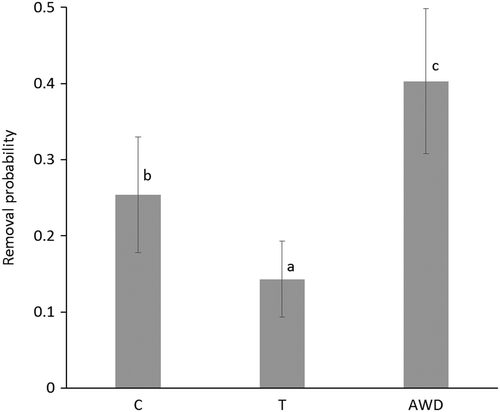
Figure 4. Measured rates in which varroa mites did not reproduce in the cells that were not removed by the bees in the three populations based on a Generalised Linear Mixed Model with a binary distribution and a logit link function (see text for statistics). C refers to the control population, T to the colonies from Tiengemeten and AWD to Amsterdamse Waterleidingduinen. Letters above bars indicate differences between the populations.
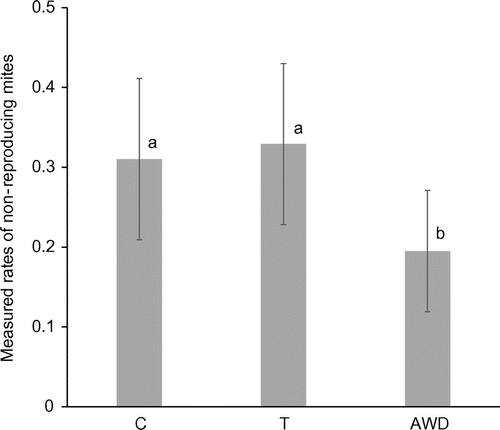
Discussion
The aim of this experiment was to test whether VSH is involved as a defence mechanism in colonies that had been selected for their resistance to the parasitic mite V. destructor. VSH is known to be used as defence mechanism against artificially introduced varroa mites by the Asian bee A. cerana (Rath & Drescher, Citation1990). A. cerana can detect and remove up to 98.8% of experimentally infested brood. A recent study highlighted an increased susceptibility of A. cerana immature workers to varroa infestation, leading to high mortality and triggering high removal rates (Page et al., Citation2016). This forces varroa to only reproduce in drone brood, since the susceptibility of worker brood as well as the hygienic removal from it prevents the mite from successfully reproducing in worker brood. These mechanisms keep the mite population from becoming very large and could explain part of the resistance in A. cerana (Evans & Spivak, Citation2010). VSH has also been observed in non-selected A. mellifera, but to a limited extend (Aumeier, Rosenkranz, & Gonçalves, Citation2000; Boecking & Ritter, Citation1993; Boecking & Spivak, Citation1999; Guzmán-Novoa, Vandame, & Arechavaleta, Citation1999). Colonies selected specifically for VSH are able to remove a high percentage of infested brood (Harbo & Harris, Citation2007). We hypothesised that the resistant colonies of Amsterdamse Waterleidingduinen and Tiengemeten populations also used VSH as a main trait of resistance, and predicted that these varroa-resistant colonies would have higher removal rates of infested pupae than colonies of the control population and that more of the remaining infested pupae would carry non-reproductive mites.
The results of the analysis of the removal rates show indeed a significant difference between the populations: the removal probability is the highest in the Amsterdamse Waterleidingduinen population and the lowest in the Tiengemeten population. The control population had intermediate values. The mean removal rate of the Amsterdamse Waterleidingduinen population was relatively high compared to other studies performed with non-selected honey bees (Rosenkranz et al., Citation2010), which supports our first hypothesis. We therefore conclude that VSH in the Amsterdamse Waterleidingduinen colonies is a trait that is at least partly responsible for the resistance developed by these colonies over the years. These colonies were not selected specifically for VSH, such as the VSH honey bees selected by the USDA in the United States of America and by other groups in Europe (Büchler et al., Citation2010; Harbo & Hoopingarner, Citation1997), which illustrates that VSH can evolve under the selection pressure created by V. destructor. The removal rates in the Tiengemeten colonies were rather low, even lower than those of the control colonies that are susceptible to Varroa. The removal rates found in the control colonies were similar as found by Boecking et al. (Citation2000), who also used artificially infested brood in varroa-susceptible colonies. In the end, in our selections around 60 cells out of 100 remain untouched in AWD, while 75 cells remain in C and 86 cells in T.
In 2004, Martin and Medina modelled the unexpectedly high varroa resistance of Africanised honey bees. The outcomes of their study showed that a reduction of the mite fecundity from 0.92 per cycle in the western honey bee to 0.73 in the Africanized honey bee was enough to bring the mite population below the threshold for collapse of the colony (Martin & Medina, Citation2004). Applied to our data and considering the reproductive rate to be 1.2 fertile daughters per cycle (Rosenkranz et al., Citation2010) and if 25% of the mites are removed by VSH (like in the control colonies), the average number of fertile daughter mites per cycle is 0.75 × 1.2 = 0.9 in the control. When 40% of the mites are removed by VSH (like in the colonies of the Amsterdamse Waterleidingduinen), the outcome is 0.72, which brings the mite pressure below the threshold of the model of Martin and Medina (Citation2004).
To test whether VSH bees removed preferentially reproducing mites, we collected data about remaining mites’ reproduction. The results do not confirm our hypothesis: in the population with the lowest VSH (Tiengemeten), 3% of the mites found were non-reproducing mites, whereas in the population with the highest VSH (Amsterdamse Waterleidingduinen), 2% of the mites found were not reproducing, compared to 3% in the control.
If we assume that only reproducing mites plus pupae are removed, the expected original % of non-reproducing mites in the Buckfast brood cells (without interference of VSH) can be calculated: 28% in the TG (14% gone (Figure ), of the 86% left 33% non-reproducing (Figure ): 0.33 × 86 = 28%), 23% in C (25% gone, 31% non-rep: 0.31 × 75 = 25%) and 12% in AWD (40% gone, 0.20 × 60 = 12%). Non-reproduction in the range from 12–28% is close to the 5–20% reported before (Rosenkranz et al., Citation2010). If we assume that VSH workers did remove the cells with mites irrespective they were reproducing or not, we expect the percentage found in the non-removed cells to be the same as originally present in the artificially infested cells, the range being 20–33% (Figure ). The variable ranges found suggest that there may have been variation in the percentage of reproducing mites in the cells apart from the interference by VSH worker bees, unfortunately we did not include a check for the percentage of reproduction in cells kept in an incubator. This obviously present variation implies at least that it is possibly erroneous to derive the VSH qualities of a colony from the percentage of non-reproducing mites found in the brood.
In the resistant colonies of Gotland, the average percentage of reproducing mites was 48% compared to 78% in the control varroa-sensitive colonies (Locke & Fries, Citation2011). The varroa-resistant population of Avignon shows similar results: 59% of mites reproduce within brood of resistant colonies, while 90% do in the control population (Locke, Conte, Crauser, & Fries, Citation2012). The high share of non-reproducing mites in the Avignon and Gotland populations may have been caused by reduced reproduction of the mites as Locke et al. (Citation2012) suggest, but may equally well in part have been caused by removal of reproducing mites by varroa sensitive hygienic worker bees. In their study, no correlation with pin-test induced hygienic behaviour and resistance was found, but it is still doubtful whether the hygienic behaviour on pin-killed brood shows any relationship with VSH.
The removal rates in the Tiengemeten colonies were lower than we expected. In previous years, these colonies were able to maintain low varroa loads without beekeeping intervention (Kruitwagen et al., Citation2017). Our finding highlights the existence of another mechanism acting to reduce the mite infestation. In addition to VSH, grooming behaviour was assessed in the Amsterdamse Waterleidingduinen, Tiengemeten and the control colonies, but grooming was not found to be significantly higher in the varroa-resistant colonies (Kruitwagen et al., Citation2017). Added to active defences such as VSH and grooming, the suppression of mite reproductive success, triggered by inhibition of egg laying, could have evolved (Garrido & Rosenkranz, Citation2003; Locke, Citation2016; Milani, Della Vedova, & Nazzi, Citation2004). In 2011 and 2012, a reduced fecundity was found for both the Amsterdamse Waterleidingduinen and Tiengemeten populations in comparison with the control (Tjeerd Blacquière, Wageningen, unpublished results). Moreover, a high percentage of mother mites failed to produce male offspring, leaving all the female offspring unmated and thus lost for the next generation.
The pressure caused by V. destructor infestation forced the two isolated populations, probably through a bottleneck, to develop resistance traits towards the parasite. As we only found VSH being prominent in one of the two selected populations, Amsterdamse Waterleidingduinen, we suggest that natural selection led to different mechanisms of varroa resistance within a rather short period of time. Resistant populations in Brazil, South Africa and eastern Russia use hygienic behaviour and grooming as main mechanisms while other populations in Sweden, France and Russia use mechanisms reducing the mite’s reproduction (Locke, Citation2016). It could be that under different conditions and in colonies with a different genetic make-up and history, different traits would be the solution to reduce the varroa load while minimising the fitness cost of resistance (Coustau, Chevillon, & Ffrench-Constant, Citation2000). Moreover, multiple underlying trades-off are still unknown (Meunier, Citation2015; Pirk, Strauss, Yusuf, Démares, & Human, Citation2015; Raberg et al., Citation2009) and the benefits of current line-selection programs can be questioned in the perspective of long term fitness of honey bee populations (Fries & Bommarco, Citation2007; Neumann & Blacquière, Citation2016.)
With this study, we made a step forward in the understanding of the development of resistance in honey bee colonies against V. destructor through natural selection. Further investigation into the regulation and impacts of resistance traits on host-parasite co-evolution, also including the interactions with viruses (McMenamin & Genersch, Citation2015), is crucial to further understand how low and non-impacting varroa loads can be reached in honey bee colonies.
Disclosure statement
No potential conflict of interest was reported by the authors.
Funding
TB acknowledges the Dutch Ministry of Economic Affairs and the European Commission for Financial support (Project NP 14-6.1/NL 2013f).
Author contributions
DP and TB designed the research and carried out the experiments, DP, TB and FvL analysed the data, and all authors wrote the paper.
Acknowledgements
We thank Dr Willem Boot and Dr Johan Calis for their Mite Shower + Companion Colony concept (see Figure 1) and for the capped drone brood frames for the start-up of it in spring.
References
- Aumeier, P., Rosenkranz, P., & Gonçalves, L.S. (2000). A comparison of the hygienic response of Africanized and European (Apis mellifera carnica) honey bees to Varroa-infested brood in tropical Brazil. Genetics and Molecular Biology, 23, 787–791.10.1590/S1415-47572000000400013
- Berry, J.A., Hood, W.M., Pietravalle, S., & Delaplane, K.S. (2013). Field-level sublethal effects of approved bee hive chemicals on honey bees (Apis mellifera L). PLoS ONE, 8, e76536.10.1371/journal.pone.0076536
- Boecking, O., Bienefeld, K., & Drescher, W. (2000). Heritability of the Varroa-specific hygienic behaviour in honey bees (Hymenoptera: Apidae). Journal of Animal Breeding and Genetics, 117, 417–424.10.1046/j.1439-0388.2000.00271.x
- Boecking, O., & Drescher, W. (1991). Response of Apis mellifera L. colonies infested with Varroa jacobsoni Oud. Apidologie, 22, 237–241.10.1051/apido:19910308
- Boecking, O., & Drescher, W. (1992). The removal response of Apis mellifera L. colonies to brood in wax and plastic cells after artificial and natural infestation with Varroa jacobsoni Oud. and to freeze-killed brood. Experimental and Applied Acarology, 16, 321–329.10.1007/BF01218574
- Boecking, O., & Genersch, E. (2008). Varroosis—The ongoing crisis in bee keeping. Journal für Verbraucherschutz und Lebensmittelsicherheit, 3, 221–228.10.1007/s00003-008-0331-y
- Boecking, O., & Ritter, W. (1993). Grooming and removal behaviour of Apis mellifera intermissa in Tunisia against Varroa jacobsoni. Journal of Apicultural Research, 32, 127–134. doi:10.1080/00218839.1993.1110129710.1080/00218839.1993.11101297
- Boecking, O., & Spivak, M. (1999). Behavioral defenses of honey bees against Varroa jacobsoni Oud. Apidologie, 30, 141–158.10.1051/apido:19990205
- Bowen-Walker, P.L., & Gunn, A. (2001). The effect of the ectoparasitic mite, Varroa destructor on adult worker honey bee (Apis mellifera) emergence weights, water, protein, carbohydrate, and lipid levels. Entomologia Experimentalis et Applicata, 101, 207–217.10.1046/j.1570-7458.2001.00905.x
- Büchler, R., Berg, S., & Le Conte, Y. (2010). Breeding for resistance to Varroa destructor in Europe. Apidologie, 41, 393–408.10.1051/apido/2010011
- Coustau, C., Chevillon, C., & Ffrench-Constant, R.H. (2000). Resistance to xenobiotics and parasites: Can we count the cost? Trends in Ecology & Evolution, 15, 378–383.10.1016/S0169-5347(00)01929-7
- Cremer, S., Armitage, S.A., & Schmid-Hempel, P. (2007). Social immunity. Current Biology, 17, R693–R702.10.1016/j.cub.2007.06.008
- Dietemann, V., Nazzi, F., Martin, S.J., Anderson, D., Locke, B., Delaplane, K.S., … Ellis, J.D. (2013). Standard methods for varroa research. In V. Dietemann, J.D. Ellis, & P. Neumann (Eds.), The COLOSS BEEBOOK, Volume II: standard methods for Apis mellifera pest and pathogen research. Journal of Apicultural Research, 52(1). doi:10.3896/IBRA.1.52.1.09
- Evans, J.D., & Spivak, M. (2010). Socialized medicine: Individual and communal disease barriers in honey bees. Journal of Invertebrate Pathology, 103, S62–S72.10.1016/j.jip.2009.06.019
- Fefferman, N.H., Traniello, J.F., Rosengaus, R.B., & Calleri, D.V., II. (2007). Disease prevention and resistance in social insects: Modeling the survival consequences of immunity, hygienic behavior, and colony organization. Behavioral Ecology and Sociobiology, 61, 565–577.10.1007/s00265-006-0285-y
- Fries, I., & Bommarco, R. (2007). Possible host-parasite adaptations in honey bees infested by Varroa destructor mites. Apidologie, 38, 525–533.10.1051/apido:2007039
- Fries, I., Imdorf, A., & Rosenkranz, P. (2006). Survival of mite infested (Varroa destructor) honey bee (Apis mellifera) colonies in a Nordic climate. Apidologie, 37, 564.10.1051/apido:2006031
- Garrido, C., & Rosenkranz, P. (2003). The reproductive program of female Varroa destructor mites is triggered by its host, Apis mellifera. Experimental & Applied Acarology, 31, 269–27310.1023/B:APPA.0000010386.10686.9f
- Guzmán-Novoa, E., Vandame, R., & Arechavaleta, M.E. (1999). Susceptibility of European and Africanized honey bees (Apis mellifera L.) to Varroa jacobsoni Oud. in Mexico. Apidologie, 30, 173–18210.1051/apido:19990207
- Harbo, J.R., & Harris, J.W. (2005). Suppressed mite reproduction explained by the behaviour of adult bees. Journal of Apicultural Research, 44, 21–23. doi:10.1080/00218839.2005.1110114110.1080/00218839.2005.11101141
- Harbo, J.R., & Harris, J.W. (2009). Responses to varroa by honey bees with different levels of varroa sensitive hygiene. Journal of Apicultural Research, 48, 156–161. doi:10.3896/IBRA.1.48.3.0210.3896/IBRA.1.48.3.02
- Harbo, J.R., & Hoopingarner, R.A. (1997). Honey bees (Hyntenoptera: Apidae) in the United States that express resistance to Varroa jacobsoni (Mesostigmata: Varroidae). Journal of Economic Entomology, 90, 893–898.10.1093/jee/90.4.893
- Harris, J.W. (2007). Bees with varroa sensitive hygiene preferentially remove mite infested pupae aged ≤ five days post capping. Journal of Apicultural Research, 46, 134–139. doi:10.1080/00218839.2007.1110138310.1080/00218839.2007.11101383
- Kruitwagen, A., van Langevelde, F., van Dooremalen, C., & Blacquière, T. (2017). Naturally selected honey bee (Apis mellifera) colonies resistant to Varroa destructor do not groom more intensively. Journal of Apicultural Research, 56(4). doi:10.1080/00218839.2017.132979710.1080/00218839.2017.1329797
- Le Conte, Y., Huang, Z.Y., Roux, M., Zeng, Z.J., Christidès, J.P., & Bagnères, A.G. (2015). Varroa destructor changes its cuticular hydrocarbons to mimic new hosts. Biology Letters, 11, 20150233.10.1098/rsbl.2015.0233
- Locke, B. (2015). Natural Varroa mite-surviving Apis mellifera honey bee populations. Apidologie, 47, 467.
- Locke, B. (2016). Inheritance of reduced Varroa mite reproductive success in reciprocal crosses of mite-resistant and mite-susceptible honey bees (Apis mellifera). Apidologie, 47, 583–588.10.1007/s13592-015-0403-9
- Locke, B., Conte, Y.L., Crauser, D., & Fries, I. (2012). Host adaptations reduce the reproductive success of Varroa destructor in two distinct European honey bee populations. Ecology and Evolution, 2, 1144–1150.10.1002/ece3.248
- Locke, B., & Fries, I. (2011). Characteristics of honey bee colonies (Apis mellifera) in Sweden surviving Varroa destructor infestation. Apidologie, 42, 533–542.10.1007/s13592-011-0029-5
- Macedo, P.A., Wu, J., & Ellis, M.D. (2002). Using inert dusts to detect and assess varroa infestations in honey bee colonies. Journal of Apicultural Research, 41, 3–7. doi:10.1080/00218839.2002.1110106210.1080/00218839.2002.11101062
- Martin, S.J., & Medina, L.M. (2004). Africanized honey bees have unique tolerance to Varroa mites. Trends in Parasitology, 20, 112–114.10.1016/j.pt.2004.01.001
- McMenamin, A.J., & Genersch, E. (2015). Honey bee colony losses and associated viruses. Current Opinion in Insect Science, 2015, 121–129.10.1016/j.cois.2015.01.015
- Meixner, M.D., Kryger, P., & Costa, C. (2015). Effects of genotype, environment, and their interactions on honey bee health in Europe. Current Opinion in Insect Science, 10, 177–184.10.1016/j.cois.2015.05.010
- Meunier, J. (2015). Social immunity and the evolution of group living in insects. Philosophical Transactions of the Royal Society B: Biological Sciences, 370, 20140102.10.1098/rstb.2014.0102
- Milani, N., Della Vedova, G., & Nazzi, F. (2004). (Z)-8-Heptadecene reduces the reproduction of Varroa destructor in brood cells. Apidologie, 35, 265–273.10.1051/apido:2003064
- Mondet, F., Alaux, C., Severac, D., Rohmer, M., Mercer, A.R., & Le Conte, Y. (2015). Antennae hold a key to Varroa-sensitive hygiene behaviour in honey bees. Scientific Reports, 5, S96.10.1038/srep10454
- Mondet, F., Kim, S.H., de Miranda, J.R., Beslay, D., Le Conte, Y., & Mercer, A.R. (2016). Specific cues associated with honey bee social defence against Varroa destructor infested brood. Scientific Reports, 6, 523.10.1038/srep25444
- Neumann, P., & Blacquière, T. (2016). The darwin cure for apiculture? Evolutionary Applications: Natural selection and managed honey bee health.
- Neumann, P., & Carreck, N.L. (2010). Honey bee colony losses. Journal of Apicultural Research, 49(1), 1–6. doi:10.3896/IBRA.1.49.1.0110.3896/IBRA.1.49.1.01
- Page, P., Lin, Z., Buawangpong, N., Zheng, H., Hu, F., Neumann, P., … Dietemann, V. (2016). Social apoptosis in honey bee superorganisms. Scientific Reports, 6, S62.10.1038/srep27210
- Pirk, C.W., Strauss, U., Yusuf, A.A., Démares, F., & Human, H. (2015). Honey bee health in Africa—A review. Apidologie, 47, 1–25.
- Potts, S.G., Biesmeijer, J.C., Kremen, C., Neumann, P., Schweiger, O., & Kunin, W.E. (2010). Global pollinator declines: Trends, impacts and drivers. Trends in Ecology & Evolution, 25, 345–353.10.1016/j.tree.2010.01.007
- Raberg, L., Graham, A.L., & Read, A.F. (2009). Decomposing health: Tolerance and resistance to parasites in animals. Philosophical Transactions of the Royal Society B: Biological Sciences, 364, 37–49.10.1098/rstb.2008.0184
- Rath, W., & Drescher, W. (1990). Response of Apis cerana Fabr. towards brood infested with Varroa jacobsoni Oud. and infestation rate of colonies in Thailand. Apidologie, 21, 311–321.10.1051/apido:19900406
- Rinderer, T.E., Harris, J.W., Hunt, G.J., & de Guzman, L.I. (2010). Breeding for resistance to Varroa destructor in North America. Apidologie, 41, 409–424.10.1051/apido/2010015
- Rosenkranz, P., Aumeier, P., & Ziegelmann, B. (2010). Biology and control of Varroa destructor. Journal of Invertebrate Pathology, 103, S96–S119.10.1016/j.jip.2009.07.016
- Rothenbuhler, W.C. (1964). Behaviour genetics of nest cleaning in honey bees. I. Responses of four inbred lines to disease-killed brood. Animal Behaviour, 12, 578–583.10.1016/0003-3472(64)90082-X
- Schoning, C., Gisder, S., Geiselhardt, S., Kretschmann, I., Bienefeld, K., Hilker, M., & Genersch, E. (2012). Evidence for damage-dependent hygienic behaviour towards V. destructor-parasitised brood in the western honey bee, A. mellifera. Journal of Experimental Biology, 215, 264–271.10.1242/jeb.062562
- van Dooremalen, C., Gerritsen, L., Cornelissen, B., van der Steen, J.J., van Langevelde, F., & Blacquière, T. (2012). Winter survival of individual honey bees and honey bee colonies depends on level of Varroa destructor infestation. PLoS ONE, 7, e36285.10.1371/journal.pone.0036285
- van Dooremalen, C., Stam, E., Gerritsen, L., Cornelissen, B., van der Steen, J., van Langevelde, F., & Blacquière, T. (2013). Interactive effect of reduced pollen availability and Varroa destructor infestation limits growth and protein content of young honey bees. Journal of Insect Physiology, 59, 487–493.10.1016/j.jinsphys.2013.02.006
- Wilson-Rich, N., Spivak, M., Fefferman, N.H., & Starks, P.T. (2009). Genetic, individual, and group facilitation of disease resistance in insect societies. Annual Review of Entomology, 54, 405–423.10.1146/annurev.ento.53.103106.093301
- Yang, X., & Cox-Foster, D.L. (2005). Impact of an ectoparasite on the immunity and pathology of an invertebrate: Evidence for host immunosuppression and viral amplification. Proceedings of the National Academy of Sciences, 102, 7470–7475.10.1073/pnas.0501860102