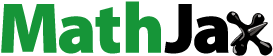
Abstract
This study explored the potential for an environmentally friendly method for refining difficult-to-solubilise noble metals (notably platinum). Conventional noble-metal refining processes rely on the use of strong inorganic acids and oxidants, which cause significant environmental impact. To overcome these challenges, we propose a non-aqueous direct leaching process using an ionic liquid, an organic acid, and a chlorinating agent. Our findings indicate that trihexyl(tetradecyl)phosphonium chloride (P66614Cl) in combination with methanesulfonic acid and trichloroisocyanuric acid effectively solubilises metallic platinum. We investigated the leaching mechanism using ultraviolet–visible spectroscopy and confirmed that Pt(0) was oxidised to Pt(IV) to form PtCl62−. Recovery of Pt from Pt-loaded P66614Cl was achieved by stripping with ammonium nitrate solution. This work advances the development of sustainable refining methods for noble metals.
1. Introduction
Noble metals (NMs), such as gold, palladium, and platinum (Pt), are used as industrial catalysts and in electronic components. However, their relative scarcity and uneven distribution pose worldwide political and economic challenges that render them costly. Therefore, not only primary but also secondary resources have attracted attention for a more stable and sustainable supply of NMs (Zupanc et al. Citation2023). In particular, NM recovery from secondary resources, such as waste electric and electronic equipment, provides advantages in terms of CO2 emissions (Rocchetti et al. Citation2013).
Conventional hydrometallurgical refining processes, which use strong inorganic acids and oxidants such as aqua regia to dissolve chemically inert NMs, are associated with environmental concerns due to the generation of large quantities of acidic wastewaters and usage of volatile organic solvents. However, the recent development of solvents such as ionic liquids (ILs) and deep eutectic solvents has made it possible to eliminate the use of volatile organic solvents in solvent-extraction systems (Dwamena Citation2019; Wongsawa et al. Citation2020). Additionally, processes such as adsorption (Nikoloski et al. Citation2015), membrane separation (Fontàs et al. Citation2007), and precipitation (Matsumoto et al. Citation2021), offer alternatives to solvent extraction in metal separation. Despite these efforts to develop environmentally friendly methods for metal separation, research on benign leaching of NMs has yet to make significant progress; hence, alternative methods for NM dissolution have been pursued, particularly the use of non-aqueous solvent systems as a replacement for harmful inorganic acids, in an effort to achieve more sustainable and selective dissolution of NMs (Li and Binnemans Citation2021).
Studies have explored the use of halogen–halide polar organic solvent systems (Nakao Citation1992 Citation1999), dihalogen–sulphur-containing ligand adducts (Cau et al. Citation2003), aromatic thiol ligands (Räisänen et al. Citation2007), “organic aqua regia” formed by thionyl chloride and pyridine (Lin et al. Citation2010), and various oxidant–ligand systems for the solubilisation of NMs. However, only a few studies have reported successful dissolution of Pt in non-aqueous systems. Sulphur-containing triiodide ligands (Bigoli et al. Citation2001), water-immiscible solvents, and HCl–oxidant mixtures (Nguyen et al. Citation2022) have been proposed as means of dissolving Pt. Although trihalide species are promising oxidants for NMs in organic solvents and ILs, solubilising Pt remains challenging, partly due to the loss of reactive trihalide species at elevated temperatures (van den Bossche et al. Citation2021). Moreover, the complexity of solvent and ligand synthesis hinders the economic viability of NM refining.
In this study, we focused on the facile and benign dissolution of Pt into ILs, with the synergistic combination of organic acids and chlorinating agents to generate chlorine gas in situ for efficient Pt dissolution. A phosphonium-based IL was used as the solvent owing to its high capacity for platinum extraction (Firmansyah et al. Citation2020). The superiority of this leaching solvent was exhibited by comparison with water and conventional organic solvents. The dissolution mechanism of Pt into ILs was investigated by kinetic analysis and ultraviolet–visible (UV–vis) spectroscopy. Recovery of Pt from the IL was demonstrated via stripping with various aqueous solutions.
2. Experimental
2.1 Chemicals
Platinum powder, 28% ammonia, and thiourea were purchased from Fujifilm Wako Chemical Ltd., Japan. Trihexyl(tetradecyl)phosphonium chloride (P66614Cl) was purchased from Sigma-Aldrich Co., USA. Trichloroisocyanuric acid (TCCA) and methanesulfonic acid (MSA) were purchased from Tokyo Chemical Industry Co., Ltd., Japan. n-Dodecane, 1-propanol, 5 M nitric acid, 99% ammonium nitrate, and 5 M hydrochloric acid were purchased from Kishida Chemical Co., Ltd., Japan. Milli-Q water was used to prepare the series of aqueous solutions used in this work.
2.2 Method for Leaching of Pt Powder
The effects of additive agents, leaching solvent, and leaching time on Pt dissolution were investigated. Undiluted P66614Cl was used as the leaching solvent. After screening several combinations of chlorinating agents and organic acids, TCCA and MSA were used as additive agents to enhance Pt dissolution. All experiments were performed at a solid-to-liquid ratio of 0.5 g/L, stirring at 400 rpm at 353 K for 24 h.
After leaching, the resulting mixture was completely separated into the solvent and residue components by centrifugal separation at 20 000 g for 10 min at 298 K. The Pt concentration in the leaching solvent was measured by an inductively coupled plasma optical emission spectrometer (Optima 8300, PerkinElmer, USA). Leaching performance (%L) was measured using the following equation:
(1)
(1)
where
is the concentration of metal M in the leaching solvent after reaction and
is the concentration of metal M assuming all Pt powder is leached. UV–vis spectra of the leaching solvents were measured at 298 K using a UV–vis spectrometer (JASCO V-750, Japan).
2.3 Method of Pt Ion Stripping from Leach Solution
To strip Pt from the solvent, an aqueous phase containing the stripping reagent was vigorously mixed with the leach solution at a volume ratio of 2:1 using a vortex mixer at 298 K for 24 h. The two solutions were then completely separated by centrifugation at 10,000 g for 1 min at 298 K. Stripping performance (%S) was measured using the following equation:
(2)
(2)
where
is the concentration of metal M in the leaching solvent after reaction and
is the concentration of metal M in the leaching solvent after stripping.
3. Results and Discussion
3.1 Impact of additive agents
Metallic Pt is much less soluble than metallic gold, even in aqua regia (Shibata et al. Citation2001). To overcome this challenge, TCCA and MSA were used as additive agents in this study. TCCA has been previously used to dissolve metallic gold in acetone with the aid of quaternary ammonium salts (Feng et al. Citation2020), while MSA has been lauded for its biodegradability and low volatility, making it an environmentally friendly organic acid (Binnemans and Jones Citation2023). P66614Cl, a hydrophobic IL, has been used as an efficient extractant for NM ions (Nguyen et al. Citation2016). Based on these prior findings, we hypothesised that the combination of undiluted IL, TCCA, and MSA would form an effective leaching solvent for metallic Pt.
The impact of these additive agents on the leaching performance of Pt is shown in . We found that Pt powder was barely leached using either the IL alone or the IL with MSA, and only slightly dissolved in the IL with TCCA. The minor dissolution of Pt in the IL with TCCA was attributed to the slight generation of chlorine gas from TCCA as an oxidant. Remarkably, when the IL was combined with TCCA and MSA, over 75% of Pt was efficiently leached. The oxidation of Pt with TCCA appeared to be augmented by MSA, which facilitated the efficient solubilisation of Pt into the IL.
3.2 Impact of solvent
The impact of different solvents on leaching was investigated. Leaching efficiencies of Pt with TCCA and MSA were evaluated using four different solvents: water, 1-propanol, n-dodecane, and the IL P66614Cl. The results are presented in . Pt was scarcely leached by water, 1-propanol, or n-dodecane, even with the addition of TCCA and MSA. These results suggest that P66614Cl plays a crucial role in Pt leaching, potentially by stabilizing the anion complex of dissolved Pt through the P66614 cation (Nguyen et al. Citation2016). Furthermore, when the reaction vials containing the first three leaching solvents were opened after leaching, a gas was emitted. This observation suggests that a chlorine gas, acting as an oxidant for Pt, was produced by reaction with TCCA and MSA, but was not consumed for Pt oxidation in these solvents.
3.3 Kinetics of leaching of Pt powder
shows the variation in leaching efficiency with time. The leaching efficiency was 80% after 24 h and increased to 90% after 48 h. EquationEquations (3)(3)
(3) and Equation(4)
(4)
(4) , which are frequently used in kinetic analyses of metal leaching based on the shrinking-core model (Cao et al. Citation2006), were used to determine the leaching mechanism:
(3)
(3)
(4)
(4)
where
is the kinetic constant,
is the reaction time, and
is the leaching efficiency, respectively.
Figure 3. Variation in leaching efficiency with time. Solvent: P66614Cl. Additive agents: MSA 13.8 wt.%, TCCA 5.3 wt.%. Conditions: 0.5 g/L, 353 K, 400 rpm, 1–48 h.
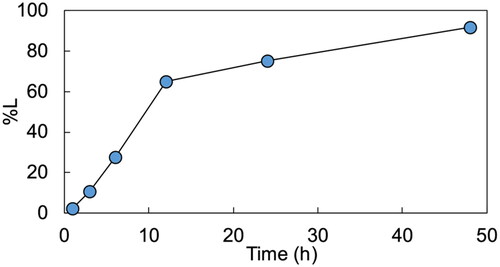
The kinetic plots for EquationEqs. (3)(3)
(3) and Equation(4)
(4)
(4) are shown in . Notably, the linearity was greater when fitting the data to EquationEq. (3)
(3)
(3) . Therefore, it was suggested that the leaching rate is controlled by diffusion-controlled reactions. The reason for the reaction proceeding in a diffusion-controlled reaction is due to the high viscosity of the IL.
3.4 Mechanism of Pt leaching in ionic liquid
The leaching mechanism of Pt by P66614Cl-TCCA-MSA system was investigated via UV–vis spectroscopy. UV–vis spectra of the IL phase before and after leaching, along with that of an aqueous PtCl62- standard solution for reference, are shown in . The spectra of IL after leaching (red line) and Pt standard solution (green dotted line) exhibited comparable peaks at around 260 nm, indicating the presence of PtCl62− (Glebov et al. Citation2015). Therefore, the metallic Pt was successfully oxidised from Pt(0) to Pt(IV) and formed PtCl62−, which was stabilised by the IL cation.
3.5 Stripping of Pt ion from leaching solvent
Owing to the hydrophobic nature of the IL, the recovery of Pt and regeneration of the IL could be easily achieved by stripping using an appropriate aqueous solution. In this study, Pt was stripped from the IL using 5% NH3, 5 M HNO3, 1 M thiourea with 1 M HCl, and 10 M NH4NO3. Stripping efficiencies of Pt using the various stripping agents are shown in . When 5% NH3, 5 M HNO3, and 1 M thiourea with 1 M HCl were used, the Pt stripping efficiency was less than 50%; conversely, 10 M NH4NO3 achieved a Pt stripping efficiency of up to 83%. UV–vis measurements were conducted to elucidate the mechanism. The UV–vis spectra of the IL phase (before and after stripping) and that of the aqueous NH4NO3 solution are shown in . Compared with the spectrum of the IL before stripping (red line), the peak at 260 nm decreased, and a peak at 250 nm and a broad peak around 300 nm appeared in the spectrum of the IL after stripping (blue line). The latter is specific to nitrate ions which occurred in the aqueous NH4NO3 solution. Therefore, it was confirmed that the mechanism for PtCl62− stripping is an anion-exchange reaction with nitrate ions.
4. Conclusion
This study successfully demonstrated the leaching of metallic Pt using the phosphonium IL P66614Cl as a solvent, along with TCCA and MSA as additive agents. The use of the IL as the solvent, combined with the two different additive agents, was found to be critical for the efficient leaching of Pt. Pt that dissolved in the IL was efficiently stripped using 10 M NH4NO3, with the mechanism confirmed based on UV–vis spectroscopy to be an anion-exchange reaction with nitrate ions. Pt is widely used in various fields, especially in automotive exhaust catalysts, so the proposed method would be applicable to the refining of secondary resources.
Acknowledgements
This study was supported by the Environment Research and Technology Development Fund (Grant No. 3-2004) provided by the Ministry of Environment of Japan. We thank Kathryn Sole, PhD, from Edanz (https://jp.edanz.com/ac) for editing a draft of this manuscript.
Disclosure statement
No potential conflict of interest was reported by the author(s).
References
- Bigoli F, Deplano P, Mercuri ML, Pellinghelli MA, Pintus G, Serpe A, Trogu EF. 2001. N,N′-dimethylpiperazinium-2,3-dithione triiodide, [Me2pipdt]I3, as a powerful new oxidation agent toward metallic platinum. Synthesis and x-ray structures of the reagent and the product [Pt(Me2pipdt)2](I3)2 [15]. J Am Chem Soc. 123:1788–1789. doi: 10.1021/ja0056015.
- Binnemans K, Jones PT. 2023. Methanesulfonic acid (MSA) in hydrometallurgy. In J Sustain Metall. Springer Science and Business Media Deutschland GmbH. 9:26–45. doi: 10.1007/s40831-022-00641-6.
- Cao Y, Harjanto S, Shibayama A, Naitoh I, Nanami T, Kasahara K, Okumura Y, Fujita T. 2006. Kinetic study on the leaching of Pt, Pd and Rh from automotive catalyst residue by using chloride solutions. Mater Trans. 47:2015–2024. doi: 10.2320/matertrans.47.2015.
- Cau L, Deplano P, Marchiò L, Mercuri ML, Pilia L, Serpe A, Trogu EF. 2003. New powerful reagents based on dihalogen/N,N′- dimethylperhydrodiazepine-2,3-dithione adducts for gold dissolution: the IBr case. Dalton Trans. 10:1969–1974. doi: 10.1039/B210281A.
- Dwamena AK. 2019. Recent advances in hydrophobic deep eutectic solvents for extraction.Separations. 6:9. doi: 10.3390/separations6010009.
- Feng F, Sun Y, Rui J, Yu L, Liu J, Zhang N, Zhao M, Wei L, Lu C, Zhao J, et al. 2020. Study of the “oxidation-complexation” coordination composite ionic liquid system for dissolving precious metals. Applied Sciences. 10:3625. doi: 10.3390/app10103625.
- Firmansyah ML, Yoshida W, Hanada T, Goto M. 2020. Application of ionic liquids in solvent extraction of platinum group metals. Solvent Extr Res Dev. 27:1–24.
- Fontàs C, Anticó E, Vocanson F, Lamartine R, Seta P. 2007. Efficient thiacalix[4]arenes for the extraction and separation of Au(III), Pd(II) and Pt(IV) metal ions from acidic media incorporated in membranes and solid phases. Sep Purif Technol. 54:322–328. doi: 10.1016/j.seppur.2006.10.003.
- Glebov EM, Pozdnyakov IP, Plyusnin VF, Khmelinskii I. 2015. Primary reactions in the photochemistry of hexahalide complexes of platinum group metals: a minireview. J Photochem Photobiol C. 24:1–15. doi: 10.1016/j.jphotochemrev.2015.05.003.
- Li X, Binnemans K. 2021. Oxidative dissolution of metals in organic solvents. Chem Rev. 121:4506–4530. doi: 10.1021/acs.chemrev.0c00917.
- Lin W, Zhang RW, Jang SS, Wong CP, Hong J i 2010. Organic aqua regia-powerful liquids for dissolving noble metals. Angew Chem Int Ed Engl. 49:7929–7932. doi: 10.1002/anie.201001244.
- Matsumoto K, Sezaki Y, Hata Y, Jikei M. 2021. Selective recovery of platinum (Iv) from HCl solutions using 2-ethylhexylamine as a precipitant. Separations. 8:40. doi: 10.3390/separations8040040.
- Nakao Y. 1992. Dissolution of noble metals in halogen-halide-polar organic solvent systems. J Chem Soc Chem Commun. 5:426–427. doi: 10.1039/C39920000426.
- Nakao Y. 1999. One-step syntheses of polyhalogenometal complexes by direct dissolution of the metals in halogen-halide-acetonitrile systems. Chem Lett. 28:433–434. doi: 10.1246/cl.1999.433.
- Nguyen VNH, Song SJ, Lee MS. 2022. Separation of palladium and platinum metals by selective and simultaneous leaching and extraction with aqueous/non-aqueous solutions. Hydrometallurgy. 208:105814. doi: 10.1016/j.hydromet.2021.105814.
- Nguyen VT, Lee JC, Chagnes A, Kim MS, Jeong J, Cote G. 2016. Highly selective separation of individual platinum group metals (Pd, Pt, Rh) from acidic chloride media using phosphonium-based ionic liquid in aromatic diluent. RSC Adv. 6:62717–62728. doi: 10.1039/C6RA09328K.
- Nikoloski AN, Ang KL, Li D. 2015. Recovery of platinum, palladium and rhodium from acidic chloride leach solution using ion exchange resins. Hydrometallurgy. 152:20–32. doi: 10.1016/j.hydromet.2014.12.006.
- Räisänen MT, Kemell M, Leskelä M, Repo T. 2007. Oxidation of elemental gold in alcohol solutions. Inorg Chem. 46:3251–3256. doi: 10.1021/ic0624468.
- Rocchetti L, Vegliò F, Kopacek B, Beolchini F. 2013. Environmental impact assessment of hydrometallurgical processes for metal recovery from WEEE residues using a portable prototype plant. Environ Sci Technol. 47:1581–1588. doi: 10.1021/es302192t.
- Shibata J, Gonomaru S, Yamamoto H. 2001. Leaching velocity of precious metals with various leaching agents. Kagaku Kogaku Ronbunshu. 27:367–372. doi: 10.1252/kakoronbunshu.27.367.
- van den Bossche A, Rodriguez Rodriguez N, Riaño S, Dehaen W, Binnemans K. 2021. Dissolution behavior of precious metals and selective palladium leaching from spent automotive catalysts by trihalide ionic liquids. RSC Adv. 11:10110–10120. doi: 10.1039/D1RA00695A.
- Wongsawa T, Traiwongsa N, Pancharoen U, Nootong K. 2020. A review of the recovery of precious metals using ionic liquid extractants in hydrometallurgical processes. Hydrometallurgy. 198:105488. doi: 10.1016/j.hydromet.2020.105488.
- Zupanc A, Install J, Jereb M, Repo T. 2023. Sustainable and selective modern methods of noble metal recycling. Angew Chem Int Ed. 62:e202214453. doi: 10.1002/anie.202214453.