ABSTRACT
To allow different views on motor learning to inform rehabilitation research, the authors aimed to explicate a frequently missed yet fundamental difference in starting point of such views. By considering how rehabilitation in practice answers the question of what parts an activity consists of, reductive and emergent approaches to motor learning are identified and traced throughout rehabilitation practice. The authors show that when a task is cut up along reductive dimensions while also apparently relying on emergent components, this unequally favors the reductive approach and acts to limit the views on motor learning available. By showing the approaches in practice, the authors hope to inspire an awareness that brings both approaches the opportunity to independently inform research so that new theories and practices can proliferate.
The ultimate goal of rehabilitation is to improve a patient's activities in daily life (ADL). To guide rehabilitation toward this goal there are many, and sometimes conflicting, theories of motor (re)learning (e.g., Newell, Liu, & Mayer-Kress, Citation2001; Wolpert, Citation1997). Having multiple differing theories available enables researchers and practitioners to take different perspectives and come up with new and fruitful ways of approaching rehabilitation problems.Footnote1 Such plurality should therefore be cherished. As we argue, however, the practical implementation of theories in rehabilitation unequally favors one perspective on motor learning and thus threatens this plurality. In this article we aim to start alleviating this threat by explicating two fundamentally different approaches to motor learning and the way each is implemented in rehabilitation. By doing so we hope to inspire awareness in the field to these differences and to explain the importance of allowing both views the autonomy to flourish and independently inform rehabilitation research.
To guide our discussion we will look at a practical question that any clinician has to consider: the question of whether an activity should be practiced as a whole or whether it should be practiced in parts. When (re)learning an activity, a patient will often not be able to perform the task in one go. The task can for example be too complex or a certain part of the task might be too painful or difficult. Thus, in therapeutic exercise the question of whether an activity should be practiced in parts or as a whole is an important one. Central to our discussion moreover, will be to consider along which dimensions to cut up an activity if it needs to be practiced in parts—that is, the question of what we count as a part of an activity. A reasonable approach to the problem can be found in many undergraduate textbooks (e.g., Edwards, Citation2010; Magill, 2003; see also Naylor & Briggs, Citation1963). Many have suggested that one practices an activity as a whole if it does not have meaningful parts with attainable subgoals and one can practice an activity in parts when it does. For example, in cyclic activities such as walking or cycling, and more generally, in any activity where there is a strong temporal relationship between the movements making up the activity, the activity should be practiced as a whole. In this approach the parts are thus identified by the dynamics of the activity and its subgoals. Subsequently we will identify this view as implying an emergent approach to motor learning.
Informed by the anatomical and physiological underpinnings of the movements that make up an activity, a more analytic approach compartmentalizes an activity in terms of underlying structures and aims to practice those parts in isolation before transferring them to the activity. For example, in rehabilitation following an upper limb amputation, patients often first learn to control their myoelectric (electromyography [EMG]) signals on a computer screen, before they apply this control to a myoelectric prosthesis (Dawson, Carey, & Fahimi, Citation2011). When patients are unable to perform a temporally tightly coupled activity this approach to the part-whole relationship is often chosen. For example, robot-assisted stepping aims to practice the whole activity, by targeting its underlying stepping motions. We explicate this view as taking a reductive approach to motor learning. Although the emergent and reductive approaches to the part-whole relationship will equally stress their commitment to improving activities in daily life, they approach the problem of how to do so completely differently.
We aim to show that if we, in rehabilitation, remain unaware of this fundamental distinction and its implications and therefore cut up activities along reductive dimensions while also apparently relying on emergent components, in practice we de facto apply only the reductive approach. If choosing the appropriate parts is just a pragmatic choice this would be no problem. However, as we will argue, both views on the part-whole relationship imply fundamentally different perspectives on motor learning and each enables a multitude of distinct ways of furthering the approach of rehabilitation problems. Although the perspectives that we wish to explicate only show in practice, one could call them metatheoretical so as to discern them from the explicitly formulated methods, ideas and hypotheses of the scientific or therapeutic work itself.
A perspective, an angle of approaching motor learning problems in rehabilitation, enables and constrains the theories and practices that are available. Because of this constraining function that our perspective brings to both scientific and therapeutic practices we contend that it is imperative that both views are given equal room to develop. Although the reductive approach is a viable view and should be pursued as far as it can be taken, when applied without constraint, it risks drowning out equally viable alternatives. In the following, our main goal is to inspire an awareness of the two principally different approaches as they are applied to rehabilitation problems in order to make room for each to develop its ideas as far as they can be taken. We aim to inspire such awareness in all those participating in the field of motor rehabilitation—theorists, researchers, and practitioners alike. We do so by first explaining and exemplifying both approaches in theories and their implementation, and then comparing the perspectives on rehabilitation research directly. Before starting on this however, we start with defining some concepts that both approaches share.
COMMON GROUND: CLASSIFICATION OF FUNCTION
In the International Classification of Functioning, Disability and Health (ICF) body function denotes the properties of anatomical parts of the human body (World Health Organization, Citation2015). For example, the elbow's function is to flex and extend and a muscle's function is to contract. To improve motor control in ADL, rehabilitation training frequently targets these body functions. From this point of view training programs aim at the movements of body parts rather than on activities such as putting on a shirt. These training strategies have also been applied in training muscle force to improve climbing stairs (Skelton, Young, Greig, & Malbut, Citation1995), using robotic guidance to go through the arm motions of reaching (Kwakkel, Kollen, & Krebs, Citation2007), or training myocontrol for using a hand prosthesis (Smurr et al., 2008). Functions pertaining to aspects of movements (e.g., force, coordination or control), or their anatomical counterparts, (e.g., the joints and muscular tissue), will therefore be called body functions here.
On the other hand, training can focus on everyday tasks. Such training would be categorized in the ICF as activities. Examples of activities are picking up a cup or buttoning up a coat. Here, function pertains not to bodily movements, but to the task that the patient aims to accomplish with its activity. Patients practice goal-directed actions rather than perform (repetitive) movements (i.e., displacement of body parts). For example, teaching a prosthesis user to pick up a cup, by having him pick up cups (Romkema, Bongers, & van der Sluis, Citation2013) or training laparoscopic surgery by simulating a surgical task (Torkington, Smith, Rees, & Darzi, Citation2001; see also Haque & Srinivasan, Citation2006). Activities we therefore define here as the adaptive coordination of the whole body to attain the goal of a task (cf. Bernstein, Citation1996; Gibson, Citation1979; Reed, Citation1996; Newell & Vaillancour, Citation2001; Warren, Citation2006).
TWO WAYS OF RELATING BODY FUNCTIONS AND ACTIVITIES
Having introduced our two basic concepts, we need to look at the relation between them to identify a reductive and an emergent approach. If we look closely at motor learning in rehabilitation, we can discern two different ways of dealing with the relation between body functions and activities. First, activities can be taken to be reducible to body functions. That is, activities can be said to be explained by describing the totality of the body functions that underlie it. For example, body functions can be considered the cause of activities or activities can be considered to be made up of (constituted of) underlying body functions. Second, activities can be said to be emergent on body functions. In that case body functions and activities are considered to be nonreductively related. Body functions and activities can for example be considered as mutually constraining each other. Or they can be understood as different aspects of the same rehabilitation problem (see also Meijer & Roth, Citation1988).
Both the reductive and the emergent view on motor learning assume a layered structure in which activities belong to a higher level than the underlying body functions do.Footnote2 In general, taking a reductive stance on a subject matter then means that we understand or explain the behavior of a system at one level by looking at the basic underlying components and the relations between these components at a level below (Silberstein, 2002; for comparative issues within medical science see Ahn, Tewari, Poon, & Philips, Citation2006; Engel, Citation1977). By contrast, an emergent stance claims that the higher level has its own intrinsic dynamics that deserves attention in its own right. It denies that understanding the behavior of the underlying components is enough to understand the behavior at the level of the activity. Thus it aims to understand activities by looking at the dynamic at the level of activities itself. Thus, in this view, the underlying level may constrain the behavior at the higher level, but it does not dictate, prescribe, or explain it.
To phrase the two ways of relating the level of body functions and the activity level in terms of practicing an activity in parts: just as anatomical parts may be considered the proper parts of a human body, so too can body functions be considered the proper parts of the motions of a human body (e.g., the possible displacements of its body parts). In as much as an activity is reduced to nothing but a moving body, body functions can therefore also be considered the proper parts of an activity. Learning an activity by cutting it up into body functional units and training these units outside the context of the activity is thus consistent with a reductive approach. The proper parts of an activity can also be considered to be themselves smaller activities, with their own (sub)goals. That is, the component parts of an activity are then considered to be functional units of action that nest into one another to form the whole activity. Learning an activity by cutting the activity up into smaller units of action and practicing their goal attainment is consistent with an emergent approach. Note that the emergent view does not deny that body functions may be considered component parts of a (moving) body, it only denies that they are the relevant components to focus on when describing an activity.
We get into the details of the two different approaches and exemplify them both with respect to the part-whole relationship in the following two sections. The main point of these sections is to show how these different approaches to motor learning bear on theories and practices of rehabilitation and to show that in practice activities are often cut up into both body functional units and units of action. Through examples of therapeutic and research practices, in the subsequent section the implications of focusing on activities while actually cutting these activities up along body functional dimensions will be dealt with.
THE REDUCTIVE PERSPECTIVE: FOCUSING ON BODY FUNCTIONS
In this section we consider in some detail the reductive approach to the question of what counts as a part by looking at reductive theories and training programs. A reductive approach attributes the improvements at the level of activities to changes at the underlying level of body functions. Therefore the reductive approach to motor learning targets body functions, even though its therapeutic goal is to (re)learn an activity (i.e., at the level of ADL). This perspective on motor learning boils down to two assumptions: (a) activities are actually merely a collection of body functions and (b) motor learning is learning to control these body functions. Together these assumptions imply a hierarchy in structure (see ). This body-oriented perspective on learning motor skills thus in principle cuts up the task along a vertical axis, along the arrows of . Following the arrows the explanation of learning an activity is reduced to describing the behavior at lower levels of description.
FIGURE 1. The reductive perspective. The activity of picking up a cup using a prosthesis is considered to reduce to a set of body functions. For example, a collection of muscles, tendons, joints, and a certain EMG signal with properties such as speed (v), force, and direction. Learning to coordinate all these body functions is subsequently considered to be reducible to acquiring a control system that coordinates the body functions.
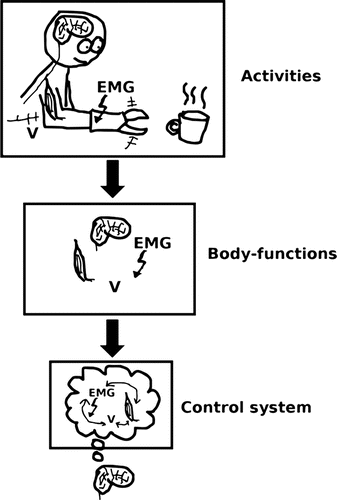
The Reductive Approach in Practice
The reductive perspective is actually the most dominant approach to motor learning in rehabilitation. To draw out the intricacies of the approach we will now highlight some examples of its theories and research areas within rehabilitation. First we discuss a general class of motor control theories that find application in rehabilitation. We will then offer two examples of the practices of reductive research programs: the field of robot-assisted walking and the field of serious gaming.
Internal Models
A particularly dominant reductive approach to motor learning is the approach that stems from motor program theories (e.g., Keele, Citation1968; Schmidt, Citation1975) that grew out of reflex arc concept of the nineteenth century and the computer metaphor of the 1950s. In this approach goals and activities are valued greatly, but only as representations in the minds of the patients. According to such theories (e.g., Krakauer, Citation2006; Wolpert, Citation1997), motor control is a (computational) process of planning a series of movements based on this represented goal-state. That is, after an environmental goal has been internalized, the (neural) system assembles the appropriate anatomical components (the muscles, the joints, or the limbs) and plans and monitors a movement pattern (i.e., sets and adjusts the appropriate velocity, torque, or power) that will move the body from its current position to the target position. At that point the current position will be identical to the represented goal state and therefore the activity has been performed. The activity in this view thus does not play a direct role in control, rather it supervenes on the underlying mechanism and the body functions it controls until the goal-state is reached.
This model of motor control has been extended and refined, but the premise is the same: activities are explained by their underlying components and interactions. A control system (e.g., an internal model) chooses and coordinates body functional parameters so that the body changes position in such a way that a goal is reached. Thus, it admits of a strongly reductive and hierarchical approach. The underlying body functions and the control system that coordinates them are together sufficient for accounting for motor control. Even the environmental goal of the activity is reduced to an internal (input) state for the underlying control system. By extension, motor learning is also approached in a strongly reductive manner. The key is to acquire a motor plan that chooses and coordinates the body functions appropriately (e.g., Dosen et al., Citation2015). Therefore, in this view the relevant parts of activities are their underlying body functions. Accordingly, motor learning would be fostered by interventions aimed at improving these body functions and, as we shall see, this is the approach employed.
Robot-Assisted Treadmill Walking
In rehabilitation following a spinal cord injury, a stroke or cerebral palsy, a patient's walking ability can be trained on a treadmill while an exoskeleton (a robot) supports the weight of the patient and guides her stepping movements (Duncan et al., Citation2011). The aim of such training programs is to improve a patient's walking ability in daily life. To do so, the training program adopts many thoroughly reductive tenets. First, it is assumed that the goal of a walking activity is circumstantial and can be dispensed with. That is, the patient simply does not need to go anywhere in the real world while on the treadmill. Rather the activity is taken to be reducible to its underlying stepping movements. Furthermore, it is assumed that it is inconsequential to the basic activity that these stepping movements neither generate nor make use of the perceptual (optic, proprioceptive) flow that accompanies walking in real life.
The robotic system treats any of these perceptual-motor dynamics as well as the (environmental) goal of the activity as if they are irrelevant to the basic activity. Furthermore, by having the robot do much of the work, many new perceptual-motor interactions are being introduced (Dobkin & Duncan, Citation2012). For example, even if the (perceptually impaired) patient can sense whether the robot is moving her leg or whether she is doing it herself, the patient's goal is now to get the leg moving correctly by learning to coordinate her effort in collaboration with the robot. As long as a stepping pattern is retained, however, the reductive logic of the training system implies that these added perceptual-motor dynamics are just as irrelevant to the activity as the dynamics they have replaced. In other words, the coordinative dynamics at the level of the activity itself is taken to be inconsequential to learning an activity—they can be dispensed with or can even be replaced, without changing the essence of the activity: its body functions.
Recently, Dobkin and Duncan (Citation2012) published a critical review on robot-supported and related training systems. They conclude that despite more than 20 years of development, the effect of robot supported treadmill training has been slim to none and go on to identify several possible reasons for this. Apart from the fact that the importance of central pattern generators (i.e., an underlying control system mentioned previously) in humans is questionable, they pointed out that the adaptive coordination at the level of the activity that is required for working the treadmill is completely different from that of walking in daily life. In terms of the part-whole relationship, despite the best efforts to maintain the whole activity, by simulating only the stepping movements used in daily life, the approach has cut the activity (i.e., walking) up along a body functional dimension and reduced it to an underlying part (i.e., stepping movements). Focusing on the task-specific dynamics at the level of activity shows that robot-assisted walking has reduced the activity so strongly that it has come to have very little to do with the original activity its sets out to improve.
Serious Gaming for Rehabilitation
A field that is heading in a similar direction is that of serious gaming for rehabilitation. Serious games are (video) games that are fun to play and offer challenging goals while supplying players with skills useful in reality (Graafland, Schraagen, & Schijven, Citation2012). Serious games are basically designed so that a body function used in ADL is given a fun and motivating role in a computer task. For example, when targeting the Center of Pressure (COP) that is found to be important in walking or sitting. In such games, the players need to actively displace their COP to pop virtual balloons (Gil-Gómez, Lloréns, Alcañiz, & Colomer, Citation2011). Likewise, the EMG signals required for using a myoelectric prosthesis are targeted and used to make players hit musical notes (Armiger & Vogelstein, Citation2008). Thus, the method of serious gaming in rehabilitation embodies the body-oriented approach.
Despite the fact that serious games aim to offer therapy by offering an activity, the logic of current serious gaming is thoroughly reductive and body function oriented. First, as in the robot-assisted stepping, a body function needed in an ADL task is taken out of that context and is practiced in a different (new and fun gaming) task. This step again crucially assumes that by isolating the underlying body function of an activity, the essence of that activity is retained. This reduction implies a hierarchy in which activities are the resultant of body functions, but body functions are not the resultant of activities. For example, one can sit or walk because the COP is adequately displaced, rather than vice versa. Second, assuming the reductive hierarchy, serious games can simply add a new and fun gaming goal to training the body functions. This step assumes that motor learning is a matter of learning to control these body functions—independently of the task or the goal for which they are used. The goal is merely an addition to the underlying control of body functions.
Using such serious games in therapeutic practice exemplifies a reductive view. In this view, the context in which a task is performed is considered to be incidental rather than essential to the activity that is learned. Thus for example, one can acquire an underlying faculty called balance control (Gil-Gómez et al., Citation2011) or the control of an EMG signal (myocontrol; Dawson et al., Citation2011; Dupont & Morin Citation1994; Gordon & Ferris, Citation2004) independently of the task in which such control is exhibited. This underlying faculty is assumed to allow for transfer of performance across activities. Implicitly thus, in these practices the part-whole relationship is again cut along hierarchical lines: the activity is taken to reduce to its underlying body functions and its control system.
In sum, to improve activities in daily life the reductive approach to motor learning cuts up the activities it aims to promote along its underlying componential structure. As we have seen in our examples of robot-assisted walking and serious gaming, such orientation toward underlying body functional components is not without consequences for the way training programs are designed. As an alternative, we will now look at the emergent view as an activity centered approach to motor learning. To answer the question of what a system's parts are, the approach does not cut up the activity into underlying elements, but into goal-directed units at the level of the activity itself.
THE EMERGENT APPROACH: KEEPING AN EYE ON ACTIVITIES
Our question of whether there are discernible parts to an activity and how to discern them can also be answered in another way. The intuition not to cut up cyclic or otherwise tightly coupled temporal processes, such as reaching and grasping during prehension shows this. It shows that the dynamics of the activity itself may be essential to learning that activity. The emergent view on motor learning expresses the conviction that when we artificially break up coordinative structures by stripping activities of the relation to their goal, and furthermore strip the bodily coordination down to some of its components to arrive at body functions, we do not get to the essence of the activity, but we lose it. In other words, the explanation of learning an activity is not sought below but within the activity's level of description. It implies therefore, that we ought to stick to the level of activities and try to establish what perceptual-motor dynamics align the patient to the environmental goal of the activity she performs and to what extent the activity can be meaningfully cut up into shorter bouts of activity with their own subgoals.
The Emergent Approach in Practice
To see how this emergent and thus activity-oriented perspective approaches motor learning and to further clarify the approach let us look at some examples of emergent theories and practices. We start this discussion with a brief overview of action system theory (Reed, Citation1982), and dynamic systems approaches to motor learning (e.g., Newell et al. (Citation2001). After that, we turn to the well-known task-oriented approach to stroke rehabilitation. As we argue, although this latter approach is sometimes misunderstood its background lies in the emergent view on motor learning.
The Theory of Action Systems
According to the theory of action systems (Reed, Citation1982, Citation1988), when a patient is learning to perform a task, she is forming an action system. In forming an action system she learns to coordinate her body to attain a specific goal in the environment. Action systems are thus not defined by their anatomical parts, but rather by their overall goal. Because of that, an action system is flexible and made up of nested units of perceiving and acting—each of which has its own subgoals. Learning an action system requires assembling and fine tuning the relations between these cycles of perceiving of, and acting on, environmental aspects relevant to the task at hand (Bingham, Coats, & Mon-Williams, Citation2007; Jacobs & Michaels, Citation2007). Because an action system emerges from tuning the relations between perceiving and acting on particular task aspects, the system becomes highly dependent on the structure of the task (Van Dijk & Bongers, Citation2014). Fine-tuning actions to this task structure is called calibration. In action system theory, transfer from one task to another is expected based on the ability to recalibrate an established action system to fit a novel task structure.
To give an example of the importance of maintaining the goal-relevant perceptual-motor dynamics, in a series of studies Rieser, Pick, Ashmead, and Garing (Citation1995) demonstrated calibration of action systems by having people walk on a treadmill that was being pulled by a tractor. When walking under these conditions the environment seems to move, or flow, past at a greater speed than would be expected during normal walking conditions. When participants are subsequently asked to walk (on the ground) to a target without using vision, they undershoot their target. That is, the tight temporal coupling between perceiving and acting is transferred from one situation to the next. Importantly, this effect of the calibration of action to the perceived rate of (optic) flow, transfers to tasks with similar goals such as side stepping, but not to tasks with a different goal such as turning in place or throwing a ball (Rieser et al., Citation1995). Action systems, in this case a system for locomotion, are thus calibrated to adapt to the specific way perception and action are attuned and, importantly, this calibration is specific to the goal of the activity and not specific to the underlying body functional structures (for further examples see e.g., Bingham et al., Citation2007; Bruggeman & Warren, Citation2010; Withagen & Michaels, Citation2002).
Because action systems are highly context sensitive and assembled relative to a goal, when a task or activity is too complex to perform at once, action system theory will preferably cut up the activity into subtasks. The performance of any of these subtasks needs to be relatively independent from the whole in terms of their dynamics, but not in terms of their goal. For example, in natural prehension, there is a tight coupling between reaching and grasping and for that reason these two aspects of performance should not be trained in isolation (Jeannerod, Citation1984). However, when looking at prehension as part of the activity of tooth brushing, getting the tooth paste can be practiced independently of unscrewing the lid or brushing the teeth (Reed, Montgomery, Palmer, & Pittenger, Citation1995). As Reed et al. exemplified, many activities in daily life can be cut up into shorter, yet meaningful, units of activity. When an aspect of an action system needs to be performed by different means, for example when prehension needs to be achieved using a myoelectric interface, these means need to be incorporated into such meaningful units. Action systems are not constituted by body functional units (i.e., by their [anatomical] means) but by the goal-directed dynamics of perceiving and acting. Therefore the theory suggests that incorporating these novel means into the original goal directed activity will lead to the biggest transfer effects on activities with similar (sub)goals. Hence the largest improvement in ADL too is expected when training goal-directed actions while incorporating novel means.
Dynamic System Approaches
Out of the insights that also fueled action system theory (Gibson, Citation1979) and combined with the theory of nonlinear dynamics (e.g., Kelso, Citation1997), dynamic system approaches to motor learning were born (Davids, Button, & Bennett, Citation2008; Newell et al., Citation2001; for similar developments, see also Carr & Shepherd, Citation1989; Law et al., Citation1996). These approaches also acknowledge the emergence of a coordinated activity, but stress how this coordination emerges from the self-organizing nonlinear dynamics of the interaction between patient, the task, and the environment. The interaction of these three aspects leads to a dynamic perceptual-motor landscape of possible movement patterns that allows the patient to attain the goal and, depending on the phase of learning, this landscape has different regions in which the movement patterns for attaining the goal of the activity are most stable (attractor states). Learning an activity is conceptualized as taking place through self-organization in which exploring and moving around will organize the dynamics of the interactions between patient, the task, and the environment until it stabilizes around such a stable attractor state. Thus, the performance of the activity emerges from the dynamics of the patient-task-environment system (Newell, Citation1986, Citation1996).
Now, because the activity is a self-organizing property that emerges from these interacting dynamics, in practice aspects of either the task, the environment or the patient act to constrain or enable certain dynamics, but they do not dictate them (cf. the reductive, body-oriented approach). When learning, the dynamics of the patient-task-environment system need to organize itself within the boundaries set up by the constraints in order to reach the goal of the activity. The job of the therapist is thus to shape any of these aspects so as reshape the constraints which nudges the self-organizing system toward stable regions.
Important to dynamic system approaches is that the training process itself is part of the dynamics and therefore modulates and changes the perceptual-motor landscape over time (Newell et al., Citation2001). That is, previous learning experience will continuously alter the shape and regions of stability in the landscape. Thus, the type and intensity of training will constrain the opportunities for learning. In fact, the dynamics of patient-task-environment of any performance are nested within the larger scale dynamics of learning and development. An interesting implication of this is that, although body functions do not dictate performance, they can be made to (appear to) do so. For example, when training to isolate EMG patterns for learning to use a myoelectric prosthesis, this experience carves out an attractor in the perceptual-motor landscape that will constrain subsequent prosthesis performance. This of course offers opportunities for application, but should also give us pause: If we choose to target aspects based on body-oriented rather than activity-oriented considerations, we may end up with a system that performs great on body-oriented outcome measures but that is better adapted to the narrow confines of the lab than to the ever-changing and context-sensitive reality of activities in daily life.
Task-Oriented Training
Based on the previously mentioned theories and ideas very close to them, the emergent approach to motor learning has found practical application in the form of task-oriented training (e.g., Winstein & Wolf, 2009). This training form has gained popularity in the field of neurorehabilitation—especially in rehabilitation training after a stroke. Task-oriented training brings some of the insights of action system and dynamic system theories to rehabilitation and centers on the idea that limitations in activity need to be targeted rather than impairments of body functions. That is, it aims to improve the attainment of the goal of a task, rather than a focus on the bodily means to do so. For example, a task-oriented training program for improving the ability to reach for objects following a stroke showed that goal directed grasping to reach objects led to improved reaching performance as shown by arm kinematics and on an activity-level questionnaire. By contrast, resistance training that displaced the arm across similar distances did not lead to improved reaching performance (Thielman, Dean, & Gentile, Citation2004). In task-oriented training practitioners aim to challenge patients into achieving real, ADL-like, goals. The training program thus favors activities over body functions. Although not widely applied outside stroke rehabilitation and easily recast or applied in reductive terms (for this worry, see also Winstein & Wolf, 2009), the effects of task-oriented training have been promising (e.g., Rensink, Schuurmans, Lindeman, & Hafsteinsdottir, Citation2009; Wevers et al., Citation2009).
What all these examples of approaching motor learning share from an emergent viewpoint is an assumption that the coordination found at the level of activities is an emergent property that can only be understood by looking at the dynamics of the unfolding activity itself. That is, activities cannot be reduced to underlying structures and their relations. In terms of the part-whole relationship: the parts of an activity are functional units at the level of the activity rather than the movements of underlying anatomical components. If research resists focusing on body functions in favor of explaining motor learning in terms of the coordinative dynamics of the activity itself, we propose it takes an emergent perspective. An emergent perspective attributes the improvements in performance of an activity to changes in its dynamics at the level of activities itself. So, while the reductive approach is shaped by a belief that in the end, activities will reduce to body functions, the emergent approach takes this belief to be unfounded (Silberstein, Citation2002). From such a perspective, the level of activities needs to be studied in its own right—by looking at the details of the dynamical relations between parts of the body and their relation to the goal that should be achieved. If an activity needs to be cut up for training purposes, it needs to be compartmentalized in units of goal-directed actions.
TOWARD PLURALITY IN METHODS
The question of what the relevant parts of an activity are, as we have seen, is not just a pragmatic question, but it is a deep conceptual issue of which the answer has far reaching consequences for approaching rehabilitation problems. As we have seen from our examples of theories and training methods, any rehabilitation training program has dealt, explicitly or implicitly, with the question. We have argued that a reductive view approaches this question hierarchically and goes down a level to identify parts of the whole, while the emergent view approaches the question by looking around at the level of activity itself to identify its parts. Having exemplified both views, we can see how both approaches view each other's methods. This will show why the reductive view easily dominates the context sensitive emergent perspective.
Reduction Dominant Methods
We have seen that the reductive view is a viable view and that it should be pursued as far as it can be taken—and so should the emergent view. However, there is a strong asymmetry between both views that should be avoided. This asymmetry in practice causes the reductive approach to drown out the emergent approach. Thus an apparent focus on training an activity combined with a body functional decomposition (e.g., robot-assisted treadmill walking), is actually only a reductive program. To see this, consider that with respect to the part-whole relationship, a reductive analysis can always be applied—there is no activity that will not submit to body functional decomposition. In the emergent view on the other hand, activities that have no subgoals or form a temporally tightly coupled whole cannot be decomposed. Despite this, as we have seen in serious gaming and robot-assisted stepping, in such cases the reductive approach is applied anyway.
Importantly, stripping an activity, any activity, from its unfolding perceptual-motor dynamics in this way, means that from an emergent point of view the essence of the activity is not retained, but it is lost. The asymmetry lies in the fact that while the activity-oriented compartmentalization preferred by the emergent approach does not conflict with reductive thinking (at worse it may be criticized for not probing deep enough), the body-oriented compartmentalization does conflict with that of the emergent view. From an emergent perspective, when one creates an activity to target body functions more efficiently, one is not getting to the essence of the original activity, but one is introducing a new, and quite possibly, irrelevant one (e.g., robot-assisted stepping or serious gaming). For this reason, an awareness of the fundamental differences in points of view is of primary importance for rehabilitation practice. Considering the part-whole relationship in practice, when apparently applying the compartmentalization along both the reductive and the emergent dimensions, from the emergent perspective this equates to applying only the reductive approach. Doing so, in other words, creates a rehabilitation program that can only be made sense of from within the reductive perspective.
This asymmetry can perhaps be further illustrated by considering how to measure training effects in terms of body functions. In , the conceptualization of such a measurement is depicted. Here, for characterizing a change in performing an activity with a myoelectric prosthesis, myoelectric control is gauged through a computer task where the goal for the patient is to match a real-time representation of the myoelectric signal to a predetermined point on a screen (see e.g., Anderson & Bischof, Citation2014; Gordon & Ferris, Citation2004). The asymmetry lies in the fact that while the reductive approach will interpret the results of such a test as evidence for the underlying body function (e.g., myocontrol), the emergent approach will not. First, with respect to the performance of the test, according to the emergent view, performing the test should be considered as an activity in its own right. Thus, one should look at the behavioral goal of the test and the perceptual-motor dynamics involved to find out what activity was being performed. Crucially, this step reconceptualizes the test not at a level below the original ADL performance, but next to it, at the same level (). This begs us to consider the validity of such a testing performance.
FIGURE 2. (a). From a reductive perspective, the improvement in motor learning is measured by gauging body functions. Thus, the assumption is that one measures at the level of the underlying structure. This is indicated by the thought bubble. However, an emergent view (b) does not idealize the activity by neglecting the specific environmental coordination, goals and constraints involved in testing. The test for body functions is therefore not positioned below the level of activity, but next to it at the same level. (see text for details).
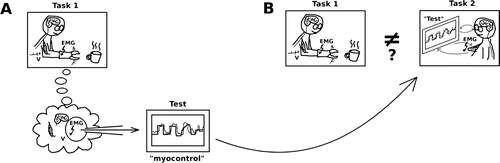
Second, with respect to the outcome-measure extracted from the test, considered as an activity the chosen outcome measure may now no longer be best suited to characterize the performance of the test (let alone the performance of ADL). For example, upon training a serious game to improve myoelectric prosthesis use, Anderson and Bischof (Citation2014) reported only the amount of co-contraction during a computer task. In this task the object was to match a myoelectrically controlled line to a predetermined point to characterize their improvement. But the outcome measure made no reference to the goal of the activity that the participant was performing. To do that, the amount of myosignal would for example have to be related to the accuracy of matching (i.e., the goal of the activity). In other words, any measure of an absolute body function reported from performing an activity, in an emergent view, lacks the theoretical importance it has to the reductive approach. Such measures can for example simply be considered a by-product, a consequence of rather than the cause of performing the activity (see e.g., Reed, Citation1988). The adopted perspective changes the framework that determines what counts as relevant and irrelevant to measure, target and improve.
Against this background, the importance of having the emergent view keep its independence relative to the reductive perspective becomes clear. The dominant reductive approach enthusiastically cuts up all tasks along hierarchical dimensions and makes research focus on body functional measures that admit only of a straightforward interpretation within the reductive framework. That is, the inclusion of body functional methods and the tendency to design training tools to target body functions makes it increasingly hard to escape the reductive framework and thus drowns out the development of other perspectives. Rehabilitation practices, methods and theories get increasingly forced to adhere to the reductive point of view at the expense of other, fundamentally different, ways of approaching the problems. What we need therefore, is to allow emergent perspectives to keep informing research.
Keeping an Open Mind: Start With a Focus on Transfer
The principle tool that the emergent view brings to rehabilitation research is that of studying transfer. That is, quantifying the effect the performance (or training) of one activity has on the performance of another activity. Traditionally, the reductive view assumes that a transfer effect shows that across activities a common underlying body function is shared. But as improvement of such a body function can be measured more accurately by laboratory testing (), testing for transfer is often omitted in favor of measuring this improvement in body function directly (this is especially true in the formative period of novel training programs, see Dobkins & Duncan, Citation2012; Goble, Cone, & Fling, Citation2014; Primack et al., Citation2012; Van Diest, Lamoth, Stegenga, Verkerke, & Postema, Citation2013). As we have seen previously, from the emergent view, such omission is both unwarranted and a fiction.
From an emergent point of view, any test (and training task for that matter) is an activity—thus any performance measure is related to another measure in terms of a transfer effect (see ). In this view two activities relate to each other, not by an assumed underlying structure, but only because both are performed by the same, learning and developing patient. A transfer effect thus does not admit of a similarity per se, but it reflects the amount of continuity in the perceptual-motor dynamics from activity to activity. Such a reinterpretation of transfer enables us to reinterpret any (body functional) test in emergent terms. Importantly moreover, it calls attention to the importance of focusing on transfer to ADL in the early stages of developing a training program. That is, rather than having transfer to ADL be the icing on the cake after all developments have been concretized, it suggests transfer to ADL tasks (rather than laboratory tests) should guide the development of training programs from the start.
CONCLUDING REMARKS
Starting with the practical question of how to cut up activities when training for their improvement we uncovered two fundamentally different understandings of the part-whole relation stemming from two fundamentally different approaches to motor learning. As we aimed to show, both approaches emphasize very different aspects of performance and design, and measure the effect of their training programs very differently. Our analysis of the background assumptions underlying the reductive and the emergent approach showed that they looked for parts of the whole along different dimensions. While the reductive approach looks along the hierarchical levels, the emergent approach looks at the level of the activity itself for identifiable subactions. We have been stressing that the emergent view therefore resists compartmentalization where reduction can always proceed. There are however many reasons why tasks cannot be practiced in one go even when an activity-oriented analysis suggests the task cannot be further decomposed, so it would be a major practical shortcoming if the emergent view has nothing to offer in such situations.
However, that the emergent approach has not been brought to bear on the problem does not mean it cannot cope with it. One possible way of offering activity-oriented practices in such circumstances is to practice activities in artificially simplified or augmented environments, such as in virtual reality or in serious gaming environments. Crucially however, the task that is recreated there should closely simulate those dynamical aspects that are found to be relevant to the activity in daily life. For example, recreating optic flow when walking around in a virtual scene or retaining and augmenting the perceptual-motor relation between the actions of a prosthetic hand and the relevant characteristics of the goal of grasping Citation(Van Dijk, Van der Sluis, & Bongers, in press). To emphasize however, for such approaches to offer an alternative to the many body functional initiatives, it needs to be understood in emergent terms. And the value of emergent training programs, as any training program, should be quantified in terms of transfer effects.
As our examples showed, many of the newest developments in rehabilitation research—especially those that are inspired by novel technological developments, such as rehabilitation robotics, virtual or augmented reality, or serious gaming—are still strongly drawn toward a reductive, body functional, approach. This might be due in part to the success of reductive approaches in the field of mechanical and computer engineering. As we have argued, a learning patient does not need to be conceptualized in the same terms. It is our hope that an awareness of the different points of views that are implicit and explicit in motor learning theories, rehabilitation programs and outcome measures, will help give the emergent approach the room to flourish. Thus we hope it can contribute to the plurality in views on motor learning in rehabilitation and help to inspire new ways of making creative and fruitful use of the full potential technological innovation offers.
ACKNOWLEDGMENTS
The authors thank Sil Kloppenburg and the members of the Groningen Research in Prosthestics (GRIP) group for their helpful discussions. The authors are also grateful to the two anonymous reviewers for their useful suggestions.
Notes
1. It should be noted that here we do not consider the therapeutic approaches of practitioners who base their methods on practical experience, and the way such approaches may affect the advancement of knowledge. It is for example interesting to consider that such practice might offer a way of exploring for novel and fruitful approaches that do not (yet) conform to any of the established perspectives.
2. Note that there are many varieties of reduction and emergence (e.g., Kim, Citation2003; Silberstein, Citation2002; Silberstein & McGeever, Citation1999). To make our point this most basic distinction will suffice.
REFERENCES
- Ahn, A. C., Tewari, M., Poon, C., & Philips, R. S. (2006). The limits of reductionism in medicine: Could systems biology offer an alternative? PLoS Medicine, 3(6), e208.
- Anderson, F., & Bischof, W. F. (2014). Augmented reality improves myoelectric prosthesis training. International Journal on Disability and Human Development, 13, 349–354.
- Armiger, R. S., & Vogelstein, R. J. (2008). Air-guitar hero: A real-time video game interface for training and evaluation of dexterous upper-extremity neuroprosthetic control algorithms. Proceedings of the IEEE Biomedical Circuits and Systems Conference (BioCAS 2008), 121–124.
- Bernstein, N. A. (1996). Dexterity and its development. In M. L. Latash & M. T. Turvey (Eds.), Dexterity and its Development (pp. 3–244). Mahwah, NJ: Erlbaum.
- Bingham, G., Coats, R., & Mon-Williams, M. (2007). Natural prehension in trials without haptic feedback but only when calibration is allowed. Neuropsychologia, 45, 288–294.
- Bruggeman, H., & Warren, W. H. (2010). The direction of walking—but not throwing or kicking—is adapted by optic flow. Psychological Science, 21, 1006–1013.
- Carr, J. H., & Shepherd, R. B. (1989). A motor learning model for stroke rehabilitation. Physiotherapy, 75, 372–380.
- Davids, K. W., Button, C., & Bennett, S. J. (2008). Dynamics of skill acquisition: A constraints-led approach. Champaign, IL: Human Kinetics.
- Dawson, M. R., Carey, J. P., & Fahimi, F. (2011). Myoelectric training systems. Expert Review of Medical Devices, 8, 581–589.
- Dobkin, B. H., & Duncan, P. W. (2012). Should body weight–supported treadmill training and robotic-assistive steppers for locomotor training trot back to the starting gate?. Neurorehabilitation and Neural Repair, 26, 308–317.
- Dosen, S., Markovic, M., Wille, N., Henkel, M., Koppe, M., Ninu, A., … & Farina, D. (2015). Building an internal model of a myoelectric prosthesis via closed-loop control for consistent and routine grasping. Experimental Brain Research, 233, 1855–1865.
- Duncan, P. W., Sullivan, K. J., Behrman, A. L., Azen, S. P., Wu, S. S., Nadeau, S. E., … & Hayden, S. K. (2011). Body-weight–supported treadmill rehabilitation after stroke. New England Journal of Medicine, 364, 2026–2036.
- Dupont, A. C., & Morin, E. L. (1994). A myoelectric control evaluation and trainer system. Rehabilitation Engineering, IEEE Transactions on, 2, 100–107.
- Edwards, W. (2010). Motor learning and control: From theory to practice. Belmont, CA: Wadsworth, Cengage Learning.
- Engel, G. L. (1977). The need for a new medical model: A challenge for biomedicine. Science, 196, 129–136.
- Gibson, J. J. (1979). The ecological approach to visual perception. Hillsdale, NJ: Erlbaum.
- Gil-Gómez, J. A., Lloréns, R., Alcañiz, M., & Colomer, C. (2011). Effectiveness of a Wii balance board-based system (eBaViR) for balance rehabilitation: A pilot randomized clinical trial in patients with acquired brain injury. Journal of Neuroengineering and Rehabilitation, 8, 30.
- Goble, D. J., Cone, B. L., & Fling, B. W. (2014). Using the Wii Fit as a tool for balance assessment and neurorehabilitation: The first half decade of “Wii-search”. Journal of Neuroengineering and Rehabilitation, 11, 12.
- Gordon, K. E., & Ferris, D. P. (2004). Proportional myoelectric control of a virtual object to investigate human efferent control. Experimental Brain Research, 159, 478–486.
- Graafland, M., Schraagen, J. M., & Schijven, M. P. (2012). Systematic review of serious games for medical education and surgical skills training. British Journal of Surgery, 99, 1322–1330.
- Haque, S., & Srinivasan, S. (2006). A meta-analysis of the training effectiveness of virtual reality surgical simulators. Information Technology in Biomedicine, IEEE Transactions on, 10, 51–58.
- Jacobs, D. M., & Michaels, C. F. (2007). Direct learning. Ecological psychology, 19, 321–349.
- Jeannerod, M. (1984). The timing of natural prehension movements. Journal of Motor Behavior, 16, 235–254.
- Keele, S. W. (1968). Movement control in skilled motor performance. Psychological Bulletin, 70, 387–403.
- Kelso, J. S. (1997). Dynamic patterns: The self-organization of brain and behavior. Cambridge, MA: MIT Press.
- Kim, J. (2003). Supervenience, emergence, realization, reduction. In M. J. Loux & D. W. Zimmerman (Eds.), The Oxford Handbook of Metaphysics (pp. 556–584). New York, NY: Oxford University Press.
- Krakauer, J. W. (2006). Motor learning: Its relevance to stroke recovery and neurorehabilitation. Current Opinion in Neurology, 19, 84–90.
- Kwakkel, G., Kollen, B. J., & Krebs, H. I. (2007). Effects of robot-assisted therapy on upper limb recovery after stroke: A systematic review. Neurorehabilitation and Neural Repair, 22, 111–121.
- Law, M., Cooper, B., Strong, S., Stewart, D., Rigby, P., & Letts, L. (1996). The person-environment-occupation model: A transactive approach to occupational performance. Canadian Journal of Occupational Therapy, 63, 9–23.
- Magill, R. A. (2003). Motor learning and control. New York, NY: McGraw Hill.
- Meijer, O. G., & Roth, K. (1988). Complex movement behaviour: The motor-action controversy. Amsterdam, the Netherlands: Elsevier.
- Naylor, J. C., & Briggs, G. E. (1963). Effects of task complexity and task organization on the relative efficiency of part and whole training methods. Journal of Experimental Psychology, 65, 217–224.
- Newell, K. M. (1986). Constraints on the development of coordination. In M. G. Wade & H. T. A. Whiting (Eds.), Motor development in children: Aspects of coordination and control (pp. 341–360). Dordrecht, the Netherlands: Martinus Nijhoff.
- Newell, K. M. (1996). Change in movement and skill: Learning, retention, and transfer. In M. L. Latash & M. T. Turvey (Eds.), Dexterity and its Development (pp. 393–430). Mahwah, NJ: Erlbaum.
- Newell, K. M., & Vaillancourt, D. E. (2001). Dimensional change in motor learning. Human Movement Science, 20, 695–715.
- Newell, K. M., Liu, Y. T., & Mayer-Kress, G. (2001). Time scales in motor learning and development. Psychological Review, 108, 57–82.
- Primack, B. A., Carroll, M. V., McNamara, M., Klem, M. L., King, B., Rich, M., … & Nayak, S. (2012). Role of video games in improving health-related outcomes: A systematic review. American Journal of Preventive Medicine, 42, 630–638.
- Reed, E. S. (1982). An outline of a theory of action systems. Journal of Motor Behavior, 14, 98–134.
- Reed, E. S. (1988). Applying the theory of action systems to the study of motor skills. In O. G. Meijer & K. Roth (Eds.), Complex movement behaviour: The motor-action controversy (pp. 45–86). Amsterdam, the Netherlands: Elsevier.
- Reed, E. S. (1996). Encountering the world: Toward an ecological psychology. New York, NY: Oxford University Press.
- Reed, E. S., Montgomery, M., Palmer, C., & Pittenger, J. (1995). Method for studying the invariant knowledge structure of action: Conceptual organization of an everyday action. The American Journal of Psychology, 108, 37–65.
- Rensink, M., Schuurmans, M., Lindeman, E., & Hafsteinsdottir, T. (2009). Task-oriented training in rehabilitation after stroke: Systematic review. Journal of Advanced Nursing, 65, 737–754.
- Rieser, J. J., Pick, H. L., Ashmead, D. H., & Garing, A. E. (1995). Calibration of human locomotion and models of perceptual-motor organization. Journal of Experimental Psychology: Human Perception and Performance, 21, 480–497.
- Romkema, S., Bongers, R. M., & Van Der Sluis, C. K. (2013). Intermanual transfer in training with an upper-limb myoelectric prosthesis simulator: A mechanistic, randomized, pretest-posttest study. Physical therapy, 93, 22–31.
- Schmidt, R. A. (1975). A schema theory of discrete motor skill learning. Psychological Review, 82, 225–260.
- Silberstein, M. (2002). Reduction, emergence and explanation. In P. Machamer & M. Silberstein (Eds.), The Blackwell Guide to the Philosophy of Science (pp. 80–107), Malden, MA: Blackwell.
- Silberstein, M., & McGeever, J. (1999). The search for ontological emergence. The Philosophical Quarterly, 49, 201–214.
- Skelton, D. A., Young, A., Greig, C. A., & Malbut, K. E. (1995). Effects of resistance training on strength, power, and selected functional abilities of women aged 75 and older. Journal of the American Geriatrics Society, 43, 1081–1087.
- Smurr, L. M., Gulick, K., Yancosek, K., & Ganz, O. (2008). Managing the upper extremity amputee: a protocol for success. Journal of Hand Therapy, 21, 160–175.
- Thielman, G. T., Dean, C. M., & Gentile, A. M. (2004). Rehabilitation of reaching after stroke: Task-related training versus progressive resistive exercise. Archives of Physical Medicine and Rehabilitation, 85, 1613–1618.
- Torkington, J., Smith, S. G. T., Rees, B. I., & Darzi, A. (2001). Skill transfer from virtual reality to a real laparoscopic task. Surgical Endoscopy, 15, 1076–1079.
- Van Dijk, L., & Bongers, R. M. (2014). The emergence of an action system: The organization of gaze in creating novel tools. Ecological Psychology, 26, 177–197.
- Van Dijk, L. Van der Sluis, C. K., & Bongers, R. M. (2015). Task-oriented gaming for transfer to prosthesis use. IEEE Transactions on Neural Systems and Rehabilitation Engineering. Published ahead of print.
- Van Diest, M., Lamoth, C. J., Stegenga, J., Verkerke, G. J., & Postema, K. (2013). Exergaming for balance training of elderly: State of the art and future developments. Journal of Neuroengering and Rehabilitation, 10, 101.
- Warren, W. H. (2006). The dynamics of perception and action. Psychological Review, 113, 358–389.
- Wevers, L., van de Port, I., Vermue, M., Mead, G., & Kwakkel, G. (2009). Effects of task-oriented circuit class training on walking competency after stroke a systematic review. Stroke, 40, 2450–2459.
- Winstein, C. J., & Wolf, S. L. (2008). Task-oriented training to promote upper extremity recovery. In J. Stein, R. L. Harvey, R. F. Macko, C. J. Winstein, & R. Zorowitz (Eds.), Stroke recovery and rehabilitation (pp. 267–290). New York, NY: Demos Medical.
- Withagen, R., & Michaels, C. F. (2002). The calibration of walking transfers to crawling: Are action systems calibrated?. Ecological Psychology, 14, 223–234.
- Wolpert, D. M. (1997). Computational approaches to motor control. Trends in Cognitive Sciences, 1, 209–216.
- World Health Organization. (2015). International classification of functioning. Geneva, Switzerland: Author.