ABSTRACT
The black rat (Rattus rattus) is an alien species that causes severe impact on island ecosystems, floras and faunas. The main aim of this study was to determine the plant and animal contributions to black rat diet in a pristine misty laurel forest area on La Palma (Canary Islands). Our working hypothesis was that this rat equally consumes plants and animals (fully omnivorous animal) wherever it is introduced, including pristine habitats. A total of 483 droppings collected from the terrain were first morphologically examined using a stereomicroscope, which showed high plant consumption (presence in 92.4% of droppings), followed by invertebrates (46.0%) and vertebrates (31.2%). DNA-based analyses revealed even higher proportions of plants (97%) and invertebrates (79%), while fine-scale sequence searches (DNA barcoding) in the GenBank (BLAST tool) provided a preliminary identification of 44 plants and 12 invertebrate taxa. To gain more in-depth insight into plant identification, we built up a local DNA reference collection (58 species), improving accuracy (30 species confirmed) compared to GenBank searches (25 species). Contingency analyses (chi-square and G-test) only showed significant differences in droppings between plant sequences and toxic plant presence. This study confirms that the black rat is here an omnivorous animal but with a strong plant diet component, including an intriguingly high number of toxic plants. Interestingly, despite rodents chewing on fruits and usually crushing seeds, 66 intact Rubus seeds (Rosaceae) were found in 15 droppings (3.1%). All these results suggest that black rats consume any plant types, including fruits and seeds that can be locally dispersed such as native brambles.
Introduction
The original distribution of the black rat or ship rat (Rattus rattus, L., 1758) is in Southeast Asia. Historical records indicate widespread introduction in Europe, particularly from the 8th century onwards due to increasing trade (Gómez and Verdú Citation2017). Maritime transport enabled this species to spread all over the Mediterranean Basin and from Europe to the Atlantic islands (MAGRAMA Citation2013). It has been described as an invasive rodent causing severe ecological impacts on islands (Harper and Bunbury Citation2015), particularly in the largest oceanic archipelagos worldwide: Hawaii (Shiels Citation2010), New Caledonia (Caut et al. Citation2008; Duron et al. Citation2019), French Polynesia (Zarzoso-Lacoste Citation2013), Galápagos (Riofrío-Lazo and Páez-Rosas Citation2015), Mozambique Channel Islands (Russell et al. Citation2011), Azores (Ceia et al. Citation2017) and the Canaries (Nogales et al. Citation2006).
The Canary Islands are a Macaronesian archipelago located in the Atlantic Ocean around 100 km off northwest Africa ()). Laurel forests (‘laurisilva’) are a unique habitat only occurring on the northern cloudy slopes of some of the islands in Azores, Madeira and Canaries. One of the best examples is found in ‘Bosque de Los Tilos’ (hereafter Los Tilos), the core area of La Palma Biosphere Reserve ()). This area is considered a well-conserved laurel forest, which is one of the most endangered habitats of Europe (UNESCO-MAB Biosphere Reserve Directory Citation2018).
Figure 1. (a) Map of the Canary Islands showing the location of La Palma Island Biosphere Reserve. (b) Current distribution of the laurel forest (grey) on the island and the study area in Bosque de Los Tilos. (c) Percentages of invertebrates and plants consumed, as detected after the barcode analysis. Each circle represents one location (including one or more separate microsites c. 200 m apart), located in the three plant formations (dominant laurel forest, Morella faya – Erica arborea woodland and fern patch).
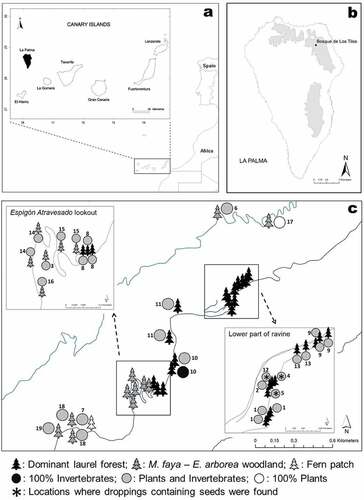
The black rat probably became established on the Canaries with European settlers in the 15th century but its trophic ecology is still poorly known, particularly in the laurel forest (Nogales et al. Citation2006). Some studies have shown a significant ecological impact of invasive black rats in the laurel forest, because of young shoot consumption and seed predation (Traveset et al. Citation2009) (Table S1). Rats can also affect animal communities through direct predation, raising conservation concerns for endangered Manx shearwater (Puffinus puffinus), two endemic Canary pigeons (Columba junoniae and C. bollii) (Hernández et al. Citation1999; Martín et al. Citation2000) and endemic terrestrial gastropods of the genera Hemicycla and Plutonia (Godoy Citation2001). Ceia et al. (Citation2017) quantified black rat diet on São Miguel (Azores), analysing items found in stomachs. However, to the best of our knowledge, no study has yet included quantification of its diet using DNA-based tools in this threatened unique habitat.
Previous studies on rodent diet analysed macro (Russell et al. Citation2011) and micro-remains (Shiels et al. Citation2013) found in gut or droppings, respectively. To gain more in-depth insight into species identification, some research analysed isotopes found in guts and droppings (Caut et al. Citation2008) or stomachs (Riofrío-Lazo and Páez-Rosas Citation2015) or searched for DNA barcode matches (Zarzoso-Lacoste et al. Citation2013, Citation2016; Khanam et al. Citation2016). Contributions based on macroscopic studies face serious difficulties in accurately identifying each food component (Zarzoso-Lacoste et al. Citation2013, Citation2016). Therefore, to circumvent these problems derived from using only that approach, reliable results for black rat diet have been obtained using DNA sequence analyses of droppings and stomach contents from different habitat types (Zarzoso-Lacoste Citation2013; Khanam et al. Citation2016). Indeed, to date, only Zarzoso-Lacoste (Citation2013) performed both morphological (macro- and micro-histological) and DNA sequence analyses of the black rat diet on oceanic islands.
The main goal of this study was to investigate the diet of the black rat in a pristine laurel forest on La Palma, by combining macroscopic and DNA sequence (barcode) methods. Our working hypothesis was that this rat could equally or indifferently consume any available food resource. We established three specific objectives: 1) to quantify black rat diet in the Canary Islands; 2) to assess whether the spatial component contributes differently to its diet, and 3) to describe any ecological role of black rat as seed predator or potential seed disperser.
Material and methods
Study area
Data were collected in ‘Los Tilos’ (28° 79ʹN, 17° 80ʹW) (), one of the densest and most humid laurel forests on La Palma. It has a relatively high annual mean precipitation (2000 mm). Dominant tree species in Los Tilos are: Ocotea foetens, Laurus nobilis (inc. L. novocanariensis, Rodríguez-Sánchez et al. Citation2009), Persea indica, Apollonias barbujana (Lauraceae), Ilex canariensis (Aquifoliaceae), Picconia excelsa (Oleaceae), Viburnum rigidum, Sambucus palmensis (Adoxaceae), Erica arborea (Ericaceae) and Morella faya (Myricaceae) (del Arco Aguilar et al. Citation2006). Sampling was performed along a forestry track that crosses the laurel forest from the ravine-bed (490 m a.s.l.) in the lower areas, where humidity is high even in summer (90–100%) and leads to a ridge (750 m a.s.l. at ‘Espigón Atravesado’ lookout point). There are human modifications that alter the ecosystem characteristics intensively (tunnels for the passage of people and vehicles, water extraction galleries, small buildings,) or slightly (plant nursery, water pipes), mostly at the beginning of the track.
Sampling design
A total of 483 recent rat droppings were collected along a 2 km-long sample transect in Los Tilos (Espigón Atravesado track), when fruits and seeds were available (June 3 to 27 July 2017; 44 days of fieldwork). Each microsite (e.g. tree hollows, walls, stones, water pipelines and channels) with visible rat droppings was marked and cleaned every day to collect new droppings. Accordingly, old droppings were not considered in this study to avoid environmental contamination and pseudoreplication. All microsites were described and georeferenced with the help of IDE Canarias visor 4.5 (Citation2018). Locations (n = 19) were designated along the track to include groups of microsites less than 200 m apart, on account of the maximum distance covered by the black rat in 1–2 years (Shiels Citation2010; Global Invasive Species Database Citation2018). Among these 19 independent locations, 63% (n = 12) were rat latrines, rat nests or microsites, where almost every day we found droppings with slightly different shapes and sizes. This allowed us to study droppings with potentially distinct diet components. Based on vegetation types, three different habitats were distinguished: (i) the dominant laurel forest (a closed -canopy featuring mainly O. foetens, L. nobilis, P. indica, A. barbujana), which forms a dense cloud forest mainly along the ravine; (ii) smaller trees and bushes (M. faya and E. arborea) forming a higher altitude, more open, woodland mostly on the ridge; and (iii) fern patches (Woodwardia radicans, Adiantum reniforme, Pteris incompleta, Polystichum setiferum, Diplazium caudatum) located on the shady, humid slopes. Additionally, we collected 58 plant species occurring in the laurel forest (82% of the species in Los Tilos) (Table S2) and built up our barcode reference collection based on DNA sequences of all these plants (see Zarzoso-Lacoste et al. Citation2013; Khanam et al. Citation2016).
Diet analysis
Each sample was preserved in silica gel for drying. Droppings with an abnormal shape or length (deviation of 25% from mean values (mean length = 11.8 mm)) were discarded. Confusion with droppings of the brown rat (Rattus norvegicus, Berkenhout Citation1769) is unlikely because in the Canaries this other rodent is only found in human settlements and crops (Nogales et al. Citation2006; Traveset et al. Citation2009; Fernández-Palacios et al. Citation2017). However, the closest urbanised village (Los Sauces) is 4 km from the study area, and we only observed the presence of R. rattus in the laurel forest during fieldwork. Moreover, it has never been recorded in the study area or any laurel forest of the Canary Islands (see Nogales et al. Citation2006). Anyway, to confirm that all droppings collected belonged to Rattus rattus, 15 droppings selected from the subsamples were sequenced. These droppings were collected at representative locations of the different laurel forest microhabitats (Table S3). We paid no attention to whether each dropping belonged to a particular individual because our goal was to determine the rat’s diet in the laurel forest. In any case, the territorial behaviour of black rats leads us to interpret that droppings at each location belonged to a single individual or familiar group.
Our consumed species identification strategy included: (i) macroscopic analysis of dropping content, (ii) DNA sequence analysis of PCR amplicon fragments by checking a correct amplification on agarose gels (hereafter ‘amplicon analysis’), and (iii) taxonomic identification of species using DNA sequences analysis (Zarzoso-Lacoste et al. Citation2013) of droppings (hereafter ‘DNA sequence barcoding’) and the BLAST tool.
The macroscopic (hereafter macrohistological, i.e. tissue remains and seeds) analysis focussed on the content of each sample when spread out on a Petri dish under a magnifier (x10). Diet items were identified according to the animal (‘invertebrate’ or ‘vertebrate’) or ‘plant’ origin and in a few cases to the species level. Invertebrates were identified from exoskeleton, wing, and shell fragments; and vertebrates from bones, feathers and hairs compared with our reference collections, and plants from seeds, leaves and stem fragments, by comparison with our corresponding local collections. We calculated the frequency of macrohistological occurrence with respect to the number of droppings (FOmacrohistological) for the three types of organism (plant, invertebrate or vertebrate), taking each dropping as a single unit without considering whether it contained more than one item of the same origin.
The DNA-based analysis (amplicon analysis and DNA sequence barcoding) included a subsample of 100 samples from the 483 droppings chosen to represent the three vegetation types, track site distances and dates. We expected that this DNA-based approach would show the most diverse elements from each sample by improving prey detection and identification. We focused the DNA-based analysis on plants, invertebrates and birds because they are potentially consumed by black rats on the Canaries (Nogales et al. Citation2006). Other groups of vertebrates were not considered in this analysis because they avoid humid forest (especially mice and lizards) or inhabit towns and other human environments (e.g. brown rat) (Fernández-Palacios et al. Citation2017). The DNeasy mericon Food Kit (Qiagen Inc., Hilden, Germany) (see Zarzoso-Lacoste et al. Citation2013; Khanam et al. Citation2016) was used to extract DNA from droppings, and DNeasy Plant Mini Kit (Qiagen Inc., Hilden, Germany) for seed embryos and our reference collection. As positive controls for bird presence, we used DNeasy Blood and Tissue Kit (Qiagen Inc., Hilden, Germany) with chicken tissues (meat and marrowbone).
PCR protocols were optimised in a final volume of 25 µl, containing 14.2 µl of water, 2.5 µl of buffer, 1.4 µl of MgCl2 50 mM, 1.6 µl of dNTPs 2.5 mM, 0.5 µl of each primer 10 µM, 0.3 µl of Taq polymerase 5 U/µl, 1 µl of BSA 1 mg/ml, 1 µl of DMSO and 2 µl of the DNA sample extraction. PCR temperature conditions followed those of Zarzoso-Lacoste et al. (Citation2013) and Khanam et al. (Citation2016), except for the annealing temperature for invertebrate PCRs (51°C). For our reference plant collection, we used the primer pair rbcL h1aF and rbcL h2aR and an annealing temperature of 52°C (Table S4). In addition, we used the primer pair M15997 (5ʹ-TCCCCACCATCAGCACCCAAAGC-3ʹ) and H16401 (5ʹ-TGGGCGGGTTGTTGGTTTCACGG-3ʹ) and an annealing temperature at 59°C to confirm that the collected droppings belong to Rattus rattus (Shirako et al. Citation2015).
Contamination by small amounts of air-borne or ground-borne cells or pollen may not have been amplified, due to the low quantities of DNA in comparison with the dominant plant/animal contents of each dropping. Two different DNA sequence strategies of this subsample were performed: PCR amplicon analysis (presence/absence of DNA bands observed on verification gels); and DNA sequence barcoding (nucleotide match of over 95% of plastid DNA regions).
The successfully amplified PCR products allowed us to calculate the frequency of DNA amplicon occurrence and DNA sequences (FODNA amplicon and FO DNA sequences) for the three types of organisms considered in this study (plants, invertebrates and birds), which was needed to test our working hypothesis. Also, this approach facilitated comparing our results with those from previous dietary studies (Zarzoso-Lacoste et al. Citation2013; Khanam et al. Citation2016). To examine the reproducibility of the PCR amplicons, instead of cloning these amplicons to individualise them, we repeated all PCR reactions once for each sample. Results of each PCR were added to the final results of each sample.
In addition, PCR products were used for direct sequencing (Sanger Technology) at the Macrogen facility (Madrid, Spain). Nucleotide sequences were edited with Geneious v.7.1.5. Sequence searches were addressed to identifying species in droppings more accurately, using: (i) the GenBank Basic Local Alignment Search Tool (BLAST), and (ii) our plant reference collection (Table S2). Taxa were identified at species level applying both methods, following the strategy of Zarzoso-Lacoste et al. (Citation2013).
Contingency analyses for plant sequences
Four independent variables were tested with the statistical software R 3.3.3 (R Core Team Citation2018). We removed data with only one observation per level, to deal with the low number of observations. We employed contingency tables (chi-square test for expected frequencies >5 (Agresti Citation2018) and G-test for expected frequencies <5 included in MASS package (Venables and Ripley Citation2002)) to evaluate the distribution of plant DNA sequences (thereafter ‘Plant sequence’). In particular, the barcodes obtained for 70 individuals or groups (identified species) of ‘Plant sequence’ (dependent variable with 70 levels) positives found in 92 samples () were analysed, contrasting with the following four independent variables. (i) ‘Plant sequence’ and ‘Locations’ (19 levels corresponding to groups of microsites less than 200 m apart, considering that foraging territories of black rats are around 4.0 ha (Shiels Citation2010; Global Invasive Species Database Citation2018), which represent physiognomic vegetation differences along the forestry track (see )) and thus to identify the distribution of plants eaten; (ii) ‘Plant sequence’ and ‘Habitat type’ (three levels corresponding to dominant laurel forest, M. faya – E. arborea woodland or fern patches) to assess vegetation use; (iii) ‘Plant sequence’ and ‘Human modification’ (three levels corresponding to low, medium or high) to quantify whether anthropogenic disturbance (tunnels, buildings or pipelines) has any influence; and (iv) ‘Plant sequence’ and ‘Toxicity’ (two levels corresponding to yes/no) to evaluate the importance of toxic plants (plants that when ingested in sufficient quantity can be harmful or fatal to animals) in black rat diet (Delgado Citation1998).
Table 1. Frequency of plants found in the 97 (out of 100) droppings DNA-based analysed (taxonomic identification of species using DNA sequences) using the GenBank tool BLAST and our DNA reference collection (rbcL sequences)
Results
Macroscopic analysis
The majority of the 483 rat droppings (93.8%) were collected along the Espigón Atravesado track. Plants were the main component of the diet (FOmacrohistological = 92.4%), followed by invertebrates (46.1%) and vertebrates (31.2%) ( and Table S1). Although gnawed vertebrate bones were found in a third of the samples, we could not confirm the identity of any vertebrate species, including birds, due to bone deterioration. Additionally, 15 (FOmacrohistological = 3.1%) of the rat droppings contained a total of 66 seeds of Rubus spp. (see ), and six (FOmacrohistological = 1.2%) of them included small intact invertebrates (all Armadillidium vulgare). Some intact seeds were found, although difficult to identify by morphological features; that is why we needed to sequence their embryos (see below).
Table 2. Macro- and microhistological analyses of black rat diet in La Palma biosphere reserve, compared with results for other archipelagos around the world
Amplicon detection and identification
The subsample of 100 rat droppings (20.7%), representing most of the situations (habitat type, human modification, sampling date), was analysed both macrohistologically and DNA-based to complement and narrow down species candidates. The DNA sequence analysis of Rattus sequences confirmed that the droppings (n = 15) belong to R. rattus, because our sequences only match with R. rattus sequences in GenBank, with an E-value of 0 and Per. Ident (The extent to which two sequences have the same nucleotides at the same positions in an alignment >98%). Macrohistological analysis of this subsample yielded FOmacrohistological = 91% for plants and FOmacrohistological = 62% for invertebrates. In comparison, amplicon analysis of PCR fragments disclosed successful amplification of plant and invertebrate DNA items (FODNA amplicon = 97% and FODNA amplicon = 79%, respectively). In contrast, no bird DNA amplicons were detected. In the same way, three droppings (FODNA amplicon = 3%) did not show any plant or invertebrate remains (PCR products unsuccessfully amplified), while 76 droppings (FODNA amplicon = 76%) contained both plants and invertebrates. Spatial distribution and the overall results for each microsite are shown in ).
DNA barcode sequence analysis identified 44 plants and 12 invertebrates from 97 rat droppings that showed positive results, 92 for plants (Table S5) and 12 for invertebrates (Table S6). GenBank searches helped identify 25 plants at the species level, while 30 others were matched with our reference collection, and some shared by both searches (). DNA sequences (100% sequence matches) from 33 of the 66 intact seeds found in rat droppings were thus identified within the genus Rubus, pointing to R. bollei and R. palmensis as species candidates. A total of 39 seeds (59%) were found in rat droppings collected on 27th July, and 27 seeds (41%) between 8th June and 13th July. Some of the plant species, such as Heberdenia excelsa, Pleiomeris canariensis, Rhamnus glandulosa, Sambucus palmensis, Sideroxylon canariense, Visnea mocanera, Chamaecytisus proliferus, Teline stenopetala and Castanea sativa are very scarce in Los Tilos and not found in droppings.
Contingency analyses for plant sequences
The number of observations per level of each variable is shown in Table S5. No significant differences were found between species identifications and three of the four independent variables: ‘Habitat type’, ‘Human modification’ and ‘Location’. However, we recorded significant differences in the case of ‘Toxicity’ (χ2 = 92, df = 68, p = 0.024), suggesting that black rats consume toxic plants more than expected randomly, such as Hedera canariensis, P. indica, V. rigidum, Polygonum sp., Crambe santosii and Orobanche minor. Marginally significant differences were also observed between species identifications and the variable ‘Location’, suggesting that black rats eat particular plant species along Espigón Atravesado track (χ2 = 162.93, df = 136, p = 0.057). However, we did not obtain significant differences with either ‘Habitat type’ (p > 0.1), since the presence of the fern Diplazium caudatum, found in 14% of droppings along almost the whole track, probably homogenised diet results in the three habitats. Additionally, ‘Human modification’ such as tunnels, buildings or pipelines did not affect black rat diet (p > 0.1).
Discussion
During the last decade, new studies have provided a more detailed picture of black rat diet in some of the archipelagos around the world. Initially, stomach and faecal contents were macrohistologically analysed, which revealed a generalist diet with a strong plant component in Hawaii (Shiels et al. Citation2013), Galápagos (Riofrío-Lazo and Páez-Rosas Citation2015), New Caledonia (Caut et al. Citation2008; Duron et al. Citation2019), French Polynesia (Zarzoso-Lacoste Citation2013), Azores (Ceia et al. Citation2017) and the Mozambique Channel Islands (Russell et al. Citation2011) (). Barcode analyses are increasingly used because they provide essential data to interpret the ecological impact of rats on islands (Zarzoso-Lacoste et al. Citation2016, Citation2019). In particular, the scarcity of information about the ecological impact of sizeable black rat populations on some laurel forest species of the Canary Islands (Hernández et al. Citation1999; Godoy Citation2001; Nogales et al. Citation2006; Traveset et al. Citation2009) can be remedied by employing further DNA-based (particularly metabarcoding) analyses, that sequence multiple small but highly informative amplicons (Pompanon et al. Citation2012; de Barba et al. Citation2014). The use of this accurate method can give more insight into the diet of introduced vertebrates to understand and more successfully manage their impact on oceanic islands.
Black rat diet on La Palma in the context of other archipelagos
Our study provides further evidence of an omnivorous behaviour pattern of the black rat with a strong plant component, in which most rat droppings (76%) contained some animal prey among predominantly plant species. From the total of 23 plant species already reported as usually consumed by rats (Salvande et al. Citation2001, Citation2003; Traveset et al. Citation2009; and Francisco Sánchez, José Javier Fernández, Narciso Lorenzo and Rafael P. Rodríguez, personal observations and communications), 13 were identified in our DNA sequence barcoding analysis. Although we did not retrieve 10 of these species in our subsample of 100 droppings, we completed the list with 17 new species (Table S1), raising to 44 the number of plant species that can be considered part of black rat diet in the Canaries. Some of these species are very scarce in the study area or toxic for animals.
In the case of animals, the consumption of Hemicycla spp., Armadillidium vulgare and Canariella giustii were confirmed. However, we failed to find evidence of Tenebrionidae and the two endemic pigeon species (Table S1) reported in previous studies (Hernández et al. Citation1999; Martín et al. Citation2000). Indeed, plants are consistently the most important food sources in all the Macaronesian archipelagos except the Azores (Ceia et al. Citation2017), closely followed by invertebrates (). In the present study, a total of 44 plants and five animal species have been recorded at the species level from four different data sources: literature, direct observation, macrohistological characterisation of tissue remains and barcode analysis of rat droppings (Tables S1). Indeed, predominant plant consumption is observed when comparing our results with previous data from published inventories for black rats: 62% of plant species (44 of 71 plants cited in Santos Citation1983), 12% of invertebrates (6 of 51 invertebrates gathered in Ortega Citation2005; Arndt Citation2006), and 18% of vertebrates (2 of 11 vertebrates, Fernández-Palacios et al. Citation2017).
Prey species were more accurately identified from amplicon sequencing, which shows a slightly higher proportion of plants (97%) than those detected macrohistologically (91%). Differences were higher in samples containing invertebrates (79% and 62%, respectively), and plants and invertebrates (76% and 59%, respectively). These differences (between plants and invertebrates FOmacrohistological) could be due to more advanced degradation and digestion of invertebrate soft parts in droppings, which are not easy to identify using a stereomicroscope (Jordan Citation2005; Pisanu et al. Citation2011; Zarzoso-Lacoste Citation2013). The predominance of plant items in droppings could be biased because of gathering samples with similar size and shape products of plant digestion processes. To circumvent this problem, we collected near rat latrines and nests, including a broad morphology range of droppings. Interestingly, the DNA barcode sequence analysis from La Palma rendered similar percentages to those found in French Polynesia for plants (100%) and invertebrates (83.3%) (Zarzoso-Lacoste et al. Citation2019). The latter study disclosed birds (1.3%) as part of the black rat diet, whereas we did not find any molecular evidence for birds. Since vertebrate remains frequently appear in the macrohistological analysis of droppings, lack of bird evidence from our samples could point to either no consumption or failure to find DNA amplicons evidence of it. This is particularly so because PCR amplification often fails when target DNA is scarce (Zarzoso-Lacoste et al. Citation2016). Furthermore, DNA contained in carrion is scarcer than in fresh meat (Foltan et al. Citation2005) and finding gnawed bones in droppings suggests either scavenging behaviour or direct predation of chicks and adults.
In sum, macrohistological and DNA-based analyses showed that the black rat eats a great variety of plants, including rare species, but less variety of invertebrates and vertebrates. This behaviour contrasts with our working hypothesis about a balanced omnivorous diet.
Consumption of toxic plants
A significant relationship was found between plant DNA sequences and toxicity, and nearness to certain locations. The high frequency of toxic plants consumed by the black rat is intriguing. Digestive tolerance of Persea indica leaves, stem and fruits is unexpected for most vertebrates, given their rich content of ryanoid diterpenes (Darias et al. Citation1986; Fraga et al. Citation2001; Delgado García Citation2002). The black rat also consumes numerous plants with toxic compounds: H. canariensis (hederagenin, toxic acids, arsenic), Polygonum sp. (nitrates), C. santosii (toxic glycosides), O. minor (toxic glycosides, tannins) (see Delgado Citation1998). This variety of compounds suggests that it does not show strong avoidance for these particular toxic chemicals, which is in accordance with a super-generalist diet based on plants. Further studies should be conducted to investigate whether black rats show preference or avoid each toxic plant when rats can choose between toxic and non-toxic plants.
The black rat ate different plant species at diverse locations, which may simply indicate differences because forest vegetation physiognomy along the track. Although this diversity may also reflect the varying habits of individual rats, Rattus rattus typically exhibits a similar omnivorous behavioural prey pattern. Given that each individual can forage territories of around 4 ha (Shiels Citation2010; Global Invasive Species Database Citation2018), we consider that the black rat benefits from a heterogeneous altitudinally and humidity-stratified vegetation, including numerous toxic plants of the Canarian laurel forest.
The black rat as a seed disperser
Black rats destroy most seeds and fruits because of the way they thoroughly gnaw and chew them (Delgado García Citation2002; Gómez and Fernández Citation2003; Salvande et al. Citation2003). However, small hard seeds can survive the chewing and digestion process and pass through intact (Williams et al. Citation2000; Shiels Citation2011; Shiels and Drake Citation2011; Riofrío-Lazo and Páez-Rosas Citation2015; Duron et al. Citation2017). The finding of 66 intact seeds (see ) exclusively of native bramble plants (Rubus spp.) in 15 rat droppings (at three locations) indicates that the black rat probably actively disperses their seeds. In Hawaii, fruits of the alien species Rubus rosifolius consumed by captured black rats showed 100% seed survival after food mastication and gut passage (Shiels Citation2010). In any case, seed dispersal services provided by the black rat on oceanic islands depend upon its dispersal effectiveness, which includes the number of seeds dispersed, seedling emergence and seedling survival (González-Castro et al. Citation2015). Unfortunately, there are no data on post-dispersal stages in the Canaries. The black rat consumes not only endemic (endangered) species of the Hawaiian flora (Diospyros spp., Cyanea superba) but also eats and disperses alien species (Clidemia spp., Miconia spp., Buddleia spp., Ficus spp.) (Shiels Citation2011). In New Caledonia, they disperse native seeds of Ficus racemigera and Freycinetia sulcata, but less efficiently than native dispersers (Duron et al. Citation2017). On offshore islands of southeast France, rats disperse seeds of the invasive Carpobrotus spp. (Bourgeois et al. Citation2005). We only found seeds of the native Rubus (R. bollei or R. palmensis) in rat droppings from the laurel forest, despite some alien species with small seeds being available nearby.
Concluding remarks
In conclusion, the diet of the black rat is omnivorous, including a wide variety of species of animals and plants, the latter being predominant in frequency of occurrence. Barcode analysis of droppings significantly helped to provide a more accurate composition of rat diet than macrohistological analyses, which leads us to recommend the use of DNA-based analyses as an essential tool on a routine basis. Future research is needed to improve the barcode analysis employed, incorporating metabarcoding techniques (Pompanon et al. Citation2012; de Barba et al. Citation2014) that use multiple-sequence primers for greater accuracy in the search for additional organisms (Zarzoso-Lacoste et al. Citation2013). Rattus rattus consumes a high number of toxic laurel forest plant species, which is related to location rather than habitat distribution (dominant laurel forest, M. faya – E. arborea woodland, fern patches). The presence of intact Rubus seeds in rat droppings indicates that at least some small seeds pass through their gut, and thus rats have a potential seed dispersal role although limited by their gnawing habits.
Nevertheless, the ecological function of dispersal already described for some other islands needs to be tested with further dispersal effectiveness experiments (viability and germination tests). The fact that the results show a considerable quantity of toxic plants in the diet of black rat leads us to enquire into a new issue: Do rats eat any plants irrespective of plant toxicity or do they intentionally search for certain toxic plants? This question, faced with all the drug experimentation historically performed on laboratory rodents, encourages new studies into this fascinating finding. Lastly, although we have mainly focussed our rat diet research on the dry season (June 3 to July 27), more detailed yearly studies contrasting spatial and seasonal variations in food availability may shed further light on its temporal impact on this important endangered endemic forest reserve, which so far at this site survives relatively undisturbed.
Geolocation information
Bosque de Los Tilos (28° 79ʹN, 17° 80ʹW) is located in the north-east of La Palma (Canary Islands).
TNAH-OA_20-094-File011.docx
Download MS Word (9.2 KB)TNAH-OA_20-094-File010.docx
Download MS Word (16.9 KB)TNAH-OA_20-094-File009.docx
Download MS Word (9.7 KB)TNAH-OA_20-094-File008.docx
Download MS Word (9.3 KB)TNAH-OA_20-094-File006.docx
Download MS Word (12.3 KB)Acknowledgements
We are very grateful to Rafael Pedro Rodríguez, Francisco Sánchez, José Javier Fernández and Narciso Lorenzo, from the ‘Servicio de Medio Ambiente del Cabildo Insular de La Palma’, who helped us during fieldwork. Our thanks to Olga Popova and Emilio Cano, laboratory technicians at the Royal Botanical Garden (Spanish Research Council, CSIC) for helping us to perform the extraction and PCR protocols. All the support received by the members of the research group was essential, especially that from Mario Fernández-Mazuecos. We thank Diane Zarzoso-Lacoste for the highly useful advice provided. The manuscript was edited by Guido Jones, currently funded by the Cabildo de Tenerife, under the TFinnova Programme supported by MEDI and FDCAN funds. Finally, we thank the Cabildo Insular de La Palma for authorisation (A/EST-010/2017) and logistical support.
Disclosure statement
No potential conflict of interest was reported by the author(s).
Supplementary material
Supplemental data for this article can be accessed here.
Additional information
Funding
References
- Agresti A. 2018. An introduction to categorical data analysis. New York: John Wiley & Sons.
- Arndt E. 2006. Niche occupation by invasive ground-dwelling predator species in Canarian laurel forests. Biol Invasions. 8(4):893–902. doi:10.1007/s10530-005-0423-x.
- Berkenhout J. 1769. Outlines of the natural history of Great Britain and Ireland: containing a systematic arrangement and concise description of all the animals, vegetables and fossiles which have hitherto been discovered in these kingdoms. London: Printed for P. Elmsly (Successor to Mr. Vaillant).
- Bourgeois K, Suehs CM, Vidal E, Médail F. 2005. Invasional meltdown potential: facilitation between introduced plants and mammals on French Mediterranean islands. Ecoscience. 12(2):248–256. doi:10.2980/i1195-6860-12-2-248.1.
- Caut S, Angulo E, Courchamp F. 2008. Dietary shift of an invasive predator: rats, seabirds and sea turtles. J Appl Ecol. 45(2):428–437. doi:10.1111/j.1365-2664.2007.01438.x.
- Ceia RS, Sanches S, Ramos JA. 2017. Foraging ecology of introduced rodents in the threatened Macaronesian laurel forest of São Miguel island (Azores) and contiguous exotic forests. Mammal Study. 42(3):141–151. doi:10.3106/041.042.0304.
- Darias V, Bravo L, Barquín E, Herrera DM, Fraile C. 1986. Contribution to the ethnopharmacological study of the Canary Islands. J Ethnopharmacol 15(2):169–193. doi:10.1016/0378-8741(86)90154-6.
- de Barba M, Miquel C, Boyer F, Mercier C, Rioux D, Coissac E, Taberlet P. 2014. DNA metabarcoding multiplexing and validation of data accuracy for diet assessment: application to omnivorous diet. Mol Ecol. 14(2):306–323. doi:10.1111/1755-0998.12188.
- del Arco Aguilar M, Wildpret W, Pérez de Paz PL, Rodríguez DO, Acebes JR, García GA, Martín VE, Reyes Betancourt JA, Salas M, Bermejo JA, et al. 2006. Mapa de Vegetación de Canarias. Santa Cruz de Tenerife: Grafcan.
- Delgado García JD. 2002. Interaction between introduced rats and a frugivore bird-plant system in a relict island forest. J Nat Hist. 36(10):1247–1258. doi:10.1080/00222930110039594.
- Delgado LMD 1998. Contribución al estudio de la flora tóxica de las Islas Canarias [PhD thesis]. Santa Cruz de Tenerife (Spain): University of La Laguna.
- Duron Q, Bourguet E, Thibault M, Scussel S, Gouyet R, Méheut M, Vidal E. 2019. Sympatric invasive rats show different diets in a tropical rainforest of an island biodiversity hotspot. Pacific Sci. 73:199–214. doi:10.1007/s10530-016-1284-1
- Duron Q, García-Iriarte O, Brescia F, Vidal E. 2017. Comparative effects of native frugivores and introduced rodents on seed germination in New-Caledonian rainforest plants. Biol Invasions. 19(1):351–363. doi:10.1007/s10530-016-1284-1.
- Fernández-Palacios JM, Arévalo JR, Balguerías E, Barone R, de Nascimento L, Delgado JD, Elías A, Fernández-Lugo R, Méndez S, Naranjo J, et al. 2017. La Laurisilva: Canarias, Madeira y Azores. Spain: Macaronesia.
- Foltan P, Sheppard S, Konvicka M, Symondson WO. 2005. The significance of facultative scavenging in generalist predator nutrition: detecting decayed prey in the guts of predators using PCR. Mol Ecol. 14(13):4147–4158. doi:10.1111/j.1365-294X.2005.02732.x.
- Fraga BM, Terrero D, Gutiérrez C, González-Coloma A. 2001. Minor diterpenes from Persea indica: their antifeedant activity. Phytochemistry. 56(4):315–320. doi:10.1016/S0031-9422(00)00376-9.
- Global Invasive Species Database. 2018. Species profile: Rattus rattus. [accessed 2018 Jan 23]. http://www.iucngisd.org/gisd/speciesname/Rattus+rattus.
- Godoy D 2001. Estudio sobre la dinámica de la depredación de semillas en el monteverde de Anaga (Tenerife) [PhD thesis]. Tenerife (Spain): University of La Laguna.
- Gómez JM, Verdú M. 2017. Network theory may explain the vulnerability of medieval human settlements to the Black Death pandemic. Sci Rep-UK. 7:1–7. doi:10.1038/srep43467.
- Gómez LA, Fernández AB. 2003. Defoliación y consumo de frutos realizados por las ratas (Rattus spp.) en árboles y arbustos de la laurisilva del Parque Nacional de Garajonay (La Gomera, Islas Canarias, España). In: Rodríguez-Luengo JL, editor. Control de Vertebrados Invasores en Islas de España y Portugal. Consejería de Medio Ambiente y Ordenación Territorial del Gobierno de Canarias (España); p. 117–124.
- González-Castro A, Calviño-Cancela M, Nogales M. 2015. Comparing seed dispersal effectiveness by frugivores at the community level. Ecology. 96(3):808–818. doi:10.1890/14-0655.1.
- Harper GA, Bunbury N. 2015. Invasive rats on tropical islands: their population biology and impacts on native species. Global Ecol Conserv. 3:607–627. doi:10.1016/j.gecco.2015.02.010.
- Hernández MA, Martín A, Nogales M. 1999. Breeding success and predation on artificial nests of the endemic pigeons Bolle’s laurel pigeon Columbia bollii and white-tailed laurel pigeon Columba junoniae in the laurel forest of Tenerife (Canary Islands). Ibis. 141:52–59.
- IDE Canarias visor 4.5. 2018. [accessed 2018 Oct 22]. https://visor.grafcan.es/visorweb/.
- Jordan MJR. 2005. Dietary analysis for mammals and birds: a review of field techniques and animal-management applications. Int Zoo Yb. 39(1):108–116. doi:10.1111/j.1748-1090.2005.tb00010.x.
- Khanam S, Howitt R, Mushtaq M, Russell JC. 2016. Diet analysis of small mammal pests: a comparison of molecular and microhistological methods. Integr Zool. 11(2):98–110. doi:10.1111/1749-4877.12172.
- MAGRAMA. 2013. Catálogo español de especies exóticas invasoras. Spain: Ministerio de Agricultura, alimentación y medio ambiente. [accessed 2018 Oct 22]. https://www.miteco.gob.es/es/biodiversidad/temas/conservacion-deespecies/rattus_rattus_2013_tcm30-69972.pdf.
- Martín A, Hernández MA, Lorenzo JA, Nogales M, González C. 2000. Las palomas endémicas de Canarias. Spain: Consejería de Política Territorial y Medio Ambiente del Gobierno de Canarias and Seo/Birdlife.
- Nogales M, Rodríguez-Luengo JL, Marrero P. 2006. Ecological effects and distribution of invasive non-native mammals on the Canary Islands. Mammal Rev. 36(1):49–65. doi:10.1111/j.1365-2907.2006.00077.x.
- Ortega G. 2005. Tipos de artrópodos terrestres en la colección entomológica del Museo de Ciencias Naturales de Tenerife (islas Canarias). Vieraea. 33:201–240.
- Pisanu B, Caut S, Gutjahr S, Vernon P, Chapuis J-L. 2011. Introduced black rats Rattus rattus on Ile de la Possession (Iles Crozet, Subantarctic): diet and trophic position in food webs. Polar Biol. 34(2):169–180. doi:10.1007/s00300-010-0867-z.
- Pompanon F, Deagle BE, Symondson WO, Brown DS, Jarman SN, Taberlet P. 2012. Who is eating what: diet assessment using next generation sequencing. Mol Ecol. 21(8):1931–1950. doi:10.1111/j.1365-294X.2011.05403.x.
- R Core Team. 2018. R: A language and environment for statistical computing. Vienna (Austria): R Foundation for Statistical Computing. [accessed 2018 Apr 14]. https://www.r-project.org/.
- Riofrío-Lazo M, Páez-Rosas D. 2015. Feeding habits of introduced black rats, Rattus rattus, in nesting colonies of Galapagos petrel on San Cristóbal Island, Galapagos. PLoS ONE. 10(5):1–14. doi:10.1371/journal.pone.0127901.
- Rodríguez-Sánchez F, Guzmán B, Valido A, Vargas P, Arroyo J. 2009. Late Neogene history of the laurel tree (Laurus L., Lauraceae) based on phylogeographical analyses of Mediterranean and Macaronesian populations. J. Biogeogr. 36(7):1270–1281. doi:10.1111/j.1365-2699.2009.02091.x.
- Russell JC, Ringler D, Trombini A, Le Corre M. 2011. The island syndrome and population dynamics of introduced rats. Oecologia. 167(3):667–676. doi:10.1007/s00442-011-2031-z.
- Salvande M, Gómez LA, Fernández AB. 2003. Consumo de semillas de Acebiño (Ilex canariensis) por la Rata (Rattus spp.) en distintos hábitats del monteverde en el Parque Nacional de Garajonay (La Gomera, Islas Canarias, España). In: Rodríguez-Luengo JLeditor. Control de Vertebrados Invasores en Islas de España y Portugal. Consejería de Medio Ambiente y Ordenación Territorial del Gobierno de Canarias; p. 111–116.
- Salvande M, Mulet M, Gómez LA 2001. Predación de semillas de gibalbera (Semele androgyna) por roedores en la Laurisilva canaria. Resúmenes V Jornadas de la Sociedad Española para la Conservación y Estudio de los Mamíferos (SECEM), Vitoria, Spain.
- Santos A. 1983. Vegetación y flora de La Palma. Spain: Editorial Interinsular Canaria.
- Shiels AB 2010. Ecology and impacts of introduced rodents (Rattus spp. and Mus musculus) in the Hawaiian islands [PhD thesis]. Hawaii (USA): University of Hawaii at Manoa. [accessed 2018 March 19]. http://manoa.hawaii.edu/hpicesu/DPW/AS_THESIS/AS_2010.pdf.
- Shiels AB. 2011. Frugivory by introduced black rats (Rattus rattus) promotes dispersal of invasive plant seeds. Biol Invasions. 13(3):781–792. doi:10.1007/s10530-010-9868-7.
- Shiels AB, Drake DR. 2011. Are introduced rats (Rattus rattus) both seed predators and dispersers in Hawaii? Biol. Invasions. 13(4):883–894. doi:10.1007/s10530-010-9876-7.
- Shiels AB, Flores CA, Khamsing A, Krushelnycky PD, Mosher SM, Drake DR. 2013. Dietary niche differentiation among three species of invasive rodents (Rattus rattus, R. exulans, Mus musculus). Biol Invasions. 15(5):1037–1048. doi:10.1007/s10530-012-0348-0.
- Shirako T, Ishizawa Y, Ajioka Y, Aichi M, Ueno K, Hoa DT, Hai T, Thanh TV, Yamada M, Minami M. 2015. Identification of Muridae species and their food resources using DNA barcoding in Cat Tien National Park, Vietnam. Mammal Study. 40(4):217–229. doi:10.3106/041.040.0403.
- Traveset A, Nogales M, Alcover JA, Delgado JD, López-Darias M, Godoy D, Igual JM, Bover P. 2009. A review on the effects of alien rodents in the Balearic (Western Mediterranean Sea) and Canary Islands (Eastern Atlantic Ocean). Biol Invasions. 11(7):1653–1670. doi:10.1007/s10530-008-9395-y.
- UNESCO-MAB Biosphere Reserve Directory. 2018. [accessed 2018 Apr 14]. https://www.unesco.org/.
- Venables WN, Ripley BD. 2002. Modern applied statistics with S. 4th ed. New York: Springer. ISBN 0-387-95457-0. http://www.stats.ox.ac.uk/pub/MASS4/
- Williams PA, Karl BJ, Bannister P, Lee WG. 2000. Small mammals as potential seed dispersers in New Zealand. Austral Ecol. 25(5):523–532. doi:10.1046/j.1442-9993.2000.01078.x.
- Zarzoso-Lacoste D 2013. Vers une meilleure compréhension des interactions trophiques directes et indirectes entre prédateurs invasifs et espèces natives au sein des écosystèmes insulaires [PhD thesis]. Marseille (France): University of Aix-Marseille.
- Zarzoso-Lacoste D, Bonnaud E, Corse E, Dubut V, Lorvelec O, De Meringo H, Santelli C, Meunier J-Y, Ghestemme T, Gouni A, et al. 2019. Stuck amongst introduced species: Trophic ecology reveals complex relationships between the critically endangered Niau kingfisher and introduced predators, competitors and prey. NeoBiota. 53:61–82. doi:10.3897/neobiota.53.35086.
- Zarzoso-Lacoste D, Bonnaud E, Corse E, Gilles A, Meglecz E, Costedoat C, Gouni A, Vidal E. 2016. Improving morphological diet studies with molecular ecology: an application for invasive mammal predation on island birds. Biol Conserv. 193:134–142. doi:10.1016/j.biocon.2015.11.018.
- Zarzoso-Lacoste D, Corse E, Vidal E. 2013. Improving PCR detection of prey in molecular diet studies: importance of group-specific primer set selection and extraction protocol performances. Mol Ecol Resour. 13(1):117–127. doi:10.1111/1755-0998.120.