Abstract
Toward the development of a practical separation method for trivalent actinides and lanthanides, we used extraction chromatography with alkylpyridinedicarboxyamides as the extractant. The results confirmed that the performance degradation of the adsorbent caused by contact with HNO3 and/or irradiation with gamma-rays would be very small during the operation of column chromatography. The optimal conditions for the column separation were also determined: eluent, 5 M HNO3; flow rate, 0.1 mL min−1. In addition, the change of average particle size of inert support material from 250–850 μm (XAD4) to 25–45 μm (Gelpack A040) brought about the improvement of separation between trivalent actinides and lanthanides.
1. Introduction
Spent nuclear fuels (SFs) are composed of various radioactive nuclides in addition to uranium and plutonium. After the reprocessing of SFs (i.e., the recovery of uranium and plutonium from SFs), the residual high-level radioactive waste (HLW) contains neptunium, americium and curium, namely the so-called minor actinides (MAs), together with fission products, e.g., lanthanides (Ln), cesium, and strontium. HLW is vitrified and then laid underground, i.e., it undergoes geological disposal [Citation1]. Several research groups are working to ensure that the disposal systems for HLW are as safe and reliable as possible over a very long period, and it has been revealed that the partitioning and transmutation of MAs before geological disposal are key technologies in this regard. With the partitioning and transmutation of MAs, the amount of long-lived α-radiotoxic MAs is reduced by transmutation of the MAs to short-lived or stable nuclides by neutron irradiation in accelerator-driven systems or reactors [Citation2]. For efficient transmutation, it is necessary to isolate the MAs from the fission products because of the high neutron capture cross-section of specific Ln nuclides and redundant by-products of the nuclear reaction [Citation3].
The partitioning of trivalent MAs [An(III)] from lanthanides [Ln(III)] is challenging because of the similarities of their chemical properties. Some solvent extraction systems using soft donor extractants have been proposed for the partitioning of An(III) from Ln(III); for example, N,N,N′,N′-tetrakis(2-methylpyridyl)ethylenediamine (TPEN) [Citation4], N,N′-dimethyl-N,N′-diphenylpyridine-2,6-dicarboxyamide (DMDPhPDA; Met-PDA) [Citation5,6], 2,6-bis(5,6-dialkyl-1,2,4-triazin-3-yl)-pyridine (R-BTP) [Citation7], 6,6′-bis-(5,6-dialkyl-1,2,4-triazin-3-yl)-2,2′-bipyridine (R-BTBP) [Citation8] and bis(2,4,4-trimethylpentyl)dithiophosphinic acid (Cyanex 301) [Citation9]. There is a fundamental issue associated with solvent extraction using soft donor extractants, i.e., the low solubilities of the extractants in non-polar organic solvents such as n-dodecane. One of the promising techniques for solving this problem is extraction chromatography, which provides the following advantages: (1) convenient preparation of an adsorbent by impregnation of the extractant into an inert support material, (2) an effective theoretical plate number, and (3) a concise operating procedure.
We previously developed an extractant, N,N′-dialkyl-N,N′-diphenylpyridine-2,6-dicarboxyamide (R-PDA), for the separation of An(III) from Ln(III), and we used it impregnating to an inert support material XAD4 (Amberlite) in extraction chromatography [Citation10]. The extractant R-PDA [R = butyl (But), octyl (Oct), decyl (Dec), dodecyl (Dod)] contains both two oxygen donors (amide group), which significantly coordinates to metal ions, and a nitrogen donor (pyridyl group), which has affinity to An(III) compared with that to Ln(III). Based on the results of an adsorption experiment by means of the batch method, it was revealed that Am(III) and Cm(III) were separated from lighter Ln(III), namely La to Gd, at 5 M (= mol dm−3) nitric acid (HNO3). The separation factor, which is defined as the ratio of the distribution coefficient (Kd) of Am(III) to that of Eu(III), indicated that Oct-PDA or Dec-PDA was one of the most suitable extractants. We also found that the deterioration of the prepared adsorbent, i.e., the amount of R-PDA that leaked from the prepared adsorbent as a result of contact with HNO3, was suppressed with the increasing length of the alkyl group in the impregnated R-PDA.
In the present study, we investigated the stabilities of Oct- and Dec-PDA/XAD4 adsorbent against nitric acid exposure and gamma-ray irradiation by the batch method for the development of a practical separation method for An(III) and Ln(III). We then examined the column operation conditions and determined the amount of R-PDA that leaked from the R-PDA/XAD4-packed column during column operation. The dependence of separation ability between An(III) and Ln(III) on the particle size of the inert support materials was also investigated.
2. Experimental
2.1. Materials
Two types of styrene-divinylbenzene copolymer were used as inert support materials. One is a commercial resin, XAD4 (Amberlite), and the other is Gelpack A040 (25–45 μm, Hitachi Chemical Techno Service Co. Ltd.). The physicochemical properties of the resins are summarized in . Such copolymers, which have a macroreticular and macropore structure, are stable chemically. The resins are promising candidates for inert support materials for extraction chromatography, because the macropore structure can be used for the impregnation of the extractant. Stock solutions of Am(III) and Eu(III) were prepared from 241Am(NO3)3 (Amersham Pharmacia Biotech, Buckinghamshire, UK) and 152Eu(NO3)3, respectively. All other chemicals were of analytical reagent grade.
Table 1. Physicochemical properties of XAD4 and Gelpack A040 (25 – 45 μm) resin.
2.2. Synthesis of alkylpyridinedicarboxyamide (R-PDA)
The synthetic route to and the structure of alkylpyridinedicarboxyamide (R-PDA) are shown in . We synthesized two types of R-PDA [R = octyl (Oct) and decyl (Dec)] by reacting the corresponding N-alkylaniline with 2,6-pyridinecarbonyl dichloride in the presence of triethylamine, as described [Citation10]. The purities were revealed to be >98% by high-performance liquid chromatography (YFLC AI-580, YAMAZEN) and 1H- and 13C-NMR spectroscopy (INOVA 400 MHz, Varian). Elemental analysis for C, H, and N was also performed using Amco EA1110 elemental analyzer.
2.3. Preparation of adsorbent
The adsorbent was prepared by impregnating a resin with an R-PDA. A known amount of resin was soaked in a methanol solution of R-PDA. The slurry was equilibrated for 24 h in a mechanical shaker at room temperature. The resultant material was dried under vacuum until it reached a constant weight after the excessive methanol solution was removed by filtration. The concentration of R-PDA in the prepared adsorbent was calculated from the difference in the weight between the blank resin and the prepared adsorbent. The concentrations of Oct-PDA and Dec-PDA in the prepared adsorbent were summarized in .
Table 2. Concentrations of R-PDA in prepared adsorbent.
2.4. Batch experiment
2.4.1. Influence of nitric acid exposure on adsorption performance of prepared adsorbent
Batch adsorption experiments were carried out to investigate the influence of nitric acid (HNO3) exposure on the distribution coefficient, Kd, of Am(III) and Eu(III) onto the adsorbent. In this experiment, Oct-PDA/XAD4 was tested as a representative adsorbent. The adsorbent (50 mg) was contacted with 2 mL of 5 M HNO3 solutions containing Am(III) and Eu(III). The contact time was varied from 3 hours to 12 days. After centrifugation and separation, the radioactivities of 241Am and 152Eu in the aqueous phase were measured using a gamma-ray spectrometer (GMX-30190-P, Ortec, Oak Ridge, TN, USA) equipped with a multichannel analyzer (MCA 7600, Seiko EG & G, Chiba, Japan). The Kd was calculated by Equation (1):
(1) where C0 and C indicate the radioactivities of 241Am and 152Eu in the aqueous phase before and after adsorption, respectively. VS indicates the volume of the aqueous phase (mL) and WR is the weight of the dry adsorbent (g).
2.4.2. Influence of gamma-ray irradiation on the adsorption performance of the prepared adsorbent
Irradiation with gamma-rays was done at the Co-60 gamma-ray irradiation facilities at the Takasaki Advanced Radiation Research Institute of the Japan Atomic Energy Agency. Samples in glass tubes were intermittently irradiated with gamma-rays from 1.0 × 1016 Bq 60Co source at an absorbed dose rate of 10 kGy h−1 in air at room temperature for a maximum of 50 h. A dose absorbed by each sample was corrected for the electron density. Absorbed doses were calibrated by a cellulose triacetate film dosimeter.
Two types of samples were prepared in glass tubes for gamma-ray irradiation as follows: (1) R-PDA/XAD4 in the absence of HNO3, i.e., “dry irradiation” and (2) R-PDA/XAD4 in the presence of 5 M HNO3, i.e., “wet irradiation”. To estimate the stability of R-PDA in adsorbent against gamma-ray irradiation, we determined the concentration of R-PDA before and after irradiation by gas chromatography coupled to a mass spectrometer equipped with a flame ionization detector (GCMS-QP2010Plus, Shimadzu, Kyoto, Japan) using tributylphosphate as an internal standard. The samples for dry irradiation were prepared as follows: (1) washing the 10 mg of irradiated resin with 0.5 mL of acetone, and then (2) diluting them 25-fold with acetone. Nitric acid for wet irradiation was immediately removed from the irradiated samples after irradiation, and then the 10 mg of irradiated resin was washed with 0.5 mL of distilled water five times and oven-dried at 323 K. The samples for wet irradiation were prepared in the same manner as the dry irradiation samples.
We conducted batch adsorption experiments to investigate the influence of gamma-ray irradiation onto the adsorbent on the Kd of Am(III) and Eu(III). The irradiated adsorbent (50 mg) was contacted with 2 mL of 5 M HNO3 solutions containing Am(III) and Eu(III) for 3 hours. After centrifugation and separation, the radioactivities of 241Am and 152Eu in the aqueous phase were measured using the GMX-30190-P gamma-ray spectrometer equipped with the MCA 7600 multichannel analyzer. The Kd was calculated by Equation (1) described above.
2.5. Column experiment
2.5.1. Leakage of impregnated R-PDA from the packed column
The adsorbent, Oct-PDA/XAD4, was packed in a glass column (5 mm in diameter and 200 mm in length). To determine the amount of R-PDA that leaked from the adsorbent during column operation, we introduced 5 M HNO3 into the column as an eluent at room temperature, using a double-plunger pump (NP-KX-110, Nihon Seimitsu Kagaku Co.). The effluent from the bottom of the column was collected in fractions. The amount of Oct-PDA in the effluent was determined using a total organic carbon analyzer (TOC-VCSN, Shimadzu).
2.5.2. Elution of Am(III) and Eu(III) from the packed column
A stock solution of the mixture of 241Am and 152Eu was dissolved into 5 M HNO3. We loaded a 0.1 mL portion of the prepared solution into a column in which 5 mm in diameter and 200 mm in length of the R-PDA/XAD4 was packed. Subsequently, 5 M HNO3 was introduced into the column as an eluent, using the double-plunger pump. The effluent from the bottom of the column was collected in fractions. The radioactivities of 241Am and 152Eu in the fractions were measured in the manner described above. Because the Kd of Dy(III) was almost same as that of Am(III) [Citation10], Dy(III) was used instead of Am(III). In such experiments, the concentrations of Dy(III) and Eu(III) were measured using inductively coupled plasma atomic emission spectroscopy (SPS 1200AR, Seiko EG & G).
3. Results and discussion
3.1. Stability of the prepared adsorbent against nitric acid exposure and gamma-ray irradiation
3.1.1. Influence of nitric acid exposure on adsorption performance of prepared adsorbent
shows the temporal changes of the distribution coefficient, Kd, of Am(III) and Eu(III) to Oct-PDA/XAD4 adsorbent. No deterioration of adsorbability or selectivity of Am(III) over Eu(III) was observed after the contact between R-PDA/XAD adsorbent and 5 M HNO3 from 3 hours to 12 days. This result indicates that the performance degradation of the adsorbent caused by contact with HNO3 would be very small during the operation of column chromatography.
3.1.2. Influence of gamma-ray irradiation on the adsorption performance of the prepared adsorbent
The residual ratio of Oct-PDA and Dec-PDA on the resin by the dry irradiation is shown in . The residual ratio was slightly decreased with the increase of adsorption dose, but more than 80% of the R-PDA remained, even with the approx. 400 kGy adsorption dose. shows the Kd of Am(III) and Eu(III) to Oct-PDA/XAD4 or Dec-PDA/XAD4 adsorbent by dry irradiation as a function of adsorption dose. A reduction in the selectivity of Am(III) to Eu(III) was observed on both adsorbents with the increase in the adsorption dose. The separation factor between Am(III) and Eu(III) decreased from 2.1 to 1.9 in the case of Oct-PDA/XAD4 under 465-kGy irradiation and from 1.9 to 1.7 in the case of Dec-PDA/XAD4 under 425-kGy irradiation. The Kd of Am(III) decreased at a rate greater than that of Eu(III). This tendency indicates that the pyridine moieties of the PDA molecule, which recognize or preferably coordinate to Am(III), are destroyed by gamma-ray irradiation.
Figure 3. Residual ratios of Oct-PDA and Dec-PDA in adsorbent irradiated by gamma-rays under the dry irradiation condition.
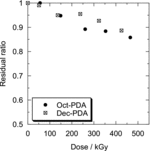
Figure 4. Distribution coefficients, Kd, of Am(III) and Eu(III) onto Oct-PDA/XAD4 (a) and Dec-PDA/XAD4 (b) irradiated by gamma-rays under the dry irradiation condition.
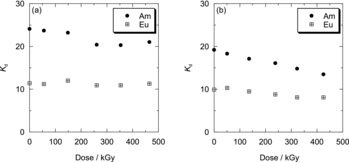
The residual ratio of Oct-PDA on the resin by the wet irradiation is shown in , together with that obtained by dry irradiation. Compared with the results of dry irradiation, no remarkable difference was observed. shows the Kd of Am(III) and Eu(III) to Oct-PDA/XAD4 adsorbent by the wet irradiation as a function of adsorption dose. The Kd of Am(III) and Eu(III) was constant after a slight increase at the 50-kGy dose. The irradiation of the 526-kGy dose caused the separation factor to decrease from 2.1 to 1.6. This result suggests that the irradiation in the presence of HNO3 solution can produce oxygen donor sites in the absorbent that exhibits oxophilicity to Am(III) and Eu(III). According to the literature on radiation chemistry of aqueous solution [Citation11], these oxygen donor sites were produced by oxidation of organic materials due to radicals generated by radiolytic decomposition of H2O and HNO3. The study on radiolysis of N,N,N′,N′-tetraoctyl-3-oxapentane-1,5-diamide [Citation12], which has a similar structure to R-PDA, indicates that the production of acidic oxygen donors due to the cleavage of amidic bond is dominant in the presence of HNO3.
3.1.3. Leakage of impregnated R-PDA from the packed column
To evaluate the stability of the column under the operating condition of separating experiments (eluent, 5 M HNO3; flow rate, 0.1 mL min−1), the leakage of impregnated R-PDA from the packed column was investigated using a maximum of 105 mL eluent. The maximum leakage amount of 2.7 ppm for Oct-PDA was observed by TOC measurement at the first fraction of 10 mL. And then, the leakage amount for Oct-PDA decreases with increasing the volume of eluent. Even if assuming that the maximum leakage amount of Oct-PDA is kept during the operation, the total leakage amount corresponds to only 0.05% of the extractant in the packed column.
3.2. Separation experiments using the R-PDA/XAD4-packed column
3.2.1. Determination of condition of separation experiments
As described above, Dy(III) can be used as substitution of Am(III) in this separation system at 5 M HNO3 [Citation10]. In the present study, before the column experiment using Am(III), we performed experiments using Dy(III) instead of Am(III) under various conditions to identify the optimal separation conditions. As a representative adsorbent, Oct-PDA/XAD4 was examined. First, we confirmed the effect of the flow rate on each elution curve in the range from 0.2 to 0.8 mL min−1. As shown in (a) and (b), it was found that the resolution between two peaks becomes better with decreasing the flow rate. (Detailed evaluation was performed in Section 3.2.2.) Then, we observed the effects of the Dy(III) and Eu(III) concentration on each elution curve. (a) shows the result obtained when the Eu(III) concentration was equal to Dy(III) concentration. In that case ([Dy] = [Eu] = 10 mM), we found that Dy(III) was separated easily from Eu(III). (b) and (c) are the results when the Eu(III) concentration was five times or 10 times the Dy(III) concentration, respectively; i.e., when [Dy] = 10 mM, [Eu] = 50 or 100 mM. No difference was observed for the position of each elution peak.
3.2.2. Evaluation of column efficiency
We next examined the separation of Am(III) from Eu(III) using both Oct-PDA/XAD4 and Dec-PDA/XAD4 adsorbents. The column operating condition was as follows; eluent, 5 M HNO3; flow rate 0.1 mL min−1 because the two elution peaks are overlapped slightly when the flow rate is 0.2 mL min−1. The elution curves obtained are shown in . The recovery rates of Am(III) and Eu(III) were calculated on the basis of radioactivity of each element. In all experiments shown in and , the recovery rates are more than 99.0%. This indicates that there is no irreversible reaction in this separation system. To evaluate the column efficiency, we fitted elution curves for Am(III) and Eu(III) using the Gaussian function, and we calculated the number of theoretical plates, N, and height equivalent to a theoretical plate, H, using Equations (2) and (3):
>(2)
(3)
Figure 9. Influence of the Eu(III) concentration on the separation of Am(III) from Eu(III) with R-PDA/XAD4. Oct-PDA/XAD4, [Am] = [Eu] = tracer (a), Dec-PDA/XAD4, [Am] = [Eu] = tracer (b), Oct-PDA/XAD4, [Am] = tracer, [Eu] = 0.1 M (c): eluent, 5 M HNO3; flow rate, 0.1 mL min−1.
![Figure 9. Influence of the Eu(III) concentration on the separation of Am(III) from Eu(III) with R-PDA/XAD4. Oct-PDA/XAD4, [Am] = [Eu] = tracer (a), Dec-PDA/XAD4, [Am] = [Eu] = tracer (b), Oct-PDA/XAD4, [Am] = tracer, [Eu] = 0.1 M (c): eluent, 5 M HNO3; flow rate, 0.1 mL min−1.](/cms/asset/71b24021-96a4-49b5-871b-49df6c0ae174/tnst_a_874301_f0009_b.gif)
Figure 10. Elution curves of Am(III) and Eu(III) with Oct-PDA/Gelpack A040 (25–45 μm) adsorbent: eluent, 5 M HNO3; flow rate, 0.1 mL min−1.
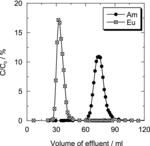
With the Oct-PDA/XAD4 adsorbent, the N and H values were found to be 43.5 and 4.60 for Am(III) and 58.0 and 3.45 for Eu(III). With the Dec-PDA/XAD4 adsorbent, the column efficiency was comparable to that of the Oct-PDA/XAD4-packed column (N = 32.3 and H = 6.19 for Am(III), and N = 38.9 and H = 5.15 for Eu(III)). We checked the elution curve of Am(III) ((c)) using the Dec-PDA/XAD4 adsorbent and 0.1 M Eu(III). No influence of Eu(III) concentration on the elution curve for Am(III) was observed (N = 29.5 and H = 6.78 for Am(III), and N = 37.7 and H = 5.30 for Eu(III)).
The H is expressed by the Van Deemter equation, i.e., Equation (4) [Citation13]:
(4) where A is the Eddy-diffusion parameter related to channeling through a non-ideal packing (m), B is the diffusion coefficient of the eluting particles in the longitudinal direction (m2 s−1), C is the resistance to the mass transfer coefficient of the analyte between the mobile and stationary phases (s), and u is the flow velocity (m s−1). According to Equation (4), the second term contributes to the decrease of H, whereas the third term contributes to the increase of H with increasing flow velocity. That is to say, the form of the Van Deemter equation is such that H achieves a minimum value at a particular flow velocity.
To investigate the effect of flow velocity on H, we determined the value of H on the basis of the elution curve of Eu(III) when the flow velocity was 0.2 or 0.8 mL min−1. The value of H decreased from 12.8 to 5.15 with the decreasing flow velocity. In contrast, the first term, which is independent of flow velocity, means that multiple distinct routes result in band spreading. In other words, the value of H is minimized by arranging the particle diameter of the inert support materials. We tested the adsorbent using Gelpack A040 (25–45 μm) as an inert support material, and the elution curve obtained is shown in . The N and H values were 239 and 0.838 for Am(III) and 109 and 1.83 for Eu(III). We also observed an improvement in the H by using the Gelpack A040 resin as the inert support material.
The resolution, Rs, between two peaks in a chromatogram is given by Equation (5):
(5) where tR1 and tR2 are the retention times for analytes 1 and 2 (tR1 < tR2) and W1 and W2 are the peak widths for analytes 1 and 2 (= 4σ), respectively. Using a relation between full width at half-maximum (FWHM) and standard deviation (σ), i.e., FWHM = 2.355σ, Equation (5) is expressed by Equation (6):
(6) With Oct-PDA as the extractant ((a) and ), we found that the RS value was 1.28 when XAD4 was used as the inert support material, and 2.51 when the Gelpack A040 was used. It was confirmed that baseline resolution was achieved by using the Gelpack A040, because the RS greater than 1.5 indicates that the mutual contamination between two components is less than 0.15%.
4. Conclusion
Extraction chromatography using Oct- or Dec-PDA as an extractant was examined toward the development of a practical separation method for An(III) and Ln(III). We investigated the stabilities of Oct- and Dec-PDA/XAD4 adsorbents under the conditions of column operation by the batch method, and we found that the adsorbent acted as proof against nitric acid exposure and gamma-ray irradiation. We also evaluated the column efficiencies of the packed column of the adsorbent by the column method. The results indicated that the optimal conditions for separation are: eluent, 5 M HNO3; flow rate, 0.1 mL min−1. In addition, the change of average particle size of inert support material from 250–850 μm (XAD4) to 25–45 μm (Gelpack A040) brought about the improvement of separation between An(III) and Ln(III).
Acknowledgements
The present study includes the results of the publication “Development of separation technology of transuranium elements and fission products by using new extractants and adsorbents” entrusted to the Japan Atomic Energy Agency by the Ministry of Education, Culture, Sports, Science and Technology of Japan (MEXT).
References
- Japan Atomic Energy Commission, Framework for Nuclear Energy Policy, Chapters 1–3, 2005. Available from: http://www.aec.go.jp/jicst/NC/eng/index.htm
- Nakayama S, Morita Y, Nishihara K, Oigawa H. Geological disposal of radioactive waste produced by application of partitioning-transmutation technology to nuclear fuel cycle. Proceedings of International Conference on Nuclear Energy System for Future Generation and Global Sustainability (GLOBAL 2005); Tsukuba, Japan; 2005. CD-ROM.
- Oh, S-Y, Chang J, Mughabghab SF. Neutron cross section evaluations of fission products below the fast energy region. BNL-NCS-67469 Informal Report; 2000.
- Watanabe M, Mirvaliev R, Tachimori S, Takeshita K, Nakano Y, Morikawa K, Mori R. Separation of americium(III) from lanthanide by encapsulating hexadentate-ligand. Chem Lett. 2002;31(12):1230–1231.
- Shimada A, Yaita T, Narita H, Tachimori S, Kimura T, Okuno K, Nakano Y. Extraction of Am(III) and lanthanide(III) ions from HNO3 solutions using N,N′-dimethyl-N,N′-diphenylpyridine-2,6-dicarboxyamide. Solvent Extraction Res Dev Japan. 2004;11:1–10.
- Shimada A, Yaita T, Narita H, Tachimori S, Okuno K. Extraction studies of lanthanide(III) ions with N,N′-dimethyl-N,N′-diphenylpyridine-2,6-dicarboxyamide (DMDPhPDA) from nitric acid solutions. Solvent Extraction Ion Exchange. 2004;22(2):147–161.
- Kolarik Z, Müllich U, Gassner F. Extraction of Am(III) and Eu(III) nitrates by 2,6-di-(5,6-dipropyl-1,2,4-triazin-3-yl)pyridines. Solvent Extraction Ion Exchange. 1999;17(5):1155–1170.
- Drew MGB, Foreman MRSJ, Hill C, Hudson MJ, Madic C. 6,6′-bis-(5,6-diethyl-[1,2,4]triazin-3-yl)-2,2′-bipyridyl the first example of a new class of quadridentate heterocyclic extraction reagents for the separation of americium(III) and europium(III). Inorg Chem Commun. 2005;8(3):239–241.
- Zhu Y, Chen J, Jiao R. Extraction of Am(III) and Eu(III) from nitrate solution with purified Cyanex 301. Solvent Extraction Ion Exchange.1996;14(1):61–68.
- Arisaka M, Watanabe M, Kimura T. New alkyl-pyridinedicarboxyamides as extractants for separation of trivalent minor actinides from fission products by extraction chromatography. Radiochim Acta. 2013;101:711–717.
- Mozumder M, Hatano Y, editors. Charged particle and photon interactions with matter. New York (NY): Marcel Dekker; 2004. Chapters 12 and 23.
- Sugo Y, Sasaki Y, Tachimori S. Studies on hydrolysis and radiolysis of N,N,N′,N′-tetraoctyl-3-oxapentane-1,5-diamide. Radiochim Acta. 2002;90:161–165.
- Van Deemter JJ, Zuiderweg FJ, Klinkenberg A. Longitudinal diffusion and resistance to mass transfer as causes of nonideality in chromatography. Chem Eng Sci. 1956;5:271–289.