Abstract
Core characteristics of a sodium-cooled fast breeder reactor (FBR) with 750 MWe output using highly decontaminated uranium and plutonium and highly minor-actinide-containing compositions were evaluated using the fast reactor cross-section set generated by the new Japanese nuclear data library JENDL-4.0. The core characteristics were compared with those obtained using the unified cross-section set ADJ2000R in order to investigate the differences between both the results. The effects on the core characteristics caused by the differences in the nuclear data of important reactions and nuclides in the cross-section sets were analyzed by a burnup sensitivity analysis. It was confirmed that adopting JENDL-4.0 to the FBR core design improves the breeding ratio, the burnup reactivity, and the reactivity control balance, because of the differences in the capture cross-sections of U-238 and Pu-239 of both the libraries. The difference in the sodium void reactivity evaluated with both the libraries was less than 1% because the increase caused by the differences in the elastic scattering cross-sections of sodium, the inelastic scattering cross-section, and the μ-average value of U-238 was practically cancelled out by the decrease caused by the differences in the capture cross-sections of Pu-239, the inelastic scattering cross-section of iron, and the capture cross-sections of Am-241.
1. Introduction
Fast breeder reactor (FBR) technology is one of the important energy options, which can meet the growing future energy demand in the world and reduce the environmental burden caused by radiological waste generated by nuclear power production.
For the FBR design, the unified cross-section set ADJ2000R, which was generated from the JENDL-3.2 by the cross-section adjustment technique adopting integral data obtained from various independent fast neutron critical facilities and reactors, was utilized for a long time [Citation1,2]. Many studies on FBR designs had been performed using ADJ2000R until recently [Citation3–5].
Meanwhile, the new nuclear data library JENDL-4.0 was released in 2010 [Citation6]. In this library, neutron reaction cross-sections of important nuclides for the FBR core design including minor actinides (MA) and fission products were improved both in quality and quantity. In addition, the neutron reaction cross-sections of uranium and plutonium isotopes were reevaluated.
Benchmark testing for the new data library was carried out using a large amount of integral data including criticality data and post-irradiation test data for fast neutron systems. It was confirmed that calculation/experiment values were considerably improved in JENDL-4.0 [Citation7].
For this reason, adopting the fast reactor cross-section set generated by the JENDL-4.0 to the FBR core design is expected to improve accuracy in predicting core characteristics [Citation8]. In addition, other characteristics such as the decay heat and the heavy metal (HM) element composition can be influenced by improving the nuclear data of MA nuclides.
To apply the new library to the FBR core design, it is important to identify the differences between the two nuclear data-sets and understand how the differences affect the core characteristics. Comprehensive and systematic understanding of the effects caused by the differences between ADJ2000R and JENDL-4.0 enables us to predict and estimate the effects on the core characteristics of the FBR designs, which were studied by ADJ2000R [Citation3–5]. This investigation is particularly important to the study of core designs for a medium-sized FBR, which was studied in detail [Citation9].
In the present study, the core characteristics of a 750 MWe sodium-cooled FBR with highly decontaminated uranium and plutonium and highly MA-containing compositions were evaluated by a diffusion and burnup calculation with JENDL-4.0 and ADJ2000R. The core characteristics evaluated using both the libraries were compared, and the differences in the core characteristics evaluated with both the libraries were investigated by a burnup sensitivity analysis [Citation10–12].
2. Calculation
2.1. Core specifications and fuel compositions
2.1.1. Core specifications
The important specifications of the 750 MWe FBR are listed in . Thermal and electric power outputs are 1765 and 750 MW, respectively. The core outlet and inlet temperatures are 550 and 395 °C, respectively. The operation cycle length is set to 18 months, and the fuel exchange is performed in six batches. The driver core height is 1.00 m. The upper and lower axial blanket lengths are 200 and 250 mm. The core equivalent diameter is 3.75 m. The core contains 274 driver subassemblies and 27 control rods (CRs), and it is surrounded by the axial blanket fuel and one layer of radial blanket sub-assemblies as shown in .
Table 1. Specifications of the 750 MWe sodium-cooled fast breeder reactor.
2.1.2. Fuel compositions
We assumed two representative fuel compositions to investigate the important core characteristics such as the reactivity control balance and the sodium void reactivity, although a variety of transuranic (TRU) compositions can be recovered by reprocessing spent LWR fuel.
It was confirmed that there is a correlation between the sodium void reactivity and the burnup reactivity, which is an important factor to the reactivity control balance because MA nuclides contribute to increase the sodium void reactivity and improve the burnup reactivity [Citation13].
It is expected that highly MA-containing fuel composition with relatively lower fissile concentration gives higher sodium void reactivity but lower burnup reactivity, which is beneficial for the reactivity control balance. However, highly decontaminated uranium and plutonium fuel composition with relatively higher fissile concentration causes lower sodium void reactivity but higher burnup reactivity.
Considering TRU practical compositions, which can be recovered from spent LWR fuel, we assumed two representative compositions, as described in . The terms “highly decontaminated U–Pu” and “lowly decontaminated TRU” here mean highly decontaminated uranium and plutonium fuel composition with relatively higher fissile concentration and highly MA-containing fuel composition with relatively lower fissile concentration.
Table 2. TRU composition.
As to the highly decontaminated U–Pu fuel composition, we assumed the TRU recovered from 45 to 49 GWd/t spent LWR fuel in the plutonium uranium extraction (PUREX) reprocessing process after 2 years of cooling and stored for 6 years after the reprocessing. As to the lowly decontaminated TRU fuel composition, we assumed the TRU stored for 40 years after discharge and recovered from the same spent LWR fuel by a TRU co-extraction reprocessing, which recovers neptunium, americium, and curium as well as plutonium. In both the compositions, it was also assumed that the TRU is recovered with the same amount of uranium in the reprocessing and then mixed with depleted uranium to fabricate core fuel.
The sodium void reactivity in a core with the lowly decontaminated TRU fuel composition is expected to be the maximum value. We can also evaluate the most conservative case in the reactivity control balance in a core with the highly decontaminated U–Pu fuel composition. Thus, the investigation with these two compositions is expected to give us the comprehensive core characteristics of the FBR.
2.2. Methods
2.2.1. Diffusion and burnup calculation
The burnup characteristics such as the keff value, the burnup reactivity, and the breeding ratio were evaluated for the core with the lowly decontaminated TRU composition and the highly decontaminated fuel U–Pu by a diffusion and burnup calculation [Citation14]. The reactivity control balance was evaluated for the core with the highly decontaminated fuel U–Pu [Citation15].
Reactivity characteristics such as the sodium void reactivity and the Doppler coefficient were evaluated with the composition at the end of an equilibrium cycle. The sodium void reactivity was evaluated by assuming a loss of sodium coolant inside fuel subassemblies except for inner ducts in the core region. The Doppler coefficient was calculated by assuming a temperature increase of 500 K in driver fuel subassemblies.
Self-shielded cross-sections were prepared by a cell calculation code [Citation16] using the reactor cross-section sets based on JENDL-4.0 and ADJ2000R.
2.2.2. Adjustment of Pu enrichment
The values of the Pu enrichment of the inner and outer core fuels were adjusted as low as possible, ensuring the core would maintain its criticality until the end of an equilibrium cycle and leveling the maximum linear heat rates of the inner and outer core fuels.
2.2.3. Burnup sensitivity analysis
The sensitivity coefficients for the core characteristics with the lowly decontaminated TRU fuel in the keff value, the breeding ratio, the sodium void reactivity, and the number density were calculated by a burnup sensitivity analysis code system based on a generalized perturbation theory [Citation10–12].
The effects on the core characteristics by each nuclide and reaction were estimated by multiplying the sensitivity coefficients Sx,g by the relative differences of each nuclear data in accordance with Equation (1):
(1) where R is some core characteristic such as the breeding ratio and the sodium void reactivity, and σx,g represents a cross-section or other nuclear data such as a ν-value for a nuclide-and-reaction x and an energy group g.
This analysis showed how the differences in nuclear data apply to the core characteristics, although the accuracy of the estimated effects on the core characteristics by this methodology was limited because of an approximation of the theory, differences in the group cross-section structure, and so on.
3. Results and discussion
3.1. Characteristics of the highly decontaminated U–Pu fuel core
3.1.1. Burnup characteristics
The important characteristics of the 750 MWe FBR core with the highly decontaminated U–Pu fuel evaluated by JENDL-4.0 and ADJ2000R are listed in .
Table 3. Core characteristics of 750 MWe SFR with highly decontaminated U–Pu fuel.
The Pu enrichment in the case calculated with JENDL-4.0 was lower than that with ADJ2000R. The burnup reactivity was lower than that evaluated with ADJ2000R because the breeding ratio with JENDL-4.0 was improved comparing to that with ADJ2000R.
3.1.2. Reactivity control balance
The reactivity control balance evaluated with JENDL-4.0 (Case 1–1) and by ADJ2000R (Case 1–2) are listed in . The CR worth was evaluated by assuming that the most valuable rod stuck accidentally and considering of uncertainty of the CR worth [Citation17].
Table 4. Reactivity control balance of 750 MWe SFR with highly decontaminated U–Pu fuel.
The CR worth of the primary CR (PCR) system and the backup CR (BCR) systems evaluated by JENDL-4.0 was almost same as that of ADJ2000R. The shutdown margin was improved for the PCR in the case evaluated by JENDL-4.0 because the power defect and the burnup loss (burnup reactivity) were lower than that by ADJ2000R.
It was also beneficial to reduce the reactivity insertion in the CR withdrawal accident. Relatively lower power defect and burnup loss allow PCRs to stay upper position, which caused smaller amount of reactivity insertion in a CR withdrawal accident.
3.2. Characteristics of the lowly decontaminated TRU fuel core
3.2.1. Burnup characteristics
The important characteristics of the 750 MWe FBR core with the lowly decontaminated TRU fuel evaluated using JENDL-4.0 and ADJ2000R are listed in .
Table 5. Core characteristics of 750 MWe SFR with lowly decontaminated TRU fuel.
The core characteristics, including the Pu enrichment evaluated with JENDL-4.0 and ADJ2000R (Cases 2–1 and 2–3), which shows us the practical core design based on both the libraries, are listed in . The values of Pu enrichment of the inner and outer core fuels were 17.8 and 24.2 wt% in the calculation with JENDL-4.0, and 18.0 and 24.3 wt% with ADJ2000R. The breeding ratio with JENDL-4.0 was higher, and the burnup reactivity was lower than those evaluated with ADJ2000R, as was found in the case of the highly decontaminated fuel core in . It was confirmed that these characteristics evaluated with JENDL-4.0 were improved for both the compositions.
Another calculation with JENDL-4.0 (Case 2–2) on the same Pu enrichment calculated with ADJ2000R (Case 2–3) was performed to confirm the differences of the core characteristics under the same condition. The burnup reactivity and the breeding ratio evaluated with JENDL-4.0 were improved as found in the comparison between Case 2–1 and Case 2–3. The difference in the keff value in the equilibrium core evaluated with JENDL-4.0 and ADJ2000R was less than 0.05%. However, the keff value with JENDL-4.0 was lower by 0.4% than that with ADJ2000R in the initial load core.
3.2.2. Reactivity characteristics
The values of the sodium void reactivity calculated with JENDL-4.0 in Cases 2–1 and 2–2 were the same as those with ADJ2000R in Case 2–3 listed in . The sodium void reactivity was calculated with JENDL-4.0 using the composition at the end of an equilibrium cycle calculated with ADJ2000R (Case 2–3), and it was the same as that with ADJ2000R.
3.2.3. Composition of spent fuel
lists the relative differences in the number densities of each nuclide in the discharged spent fuel evaluated by the diffusion and burnup calculation with JENDL-4.0 and ADJ2000R on the same Pu enrichment (comparison between Case 2–2 and Case 2–3).
Table 6. Differences in TRU compositions of discharged spent fuel compared with the same Pu enrichment.
The number densities of Pu-239 in the spent fuel in the inner and outer core regions as well as in the axial blanket region evaluated by JENDL-4.0 were higher by 0.9%–1.4% than that by ADJ2000R, from the data given in . However, the number density of Pu-239 in the radial blanket did not change. In addition, the number densities of Pu-241 in the core, the axial, and the radial blanket regions evaluated by JENDL-4.0 were lower than that by ADJ2000R.
The relative difference between the spent fuel compositions evaluated with each library at the midplane of the core and the innermost part of the outer core region, where the highest decay heat in the core is found, was also evaluated. Regarding nuclides, which were important contributors to decay heat, the number densities of Pu-238 and Cm-244 in the spent fuel increased by 3.6% and 0.8%, respectively, and the amount of Am-241 and Cm-242 decreased by 3.6% and 0.9%, respectively.
3.2.4. Decay heat of spent fuel
shows the decay heat caused by HM elements in the discharged spent fuel composition evaluated at the midplane of the core and the innermost part of the outer core region. In comparing calculations for the same Pu enrichment (Cases 2–2 and 2–3), the HM decay heat evaluated with JENDL-4.0 was larger by about 1% at most until about a year had passed since the discharge of the spent fuel and increased by about 2% at most ADJ2000R after several decades of discharge because of the differences of amounts of MA nuclides. Neptunium-239, which was an important contributor of the decay heat within a few days of the discharge, increased about 3% in the calculation with JENDL-4.0. After a year, Pu-238 and Cm-244 became the important contributors, and then Pu-238, which has a relatively longer half-life than Cm-244, became more dominant. Although it was less significant than other nuclides, Am-241 decreased by about 4% in the result calculated with JENDL-4.0 and contributed to reduce the decay heat.
3.3. Burnup sensitivity analysis of the lowly decontaminated TRU fuel core
The tendency of the differences between the core characteristics evaluated with JENDL-4.0 and ADJ2000R was similar in the core with the highly decontaminated U–Pu fuel and the lowly decontaminated TRU fuel, in terms of the breeding ratio, the burnup reactivity, and the sodium void reactivity.
To investigate these differences, we performed the burnup sensitivity analysis for the lowly decontaminated TRU fuel core. In this composition, the impact caused by the differences in the nuclear data on the core characteristics is expected to be greater than in the other composition because of its higher concentration of MA.
The sensitivity coefficients of the core characteristics with the lowly decontaminated TRU fuel such as the keff value, the breeding ratio, the sodium void reactivity, and the number density of Pu-238 were calculated by the burnup sensitivity analysis code system based on the generalized perturbation theory.
3.3.1. Criticality
As can be seen from the data in , the keff value calculated with JENDL-4.0 in the equilibrium core was larger than that with ADJ2000R on the same Pu enrichment (Cases 2–2 and 2–3). However, the keff value in the initial load core with JENDL-4.0 was smaller than that with ADJ2000R, oppositely.
These results suggested that the relatively large capture cross-section of U-238 of JENDL-4.0 compared to ADJ2000R reduced the keff value in the initial load core, but increased the keff value in the equilibrium core though the burnup process with a high conversion ratio caused by its larger capture cross-section.
To confirm this expectation, the sensitivity analysis of the keff value in the initial load core was done as shown in . Although the differences in the inelastic scattering cross-section of U-238, the capture cross-sections of Pu-239, and iron contribute to the increase in keff, the differences in the capture cross-section of U-238 and the inelastic scattering cross-section of iron decreased keff. The difference in the capture cross-section of U-238 was obviously an important factor in the initial load core in reducing the keff value with JENDL-4.0, as it was expected from the differences in the core characteristics.
shows a breakdown of the burnup sensitivity coefficients of the capture cross-section of U-238 such as the direct term, the flux term, and the number density term in the equilibrium core. The number density term, which is a factor that affects the core characteristics indirectly though changes in the number densities of daughter nuclides such as Pu-239 caused by the differences of cross-sections, reduced the large effect of the direct term. Because of the lack of the positive values of the number density term, the sensitivity coefficients of the capture cross-section of U-238 became large negative values in the initial load core. On the other hand, the sensitivity coefficients of the capture cross-section of U-238 of the equilibrium core were smaller than those of the initial load core because of the positive values of the number density term.
Figure 4. Breakdown of the sensitivity coefficient of the capture cross-section of U-238 for the keff value.
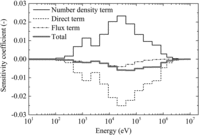
shows the sensitivity coefficients of the initial load core and the equilibrium core, and the difference in the capture cross-section of U-238 and its effect on the keff value. Unlike the large negative values of the sensitivity coefficients of the initial load core, the coefficients of the equilibrium core were small because of their number density terms, as shown in . In this reason, the difference in the capture cross-section of U-238 was no longer an important factor to reduce the keff value in the equilibrium core, as it was clearly found in the comparison between and . Finally, it was confirmed that the effect of the difference in the capture cross-section of U-238 to reduce the keff value became less significant with burnup. This is why the keff value in the equilibrium core with JENDL-4.0 was almost same as that of ADJ2000R regardless of the lower keff value with JENDL-4.0 in the initial load core.
3.3.2. Breeding ratio
The number densities of Pu-239 in spent fuel in all the region evaluated by JENDL-4.0 were higher than those by ADJ2000R, whereas the number densities of Pu-241 were smaller than those with ADJ2000R, as can be seen from the data in . The differences in the number densities of Pu-240 in the inner and outer core regions evaluated with JENDL-4.0 and ADJ2000R were 0.2% although the number densities of Pu-239 with JENDL-4.0 were greater by 0.9%–1.4% than those with ADJ2000R. This suggested that the larger breeding ratio evaluated with JENDL-4.0 compared to that of ADJ2000R can be attributed to an increase of the capture cross-section of U-238 and a decrease of the capture cross-section Pu-239.
To confirm this estimation, the sensitivity analysis was performed for the breeding ratio and is shown in . The differences in the capture cross-sections of U-238 and Pu-239 were important contributors to the increase in the breeding ratio with JENDL-4.0, as was expected.
shows an example of the sensitivity analysis of the breeding ratio of the capture cross-section of Pu-239. The sensitivity coefficients of the breeding ratio of the capture cross-section of Pu-239 were negative. The capture cross-section of Pu-239 of JENDL-4.0 is smaller by 10% at maximum in the range of 1–10 keV and smaller by 13% at maximum in the range of 40–400 keV than that of ADJ2000R. It was obvious that the negative sensitivity coefficients and the smaller capture cross-section of Pu-239 provided positive contributions to the breeding ratio in the calculation with JENDL-4.0.
3.3.3. Sodium void reactivity
The results of the sensitivity analysis for the sodium void reactivity in show that the sodium void reactivity with JENDL-4.0 was the same as that with ADJ2000R, as can be seen from the data in . The increase caused by the differences in the elastic scattering cross-sections of sodium, the inelastic scattering cross-section and μ-average value of U-238, and the ν-value of Pu-239 was canceled out by the decreases caused by the differences in the capture cross-sections of Pu-239, the inelastic scattering cross-section of iron, and the capture cross-sections Am-241.
shows the sensitivity coefficients of the ν-value of Pu-239 as one of the factors that increased the sodium void reactivity. The energy distribution of the sensitivity coefficients of the sodium void reactivity was different and more complex than that of the keff value. When a void is induced in the sodium coolant in the core region, the neutron spectrum becomes harder. Thus, the sensitivity coefficients below 2 keV are largely negative, and the coefficients above 105 eV are positive for ν-values of HMs. Because the relative difference in the ν-value of Pu-239 has an energy distribution that was coincidentally similar to its sensitivity coefficients, the differences in the ν-value of Pu-239 contributed relatively largely to the sodium void reactivity despite the small difference, which only slightly influenced the keff value shown in . In , it can also be seen that the elastic scattering cross-section of sodium was larger than that from ADJ2000R by 2% in the 20–800 keV range, where the sensitivity coefficients were positive.
Figure 11. Effect on sodium void reactivity caused by the difference in the elastic scattering cross-section of sodium-23.
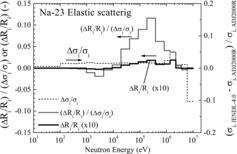
shows the sensitivity coefficients of the capture cross-section of Am-241. The capture cross-section of Am-241 in JENDL-4.0 was about 10% lower than that of ADJ2000R in the region below 2 keV, where the sensitivity coefficients were positive. These differences were important contributors to reducing the sodium void reactivity in JENDL-4.0 compared to ADJ2000R.
3.3.4. Number density of Pu-238 in spent fuel
Pu-238 is one of the important contributors to the decay heat of spent fuel. The number density of Pu-238 in the outer core region evaluated with JENDL-4.0 was higher than that with ADJ2000R, as can be seen from the data in .
The number density of Pu-238 was largely affected by the capture cross-section of its parent nuclide Np-237, and the capture and fission cross-sections of Pu-238 itself as shown in .
The capture cross-section of Np-237 in JENDL-4.0 was smaller than that in ADJ2000R in the region from 450 eV to 400 keV, where the sensitivity coefficients were largely positive, as shown in . This difference in the capture cross-section reduced the number density of Pu-238. However, both the capture and fission cross-sections of Pu-238 in JENDL-4.0 were smaller than those in ADJ2000R by 14% at maximum in the 450 eV–400 keV range and 20% at maximum in the 2 keV–1.4 MeV range, respectively, in the region with the negative sensitivity coefficients, as shown in and , respectively. These differences in the capture and fission cross-sections increased the number density of Pu-238.
Figure 14. Effect on the atomic number density of Pu-238 caused by the difference in the capture cross-section of Np-237.
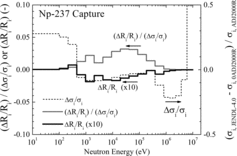
Figure 15. Effect on the atomic number density of Pu-238 caused by the difference in the capture cross-section of Pu-238.
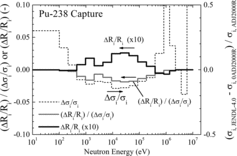
Figure 16. Effect on the atomic number density of Pu-238 caused by the difference in the fission cross-section of Pu-238.
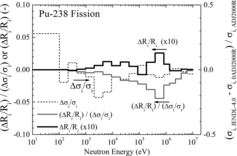
The increase in the number density of Pu-238 due to the differences in the capture and fission cross-sections of Pu-238 exceeded the effect of the difference in the capture cross-section of Np-237, while the difference in the capture cross-section of Np-237 contributed to decrease the number density of Pu-238.
Although the relatively smaller capture cross-sections of Pu-238 in JENDL-4.0 is expected to contribute to decrease the breeding ratio slightly, the effect caused by this was smaller than 0.1% as in .
4. Conclusion
The core characteristics of the 750 MWe sodium-cooled FBR with the highly decontaminated U–Pu and the lowly decontaminated TRU fuel were evaluated using ADJ2000R and the fast reactor cross-section set based on JENDL-4.0.
Although there was no significant effect on the FBR core characteristics caused by the differences in the nuclear data from both the libraries, the improvements in the breeding ratio, the burnup reactivity, and the reactivity control balance were confirmed. The difference in the sodium void reactivity was less than 1%. The decay heat caused by TRU elements increased by 2% at most in the spent fuel with the lowly decontaminated TRU.
The effects on the core characteristics caused by the differences in the nuclear data of important reactions and nuclides were analyzed by the burnup sensitivity analysis method for the core with the lowly decontaminated TRU fuel.
The keff value evaluated for the initial load core on the same Pu enrichment using JENDL-4.0 was sma- ller than that by ADJ2000R because of the differences in the capture cross-section of U-238, the inelastic scattering cross-section of iron and so on, although the differences in the inelastic scattering cross-section of U-238, the capture cross-section of Pu-239, and the capture cross-section of iron contribute to increase the keff value. The keff value in the equilibrium core with JENDL-4.0 was larger than that with ADJ2000R, since the effect of the capture cross-section of U-238 to decrease the keff value became less significant in the equilibrium core.
The breeding ratio with JENDL-4.0 was improved because of the differences in the capture cross-sections of U-238 and Pu-239. The differences also made the burnup reactivity lower and improved the reactivity control balance.
The difference in the sodium void reactivity calculated with JENDL-4.0 and ADJ2000R was less than 1%, because the increase caused by the differences in the elastic scattering cross-sections of sodium, the inelastic scattering cross-section, and the μ-average value of U-238 was practically canceled out by the decrease caused by the differences in the capture cross-sections of Pu-239, the inelastic scattering cross-section of iron, and the capture cross-sections of Am-241.
The number density of Pu-238 increased because of the decrease in the capture and fission cross-sections of Pu-238. It was confirmed that the increase in the number density of Pu-238 by about 4% contributed to increase the decay heat of the spent fuel in the outer core region.
Acknowledgements
The authors wish to express their gratitude to Mr A. Soga and Mr H. Komoda from NESI, Inc., for their assistance with the preparatory work for calculations in this study.
References
- Ishikawa M, Numata K, Sato W, Sugino K. Development of the unified cross-section set ADJ2000 for fast reactor analysis ( JNC TN9400 2001-071). Japan: Japan Nuclear Cycle Institute; 2001. Japanese.
- Hazama T, Chiba G, Numata K, Sato W. Development of the unified cross-section set ADJ2000R for fast reactor analysis ( JNC TN9400 2002-064). Japan: Japan Nuclear Cycle Institute; 2002. Japanese.
- Mizuno T, Ogawa T, Naganuma M, Aida T. Advanced oxide fuel core design study for SFR in the ‘Feasibility Study’ in Japan. Proceedings of the GLOBAL 2005; 2005 Oct 9–13; Tsukuba (Japan). [CD-ROM].
- Naganuma M, Ogawa T, Ohki S, Mizuno T, Kotake S. Minor actinide-bearing oxide fuel core design study for the JSFR. Nucl Technol. 2010;170:170–180.
- Okubo T, Ohki S, Ogura M, Okubo Y, Kotake S. Conceptual design for a large-scale Japan sodium-cooled fast reactor (3): core design in JSFR. Proceedings of the International Congress Advances in Nuclear Power Plants 2011; 2011 May 2–6; Nice (France).
- Shibata K, Iwamoto O, Nakagawa T, Iwamoto N, Ichihara A, Kunieda S, Chiba S, Furutaka K, Otuka N, Ohsawa T, Murata T, Matsunobu H, Zukeran A, Kamada S, Katakura J. JENDL-4.0: a new library for nuclear science and engineering. J Nucl Sci Technol. 2011;48:1–30.
- Chiba G, Okumura K, Sugino K, Nagaya Y, Yokoyama K, Kugo T, Ishikawa M, Okajima S. JENDL-4.0 benchmarking for fission reactor applications. J Nucl Sci Technol. 2011;48:172–187.
- Sugino K, Jin T, Hazama T, Numata K. Preparation of fast reactor group constant sets UFLIB.J40 and JFS-3-J4.0 based on the JENDL-4.0 data ( JAEA-Data/Code 2011-017). Japan: Japan Atomic Energy Agency; 2012. Japanese.
- Aoto K, Uto N, Sakamoto Y, Ito T, Toda M, Kotake S. Design study and R&D progress on Japan sodium-cooled fast reactor. J Nucl Sci Technol. 2011;48:463–471.
- Takeda T, Umano T. Burnup sensitivity analysis in a fast breeder reactor – Part I: sensitivity calculation method with generalized perturbation theory. Nucl Sci Eng. 1985;91:1–10.
- Hanaki H, Sanda T, Ohashi M. Preparation of computer codes for analyzing sensitivity coefficients of burnup characteristics (II) ( PNC TJ9124 94–007). Japan: Power Reactor and Nuclear Fuel Development Corporation; 1994. Japanese.
- Tatsumi M, Hyoudou H. Systemization of burnup sensitivity analysis code (II) ( JAEA-Review 2008-038). Japan: Japan Atomic Energy Agency; 2008.
- Maruyama S, Ohki S, Okubo T, Kawashima K, Mizuno T. Correlations among FBR core characteristics for various fuel compositions. J Nucl Sci Technol. 2012;49:640–654.
- Fowler TB, Vondy DR, Cunningham GR. Nuclear reactor core analysis code: CITATION ( ORNL-TM-2496, Rev.2). Oak Ridge (TN): Oak Ridge National Laboratory; 1971.
- Hara A. Development of three-dimensional diffusion calculation code MOSES (II) ( PNC-TN9520-89-018). Japan: Power Reactor and Nuclear Fuel Development Corporation; 1989. Japanese.
- Nakagawa M, Tsuchihashi K. SLAROM: a code for cell homogenization calculation of fast reactor ( JAERI 1294). Japan: Japan Atomic Energy Research Institute; 1984.
- Ogawa T, Kobayashi N, Ohki S, Naganuma M, Kubo S, Mizuno T. Study on reactor core and fuel design of sodium-cooled fast reactor (mixed oxide fuel core)–Results in JFY2006–JAEA-Research 2007-084. Japan: Japan Atomic Energy Agency; 2008. Japanese.