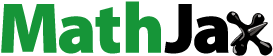
Abstract
Enhancement of uranium extraction from seawater using chromic-acid-treated amidoxime adsorbent was studied. Chromic-acid-treated amidoxime fibers were synthesized based on the simultaneous irradiation grafting method at a low temperature. Low-density polyethylene (LDPE) fibers were treated with chromic acid for up to 90 minutes. After the treatment, the fibers were submerged in 60:40 acrylonitrile:methacrylic acid monomer by volume and irradiated with 40 kGy γ-ray. The maximum grafting efficiency of about 90% occurred at 20 minutes of acid treatment time, as a significant enhancement of 30% compared to the literature-reported value of about 70%. When submerged in shallow seawater with an average temperature of 30 °C for 4 weeks, the amidoxime adsorbent exhibited the adsorption capacity of 2.06 g-U/kg-adsorbent, which was 37% higher than the literature-reported value. These significantly increased grafting and adsorption efficiencies were attributed to the increased surface area of chromic-acid-etched LDPE fibers. Moreover, for the submersion up to 8 weeks, the adsorption increased to 2.15 g-U/kg-adsorbent. The adsorption capacity was evaluated to reduce to about 65% after eight cycles of repeated usage. Uranium concentrations in Thailand's seawater collected at various depths and locations were analyzed using inductively coupled plasma-mass spectrometry (ICP-MS) to be about 3 ppb everywhere.
1. Introduction
Natural uranium is a raw material for the production of nuclear fuels for research and power reactors. Conventional uranium resources have been obtained from uranium mines and by-products from other mines. Assuming that the demand for uranium remains constant, conventional resources are expected to last for about 80 years. There have been several interests and experimental works on the recovery of uranium from seawater, since it is estimated that there are about 4.5 billion tons of uranium ion dissolved in seawater worldwide. If only half of this quantity can be extracted, it can provide natural uranium for the nuclear industry for about 6500 years under the assumption that the electricity production from nuclear power plants worldwide increases about eight times (to about 3000 GWe). Therefore, seawater can be considered as an inexhaustible resource of natural uranium.
Seawater contains a large number of dissolved elements, as there are about 80 detectable elements so far. However, the chemistry of seawater is controlled by the following six main ions: CI−, Na+, Mg2+, Ca2+, K+ and SO42−, as they constitute more than 99.5% of all the ionic species dissolved in seawater [Citation1]. Uranium is present in seawater at an average concentration of about 3.3 ppb (about 1000 times lower than the 3 ppm average concentration in tectonic plates), and is present mainly as the tricarbonate uranylate anion, [UO2(CO3)3]4−, as shown in . Moreover, uranium concentration in seawater appears to be constant in almost all areas of the oceans and their depths [Citation2,3].
Table 1. Concentrations of chemical species of uranium dissolved in natural seawater at 25 °C and pH 8.1 [Citation1].
There have been several successful studies on uranium extraction from seawater, and the first one dated back to year 1953. Different adsorbents have been studied, which can be categorized into four categories: (1) porous membrane, (2) polymers, (3) inorganic compounds (e.g., lead naphthalene tetracarboxylate, lead pyrophosphate, lead stannate, lead sulfide, hydrous titanium oxide and basic zinc carbonate) and (4) chemical functional groups grafted onto substrates. Functional groups exhibiting the ability to adsorb uranium in seawater by displacing carbonate ions in [UO2(CO3)3]4− are listed in .
Table 2. Functional groups exhibiting the ability to adsorb uranium by displacing carbonate ions in [UO2(CO3)3]4− [Citation1].
The most recent progress on uranium extraction from seawater was made by Tamada [Citation4]. Radiation grafting of the amidoxime functional group onto woven low-density polyethylene (LDPE) fabrics and non-woven LDPE fibers was carried out, and adsorbents were employed to adsorb uranium from Japan's seawater. It was concluded that the amidoxime-grafted woven fabric exhibited the uranium adsorption capacity of about 1.5 mg/g adsorbent for a soaking time of 30 days in 30 °C Okinawa seawater. For the same soaking time, the woven LDPE fabrics exhibited the capacity of only about 0.5 mg/g adsorbent in 20 °C Aomori seawater. It was concluded that the effect of 10 °C difference in seawater temperature contributed to 1.5 times and that a greater surface area of non-woven LDPE fibers compared to woven LDPE fabrics contributed 2 times, leading to a total of about 3 times greater uranium adsorption capability of the fibers submerged in warmer seawater compared to that of the fabrics submerged in a colder area.
Since it is evident that the surface area of the adsorbent plays an important role in the efficiency of uranium adsorption, the present work discusses the enhancement of uranium extraction from seawater using chromic-acid-modified amidoxime fibers, as chromic acid can be used to treat polymer surface to increase the surface area [Citation5]. The ability of the adsorbent to be reused was evaluated as well.
It is important to note that although there are several resins exhibiting much higher uranium adsorption capacity compared to the amidoxime group [Citation6], those resins cannot be practically used to adsorb uranium in seawater on a large scale because of the following reasons. First, since uranium exists in seawater with the concentration of only about 3 ppb, an absorbent with extremely high selectivity for uranium in seawater is required. Second, resin-type adsorbents require containers with fine meshes to keep them un-dispersed into seawater, and there will be problems with insufficient water circulation inside the containers. As summarized by Tamada [Citation4], to maintain mechanical strength of the adsorbent in seawater with unpredictable water current and wave motions, the graft copolymerization technique was employed to graft the required functional group onto surfaces of LDPE fibers, allowing the fibers to retain the mechanical strength while exhibiting high uranium adsorption capability on the surface at the same time. Among other uses in seawater, LDPE fibers are commonly used as fences to contain oil spill into seawater. Thus, at the present time, the amidoxime-grafted LDPE fibers are the most physically, mechanically and chemically suitable and practical uranium adsorbent in seawater on a large scale for several months of submerging time.
2. Experimental
2.1. Materials
Fibers used in the present study were made from pristine LDPE granules purchased from Thai Polyethylene Co., Ltd. in Thailand. An extrusion machine was used to prepare LDPE fibers from the granules. The average diameter of each fiber was about 50–200 μm. Attempts to directly use poly-acrylonitrile fibers were made, but the fibers always melted during the amidoxime preparation process. Poly-acrylonitrile fibers were purchased from Suzhou Longbang Polymer Fiber Co., Ltd. in China.
Additionally, high-density polyethylene (HDPE) was tested, as HDPE fibers are widely used in various industries and it would be convenient to locate a local manufacturer compared to LDPE fibers, which has no known use in any major industry. HDPE granules for the production of HDPE fibers were purchased from Thai Polyethylene Co., Ltd. in Thailand.
Chromic acid solution (K2Cr2O7 + H2SO4 + H2O) was prepared [Citation5]. K2Cr2O7 and H2SO4 were of analytical grade purchased from Ajax Finechem company and Qrec company, respectively. For amidoxime grafting, acrylonitrile (AN), methacrylic acid (MAA), dimethyl sulfoxide, N,N-dimethylformamide (DMF), hydroxylamine hydrochloride (NH2OH•HCl), methanol and potassium hydroxide (KOH) were used. They were of analytical grade purchased from Merck-Shuchardt and used without further purification. For the elution process of the adsorbed uranium, analytical-grade hydrochloric acid (HCl) was used, which was purchased from Merck-Shuchardt company. Deionized water (DI water) was purchased from RHK Group company in Thailand.
2.2. Synthesis
2.2.1. Chromic-acid-modified LDPE fibers
To prepare chromic-acid-modified LDPE fibers, LDPE fibers produced from the extrusion machine were immersed in the chromic acid solution at 70 °C for 2.5–90 minutes. Afterwards, the fibers were thoroughly rinsed with acetone and DI water [Citation7] and dried in a forced-convection oven at 50 °C for 24 hours.
2.2.2. Preparation of amidoxime fibers
It has been revealed from earlier studies that the extraction of uranium from seawater can be effectively achieved using fibers grafted with amidoxime functional group for their capability to displace carbonate ions in [UO2(CO3)3]4- at the pH of natural seawater. The present work follows Tamada's synthetic procedure of the adsorbent [Citation4] (except for the use of chromic acid) as summarized in .
2.2.3. Irradiation-induced graft copolymerization
The simultaneous irradiation grafting method at low temperature was employed. Low temperature was maintained by placing ice cubes in water surrounding the container for irradiation. LDPE fibers (or chromic-acid-treated LDPE fibers) were submerged in the container containing 60:40 AN:MAA solution by volume. The presence of MAA enhanced the hydrophilicity of the fibers, and this optimized mixing ratio was adopted from Kawai's work [Citation8], who studied different mixing ratios and different chemicals and concluded that MAA with this ratio was the most suitable one in terms of uranium uptake by the adsorbent. The container was irradiated with γ-ray from 60Co (Gammacell 220 Excel at Office of Atoms for Peace in Bangkok, Thailand). The dose rate was 6.21 kGy/hour and the total dose was varied from 5 to 50 kGy. After irradiation, the fibers were washed with DMF to remove residual AN and poly(acrylonitrile) homopolymer from the fiber surfaces. The cografted fibers were dried in a forced-convection oven at 50 °C for 24 hours to obtain a constant weight, and the degree of cografting was calculated from the weight gain using the following formula:
(1)
(1) where W0 and W1 are the weights of the starting and cografted fibers, respectively. Then, the cografted fibers were investigated for the characteristic band of the cyano group by Fourier transform infrared (FTIR) spectroscopy technique.
2.2.4. Amidoximation of cyano group
The grafted cyano group on the fibers was converted into the amidoxime group by soaking the fibers in 3 (w/v)% NH2OH•HCl solution (50/50 (v/v)% water/methanol and neutralized to pH of 7 by adding KOH solution) at 80 °C for 15–120 minutes. After the reaction, the fibers were rinsed with a methanol/water solution and immersed in 1 M HCl. Then, the fibers were rinsed repeatedly with DI water and dried in a forced-convection oven at 50 °C for 24 hours to obtain a constant weight. The amidoxime group density was calculated using the following formula:
(2)
(2) where W2 is the weight of the amidoxime fibers. The number 69.5 in the denominator of Equation (2) represents the molecular weight of NH2OH•HCl [Citation9]. The prepared fibers were investigated for the characteristic band of the amidoxime group using FTIR technique. Finally, the fibers were immersed in 2.5 (w/v)% KOH solution for 1 hour at 80 °C [Citation10–12]. Prior to uranium adsorption experiments, the fibers were repeatedly washed with DI water [Citation8,Citation13].
2.3. Uranium concentrations in Thailand's seawater at various locations and depths
Despite the well-known fact that uranium concentration in seawater appears to be constant in almost all areas of the oceans and their depths [Citation2 Citation3], there is no publicly available data on uranium concentration in Thailand's seawater. Therefore, in the future, if an enterprise wishes to perform uranium extraction from Thailand's seawater on a commercial scale, the existence of uranium ions in the seawater must be confirmed. Seawater samples were collected at various locations and depths in the Gulf of Thailand and the Andaman Sea. The collection locations are presented in . For surface water sample collection, a simple plastic container was submerged into water just enough so that the opening was underneath the water surface. For water samples collected at 30 m and deeper, a commercial 12-L Niskin water sampler was used. Collected samples were sent to JFE Techno-Research Corporation Keihin Division in Japan for analysis of uranium concentrations using the inductively coupled plasma-mass spectrometry (ICP-MS) technique.
2.4. Extraction and elution properties of synthesized amidoxime fiber
2.4.1. Uranium extraction from Thailand's seawater
Eighteen sets of chromic-acid-treated amidoxime fibers of approximately 20 g each were submerged in shallow seawater with the depth of approximately 3–6 m in front of Phuket Marine Biological Center (PMBC) in Phuket province, Thailand. The average temperature of the seawater was 29–30 °C, and the submerging time for each three sets of the adsorbent was 1, 2, 3, 4, 6 and 8 weeks.
2.4.2. Uranium elution from amidoxime fibers
The fibers were removed from seawater, rinsed with DI water and immersed in 1 M HCl at 50 °C for 1 hour to elute adsorbed metals. The HCl volume for elution of each set of fibers was 200 mL. Elution was performed at 50 °C as more uranium would be eluted than performing at room temperature for as long as 24 hours, confirmed by our preliminary experiments as shown in . Uranium concentration in the eluent was analyzed by an inductively coupled plasma atomic emission spectrometer.
Table 3. Quantity of eluted uranium under different elution conditions.
2.4.3. Usage repeatability
For economic reasons in the future, it is important that the adsorbent can be reused several times. Thus, the ability of the adsorbent to be reused was evaluated by submerging the chromic-acid-treated amidoxime fibers in seawater sample with added 14.3 ppm of [UO2(CO3)3]4− at room temperature for 3 days. The seawater sample was collected from surface water of Tao Island, Surat Thani Province, Thailand. For every 3 days representing one cycle, the fibers were removed from seawater, the adsorbed metals were eluted using the same procedure discussed in Section 2.4.2, and prior to seawater submersion again the fibers were conditioned by immersing in 2.5 (w/v)% KOH solution for 1 hour at 80 °C and repeatedly washed with DI water. This operation was similar to that described at the end of Section 2.2.4.
3. Results and discussion
3.1. SEM analysis of fibers at various stages
Scanning electron microscope (SEM) was employed to observe the surface morphology of the fibers at various stages. (a) illustrates the surface of the produced LDPE fiber with a very smooth appearance of some particles (or part of LDPE) on the surface, as indicated by arrows. (b) shows the surface of the cografted non-chromic-acid-treated LDPE fiber. Columns of grafted chemical chains were visible in the figure. (c) displays the surface of the non-chromic-acid-treated amidoxime fiber. Columns of grafted chemical chains completely disappeared. The amidoxime functional group must have completely filled in the spaces between the columns. (d)–(h) shows the surfaces of chromic-acid-etched LDPE fiber after the etching time of 15, 20, 25, 30 and 60 minutes, respectively.
Figure 3. SEM images illustrating surface features of fibers at various stages (a) Starting LDPE fiber, (b) cografted LDPE fiber, (c) amidoxime fiber, (d) chromic-acid-etched LDPE fiber after the etching time of 15 minutes, (e) chromic-acid-etched LDPE fiber after the etching time of 20 minutes, (f) chromic-acid-etched LDPE fiber after the etching time of 25 minutes, (g) chromic-acid-etched LDPE fiber after the etching time of 30 minutes, (h) chromic-acid-etched LDPE fiber after the etching time of 60 minutes.
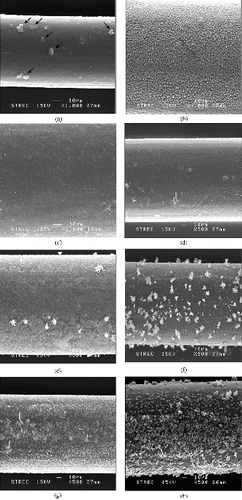
For the etching time of 15 minutes, surface roughness increased only slightly, as the fiber appeared to be almost identical to that in (a) (unetched). The slight increase in surface roughness was responsible for the small increase in the grafting efficiency. For the etching time of 20 minutes, the surface roughness became much higher, and so was the grafting efficiency. For the etching time of 25 minutes, the surface appeared quite smooth again, with a number of white needle-like crystals present. Energy dispersive X-ray spectroscopy (EDS) analysis of these crystals indicated that they were composed mainly of sulfur with a small amount of chromium, indicating some leftover chromic acid. However, etched LDPE fibers shown in (d)–(h) were prepared for the purpose of SEM observation only, so they were not thoroughly rinsed with acetone and DI water. Etched fibers that would undergo the amidoximation process would be thoroughly cleaned to ensure no leftover chromic acid. The surface smoothness was responsible for the decrease in grafting efficiency from 20 to 25 minutes of etching time. For the etching times of 30 and 60 minutes, the surfaces appeared to be rough again, but this time on a macroscopic scale. This kind of surface roughness must not be suitable for grafting of monomers, as the grafting efficiency became abruptly reduced at the etching time of 30 minutes and more, as evident in .
It is important to note that, due to the manufacturing process, the diameter of each fabricated LDPE fiber was different from one another. Although changes in the diameter of the fiber by each treatment were important, it was not possible to directly compare the fiber sizes in (a)–(h) as they were not from the same one. Nonetheless, by eye inspection, cografted fibers were larger than the original fibers, but after amidoximation, the diameter appeared to be unchanged.
3.2. Degree of cografting of AN/MAA onto LDPE fibers
Results of radiation-induced graft-copolymerization of the mixture of AN and MAA onto non-chromic-acid-treated LDPE fibers by the simultaneous irradiation grafting method are presented here. shows the degree of cografting vs. gamma-ray dose. It is evident that as the total dose increased, the degree of cografting increased. The degree of cografting became saturated at approximately 78% with 40 kGy gamma-ray dose. Therefore, the optimum gamma-ray dose of 40 kGy was selected for the amidoximation process of chromic-acid-treated fibers. In Saito's work, the maximum degree of cografting was reported to be about 70% at 40 kGy [Citation9]; therefore, the result of the present work is comparable to the literature-reported value. For HDPE fibers, the grafting efficiency reached only 45% at 40 kGy dose. This value was much lower than that of LDPE fibers; therefore, HDPE fibers were not of practical use for the present purpose. The low degree of grafting of HDPE fibers was expected, as the polymer chains of HDPE stayed closer to each other than LDPE. Thus, there is not much space between polymer chains to react to the monomers, resulting in a low grafting efficiency.
3.3. Amidoxime group density
The cyano group was converted into the amidoxime group by reaction with NH2OH•HCl solution with the reaction time varying from 15 to 120 minutes. As shown in , the amidoxime group density reached the maximum value of approximately 1.85 mol/kg after 75 minutes of reaction time. Therefore, the optimized amidoximation time of 75 minutes was selected for synthesizing chromic-acid-treated amidoxime fibers. In Saito's work, the maximum degree of cografting was reported to be about 1.8 mol/kg at 40 kGy [Citation9]; therefore, the result of the present work is comparable to the published work in literature. The trend of the result was also expected, as more reaction time resulted in more cyano groups being converted into the amidoxime group, and as after all the available cyano groups were converted, the amidoxime group density became saturated.
It is of interest to determine the theoretical maximum amidoxime group density to compare with the obtained experimental result of 1.85 mol/kg, which can be mathematically derived as follows. Assuming that the mass of LDPE fiber is 1 g, for the saturated degree of cografting of 78%, this means that the mass of 60:40 (by volume) AN:MAA solution is 0.78 g. Assuming that AN and MAA have roughly the same density, this indicates that the total mass of AN attaching to the fiber is 0.468 g and that the mass of MAA attaching to the fiber is 0.312 g. The molecular mass of AN is 53.06 g/mol, therefore, there are 8.82 mmol of AN present. This same amount of moles will become the amidoxime group after the amidoximation process. During the amidoximation process, one nitrogen atom on the cyano group is replaced by NH2OH, so the ratio of mass increase is 3.36. Thus, the mass increase on the AN part from this process is 0.468 × 3.36 = 1.57 g. Hence, the total mass of the amidoxime fiber is 1 (for the original fiber) + 1.57 (amidoximated AN) + 0.312 (MAA) = 2.88 g. Therefore, the theoretical maximum amidoxime group density for this experiment is 8.82 × 10−3/2.88 × 10−3 = 3.06 mol/kg. The experimentally obtained amidoxime group density is about 40% lower than this theoretical maximum value. This indicates that not all the available cyano groups were completely converted into the amidoxime groups. Moreover, because functional groups such as hydroxamic acid and acidic amide are formed as side reactions of the amidoximation process [Citation9], the calculated amount of amidoxime groups must have overestimated the actual value.
3.4. FTIR analysis of functional groups
Results of FTIR analysis are presented in . For the FTIR spectra of the LDPE fiber shown in (a), the characteristic absorption bands of polyethylene at 2850 and 1470 cm−1 due to C–H alkyl stretch and C–H, respectively, are clearly present. In fact, as expected, these two absorption bands are present in all figures. The absorption bands of cografted AN/MAA appear at 2250 and 1720 cm−1 due to C≡N of poly(acrylonitrile) and C = O of poly(methacrylic acid), respectively, as shown in (b). After the amidoxime group conversion, the C≡N band at 2250 cm−1 disappears, and the N–H stretch band of the amidoxime group at 3180 cm−1 as well as the C = O band of the amidoxime group at 1676 cm−1 become present, as shown in (c).
3.5. Uranium concentration in Thailand seawater
Uranium concentrations in seawater samples collected at various depths and locations in the Andaman Sea and in the Gulf of Thailand are summarized in . The analysis uncertainty of approximately 3 ppb uranium concentration level was ±1σ or ±0.5 ppb. Thus, it appears that uranium concentration in Thailand's seawater is generally uniform at about 3 ppb regardless of location and depth of seawater, consistent with the literature-reported value for other areas of the world [Citation2,3].
Table 4. Uranium concentrations in Thailand seawater.
3.6. Effect of chromic acid treatment on uranium adsorption efficiency
After different surface treatment durations, the grafting efficiency was determined from the degree of cografting as shown in and from the uranium adsorption efficiency as shown in . In , for each chromic acid treatment duration, three samples were used and the reported value was the averaged one. The standard deviations of all data points were 2.2% at most, so no error bar was included in the figure. The degree of cografting reached the maximum of about 90% at the etching time of 20 minutes, and this was as much as 30% higher than the value of about 70% reported in [Citation9].
Figure 8. Uranium uptake of chromic acid pre-treated amidoxime fibers submerged in seawater for 30 days.
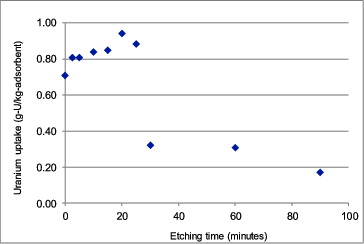
To further confirm the enhanced adsorption efficiency of chromic-acid-treated amidoxime fibers in a laboratory scale, they were submerged in seawater sample with added 500 ppb of [UO2(CO3)3]4− at room temperature for 30 days. The seawater sample was collected from surface water of Tao Island, Surat Thani Province, Thailand. From , for the etching time of 0 minute representing LDPE fiber without etching, the uranium adsorption efficiency was determined to be 0.71 g-U/kg-adsorbent. For the optimized etching time of 20 minutes, the uranium adsorption efficiency reached the maximum at 0.94 g-U/kg-adsorbent, or 32.4% higher than that of non-treated amidoxime fibers.
3.7. EDS analysis of chromic acid pre-treated amidoxime fibers
EDS is an analytical technique routinely used for elemental identification. EDS systems are mounted on SEMs and use the primary beam of the microscope to generate characteristic X-rays. The composition of the sample is determined by analyzing the energy of the characteristic X-rays.
Analyzed specimens were chromic acid pre-treated amidoxime fibers, one before submerging in seawater and the other one after submerging for 30 days. From the spectrum in , before submerging in seawater, only carbon and an abundant quantity of oxygen were present, and no uranium was detected by the instrument. From the spectrum in , after submerging in seawater, the amidoxime groups adsorbed many elements from seawater. Detected elements were magnesium, calcium and uranium. Sodium was also detected because it adhered to the adsorbent, but not extracted by the adsorbent. According to the inset, the weight percentage of the detected uranium was 2.6%. This confirms the presence and ability of amidoxime functional groups on chromic acid pre-treated fiber to adsorb uranium in seawater.
3.8. Adsorption capacity of chromic-acid-treated amidoxime fiber
Uranium adsorption capacity of amidoxime fibers prepared from chromic-acid-treated LDPE fibers submerged in shallow seawater in front of PMBC in Phuket province, Thailand, is presented in , together with results from other studies.
Figure 11. Uranium uptake of chromic acid pre-treated amidoxime fibers submerged in 30 °C Phuket seawater compared to other studies.
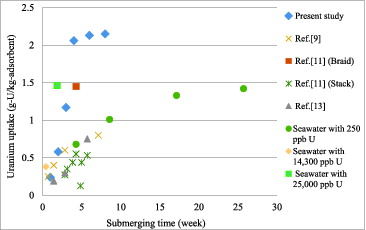
The adsorption capacities at 1, 2, 3, 4, 6 and 8 weeks were 0.24, 0.58, 1.17, 2.06, 2.13 and 2.15 g-U/kg-adsorbent, respectively. When compared with results reported by Tamada [Citation4] on the adsorption capacity of about 1.5 g-U/kg-adsorbent of the braid-type adsorbent for the soaking time of 30 days in 30 °C seawater, the present work's adsorption efficiency of 2.06 g-U/kg-adsorbent for 30 days of submerging time in 29–30 °C seawater was about 37% higher. Moreover, after approximately 2 weeks of submerging time, the adsorption capacity in the present study was clearly and substantially higher than those of other studies. This significantly enhanced adsorption was clearly attributable to the increased grafting efficiency from the surface treatment.
When comparing the result in to that in , it can be observed that submersion in open ocean for 1 month yielded the adsorption efficiency of approximately two times higher than that submerged in seawater sample with added 500 ppb of [UO2(CO3)3]4− in a small-scale laboratory set-up. Although higher uranium concentration in seawater should be considered a prime condition for uranium uptake by the amidoxime fibers, the effects of natural wave motion and continuous ocean current which introduce new seawater into the adsorbent all the time would outweigh the effect of uranium concentration, making open-ocean submersion more effective. To substantiate this claim, three more small-scale experiments were conducted as follows:
Seawater sample in a container was added with 250 ppb of [UO2(CO3)3]4− and non-chromic-acid-etched amidoxime fibers were submerged in it for up to 180 days.
Seawater sample in a container was added with 14,300 ppb of [UO2(CO3)3]4− and non-chromic-acid-etched amidoxime fibers were submerged in it for 3 days.
Seawater sample in a container was added with 25,000 ppb of [UO2(CO3)3]4− and non-chromic-acid-etched amidoxime fibers were submerged in it for 13 days.
Results of these experiments are included in . For 250 ppb of uranium concentration, the adsorption efficiency was still not as high compared to the real ocean submersion for the same soaking duration. Thus, for the small-scale laboratory test with artificially enhanced hundreds of ppb of uranium concentration in seawater sample, the effect of higher uranium concentration was not as pronounced as the effects of natural wave motion and continuous ocean current. However, for tens of thousands of ppb of uranium concentration (conditions no. 2 and 3), the extremely and artificially high uranium concentration now strongly affected the adsorption efficiency. However, this condition is not possible in real marine applications and the results should only be regarded as artificially true. Nevertheless, this opens up the possibility of a separate study for interested researchers on the effect of uranium concentration in seawater sample on uranium uptake by amidoxime fibers.
From the result in , it is also important to note that uranium uptake took place mostly within the first month of submersion. Afterward, the adsorbent appeared to be almost saturated, as continued submersion from 1 month to 2 months increased the adsorption by only about 4%.
3.9. Usage repeatability
The adsorption efficiency after each 3-day cycle for up to eight cycles is demonstrated in .
From the figure, a linear downward trend is observed. This was because every time the adsorbent underwent the elution process, some amidoxime sites were damaged. More elution cycles resulted in more amidoxime sites being destroyed. In terms of economics, it may be more favorable to continue using low-efficiency fibers than to spend resources to synthesize new ones. However, fibers with too low efficiency may make the extraction process unattractive economically. Thus, the economics of the process will determine the minimum efficiency that the fibers should have, and data in only serve to confirm the hypothesis that more elution cycles resulted in less adsorption efficiency of the amidoxime fibers. The extrapolated linear best-fit trend line shown in the figure predicts that the adsorption capacity will reduce to about 50% after 11 cycles of usage.
However, in Saito's work [Citation9], the 5-day period of contact with natural seawater followed by an elution period of 1 hour using 1 M HCl (temperature not indicated) and the subsequent KOH treatment, the adsorption capacity remained relatively unchanged even after 10 cycles. The underlying mechanism for the degradation of the amidoxime fiber of the present study needs to be investigated, but it is beyond the scope of the present study. However, it is possible that elution with 1 M HCl at 50 °C accelerated the degradation (assuming that Saito performed HCl elution at room temperature).
4. Conclusion
Enhancement of uranium extraction from seawater using chromic-acid-treated amidoxime adsorbent was studied. Chromic-acid-treated amidoxime fibers were synthesized based on the simultaneous irradiation grafting method at low temperatures. The maximum grafting efficiency of about 90% occurred at 20 minutes of acid treatment time. When submerged in open seawater with the average temperature of 30 °C for up to 8 weeks, the adsorption capacity of the fibers was evaluated to be as high as 2.15 g-U/kg-adsorbent. The significantly enhanced adsorption was due to the increased grafting efficiency from the surface treatment which increased the surface area of fibers.
The ability of the adsorbent to be reused was evaluated. More elution cycles resulted in less adsorption efficiency of the amidoxime fibers. The adsorption capacity was evaluated and predicted to reduce to about 50% after 11 cycles of repeated usage.
Uranium concentrations in seawater samples collected at surface, 30-m 100-m and 150-m depths at various locations in the Andaman Sea and in the Gulf of Thailand were analyzed using the ICP-MS technique to be about 3 ± 0.5 ppb, regardless of the location and depth of seawater. This result is consistent with the literature-reported values for other areas of the world.
Acknowledgements
The authors would like to thank the Electricity Generating Authority of Thailand (EGAT), the Ministry of Science and Technology (MOST) and the 90th Anniversary of Chulalongkorn University Fund (Ratchadaphiseksomphot Endowment Fund) for the funding support of this research work. The authors would like to thank Assoc. Prof. Chyagrit Siri-Upathum for the starting idea to conduct research on uranium extraction from seawater in Thailand and for recommendations on various aspects of the experiments. The authors appreciate Dr. Masao Tamada for the recommendation of JFE Techno-Research Corporation Keihin Division in Japan for analysis of uranium concentrations in seawater samples. The authors would like to thank Faculty of Science and Technology, Rajamangala University of Technology Tawan-Ok for the equipment support. Finally, the authors would like to thank Office of Atoms for Peace (OAP) and Thailand Institute of Nuclear Technology (TINT) for gamma-ray irradiation during adsorbent synthesis.
References
- Schwochau K. Inorganic Chemistry. Heidelberg: Springer; 1984. Chapter 3, Extraction of Metals from Sea Water; p. 91–133.
- Ku T, Knauss K, Mathieu G. Uranium in open ocean: concentration and isotopic composition. Deep Sea Res. 1997;24:1005–1017.
- Henderson G, Anderson R. The U-series toolbox for paleoceanography. Rev Mineral Geochem. 2003;52:493–531.
- Tamada M. Current status of technology for collection of uranium from seawater. Paper presented at: International Seminar on Nuclear War and Planetary Emergencies - 42nd Session; 2009; Aug 19–24; Erice, Italy.
- Wang H, Chen S, Zhang J. Surface treatment of LLDPE and LDPE blends by nitric acid, sulfuric acid, and chromic acid etching. Colloid Polym Sci. 2009;287:541–548.
- Sabarudin A, Oshima M, Takayanagi T, Hakim L, Oshita K, Gao Y, Motomizu S. Functionalization of chitosan with 3,4-dihydroxybenzoic acid for the adsorption/collection of uranium in water samples and its determination by inductively coupled plasma-mass spectrometry. Analytica Chimica Acta. 2007;581:214–220.
- Wan Y, Luo H, Wang Y, Raman S, Huang Y, Zhang T, Liu H. Characterization of three-dimensional braided polyethylene fiber–PMMA composites and influence of fiber surface treatment. J Appl Polym Sci. 2006;99:949–956.
- Kawai T, Saito K, Sugita K, Kawakami T, Kanno J, Katakai A, Seko N, Sugo T. Preparation of hydrophilic amidoxime fibers by cografting AN and MAA from an optimized monomer composition. Radiat Phys Chem. 2000;59:405–411.
- Saito K, Hori T, Furusaki S, Sugo T, Okamoto J. Porous amidoxime-group-containing membrane for the recovery of uranium from seawater. Ind Eng Chem Res. 1987;26:149–153.
- Zhang A, Asakura T, Uchiyama G. The adsorption mechanism of uranium(VI) from seawater on a macroporous fibrous polymeric adsorbent containing amidoxime chelating functional group. React Funct Polym. 2003;57:67–76.
- Seko N, Tamada M, Yoshii F. Current status of adsorbent for metal ions with radiation grafting and crosslinking techniques.Nucl Instrum Methods Phys Res B. 2005;236:21–29.
- Zhang A, Uchiyama G, Asakura T. pH effect on the uranium adsorption from seawater by a macroporous fibrous polymeric material containing amidoxime chelating functional group. React Funct Polym. 2005;63:143–153.
- Kawai T, Saito K, Sugita K, Katakai A, Seko N, Sugo T, Kanno J, Kawakami T. Comparison of amidoxime adsorbents prepared by cografting MAA and 2-hydroxyethyl methacrylate with AN onto polyethylene. Ind Eng Chem Res. 2000;39:2910–2915.