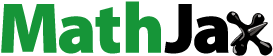
Abstract
Sample reactivity experiments on the uncertainty analyses of Pb nuclear data are carried out by substituting Al plates for Pb ones at the Kyoto University Critical Assembly, as part of basic research on Pb–Bi for the coolant. Numerical simulations of sample reactivity experiments are performed with the Monte Carlo calculation code MCNP6.1 together with four nuclear data libraries JENDL-3.3, JENDL-4.0, ENDF/B-VII.0 and JEFF-3.1, to examine the accuracy of cross-section uncertainties of Pb isotopes by comparing measured and calculated sample reactivities. A library update from JENDL-3.3 to JENDL-4.0 is demonstrated by the fact that the difference between Pb isotopes of the two JENDL libraries is dominant in the comparative study, through the experimental analyses of sample reactivity by the MCNP approach. In addition, JENDL-4.0 reveals a slight difference from ENDF/B-VII.0 in all Pb isotopes and 27Al, and from JEFF-3.1 in 238U and 27Al. Based on these results, further experiments are needed to investigate the uncertainties of Bi isotopes with the use of the Pb–Bi and Bi plates.
1. Introduction
The accelerator-driven system (ADS) has been considered an attractive candidate for solutions to achieve nuclear transmutation of minor actinides (MAs) and long-lived fission products (LLFPs) with the use of fast neutrons obtained by the injection of high-energy protons onto a heavy metal target. As part of ADS basic research at the Kyoto University Critical Assembly (KUCA), a series of reactor physics experiments on ADS [Citation1–4] has been carried out by coupling with the KUCA core and the fixed-field alternating gradient (FFAG) accelerator [Citation5,Citation6]. The ADS experimental facilities are being prepared for the investigations of nuclear transmutation of MA and LLFP, as are the Transmutation Experimental Facility (TEF) [Citation7–9] at the Japan Atomic Energy Agency (JAEA) and the Multi-purpose hYbrid Research Reactor for High-tech Applications (MYRRHA) [Citation10–12] at SCK•CEN. In the TEF and MYRRHA facilities, liquid lead–bismuth (Pb–Bi) has been selected as not only material for the target to generate spallation neutrons but also material for the coolant in fast neutron spectrum cores.
Attempts were made to investigate the neutron characteristics of lead–bismuth eutectic (LBE) from the aspect of reactor physics and nuclear data through experimental and numerical analyses. Among these attempts, at the critical assembly BFS-61 for the lead-cooled fast reactor (LFR) [Citation13], experimental benchmarks on plutonium-fueled and Pb-cooled cores were analyzed with the combined use of Monte Carlo calculations and two nuclear data libraries [Citation14] (ENDF/B-VI and JENDL-3.2). From a series of the results in LFR analyses, a considerable difference between the two libraries was briefly discussed in the estimation of cross-sections for the inelastic moderation process of Pb, by analyzing the Pb void effect of the coolant. Second, due to the revaluation of Pb inelastic scattering reactions in the JENDL-4.0 benchmarks [Citation15], criticality by the deterministic approach resulted remarkably well in about 30% of the C/E (calculation/experiment) values by comparing JENDL-3.3 [Citation16] and JENDL-4.0 [Citation17] libraries, and analyzing SEG (Scientific Ecology Group, Plant, ORNL) facility data. Third, for the numerical analyses [Citation18] of the JAEA ADS model [Citation7–9], in addition to the uncertainties of 241Am cross-sections, LBE void reactivity was considered particularly sensitive to small changes in the inelastic scattering cross-sections of 206Pb and 207Pb, by comparing JENDL-3.3 and JENDL-4.0 libraries.
Cross-section uncertainties of Pb isotopes could consequently affect to the precision of nuclear design calculations of preliminary analyses, before the actual operation of upcoming ADS, since Pb is composed partly of coolant material (LBE) in ADS facilities. Experimental studies were then requisite to determine the main reasons for cross-section uncertainties of Pb isotopes mentioned in previous studies [Citation13,Citation15,Citation18]. As a suitable way of investigating of cross-section uncertainties, sample reactivity measurements in critical states were considered effective with the use of reference and test materials in a zero-power state, such as a critical assembly, because integral parameter information on cross-sections of test materials can be acquired experimentally. In addition, numerical analyses of sample reactivity by the Monte Carlo approach can be advantageous from the viewpoint of high-precision integral parameters. For the required experimental study on Pb nuclear data uncertainties, the sample reactivity experiments were carried out at the KUCA core by the substitution of reference (Al) for test (Pb) materials, and numerical simulations were performed with the Monte Carlo calculation code MCNP6.1 [Citation19] together with nuclear data libraries JENDL-3.3, JENDL-4.0, ENDF/B-VII.0 [Citation20] and JEFF-3.1 [Citation21].
The main objective of this study was to conduct comparative study on the accuracy of cross-sections of the Pb isotopes, with a focus on the differences between JENDL-4.0 and the three other libraries, through a comparison between measured and calculated sample reactivities. The details of sample reactivity experiments and evaluation methods are described in Section 2. The results of numerical analyses by MCNP6.1 with nuclear data libraries are presented in Section 3 and the conclusions are summarized in Section 4.
2. Sample reactivity experiments
2.1. Core configuration
Of three cores designated A, B and C at KUCA, A and B are polyethylene solid-moderated and -reflected cores, and C is a light water-moderated and water-reflected one. The three cores are operated at a low mW power in the normal operating state. The sample reactivity experiments were carried out in the A-core () that has polyethylene moderator (current polyethylene “p” in ) and reflector (conventional polyethylene) rods ((a)), and four different fuel assemblies: normal “F,” partials “40” and “14,” and reference “f” fuel assemblies ((a), (b), (c) and (d), respectively). Normal fuel assembly “F” is composed of 60 unit cells, and upper and lower polyethylene blocks about 560 and 540 mm long, respectively, in an aluminum (Al) sheath (54×54×1,520 mm; b)). For the normal and partial fuel assemblies, a unit cell in the fuel region is composed of a highly-enriched uranium (HEU; c)) fuel plate 1/16″ thick and polyethylene (current polyethylene) plate 1/8″ thick. The numerals 40 and 14 correspond to the number of fuel plates in the partial fuel assembly used for reaching the criticality mass. The reference fuel assembly “f” is composed of 40 unit cells with an HEU fuel plate 1/16″ thick and Al plate 1/16″ thick, 20 unit cells of HEU and the polyethylene plate as in the normal fuel assembly, as shown in d).
Table 1. Atomic densities.
2.2. Experimental settings
In the sample reactivity experiments, a test-zoned fuel region was arranged for measuring the effects of substituting Al plates for Pb ones (d)) upon the criticality. In the test zone, five test fuel assemblies were set around the core at positions (14, O), (15, M), (15, O), (16, O) and (15, L), as shown in . The patterns of sample reactivity experiments were ranging between three and five, as shown in , substituting the reference fuel rods for Pb fuel rods. The test fuel rod was the same as in the reference fuel rod substituting Al plates for Pb ones shown in . The spectrum of experimental core at KUCA was compared with that of LBE core [Citation8] in the JAEA ADS model, as shown in . The experimental core was a relatively hard spectrum one implemented in KUCA, though not to a fast spectrum core in actual ADS. The substitution was conducted in a total of 40 unit cells of the central region of fuel rods, such as changing Al plates in d) into Pb ones in . The sample reactivity caused by the substitution was experimentally obtained through the difference between the excess reactivities of Al reference core () and Pb test core (). In the experiments, the critical state was adjusted by maintaining the control rods (C1, C2 and C3: and e)) in certain positions shown in ; the excess reactivity was then deduced by the difference between the critical and super-critical states in the core. The experimental excess reactivity was obtained with the combined use of both the reactivity worth of each control rod evaluated by the rod drop method and its integral calibration curve obtained by the positive period method. The estimated experimental error of excess reactivity measurement was less than 5%. In the Al reference and Pb test cores, the effective delayed neutron fraction (βeff) was acquired by MCNP6.1 (2,000 active cycles of 50,000 histories; 2 pcm statistical error) with JENDL-4.0, and the values of 798 and 801 pcm were applied to these two cores, respectively, when the excess reactivity in dollar units was converted into that in pcm units.
Figure 4. Schematic drawing of test fuel rod “Pb” containing Pb plates (1/8″p10EUEU < 1/8″PbBi40EUEU > 1/8″p10EUEU).
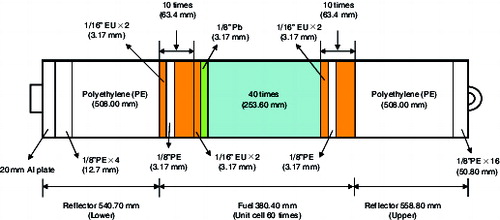
Figure 5. Comparison between the neutron spectra of HEU-Pb test zone in KUCA and LBE-cooled core in JAEA ADS model.
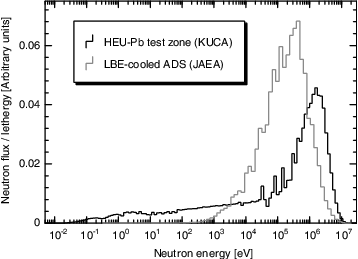
Table 2. Control rod positions in reference and test cores (Cases 1 through 4).
2.3. Evaluation method
Experimental sample reactivity ΔρExpAl → Pb was deduced by the difference between two excess reactivities ΔρExp, AlExcess and ΔρExp, PbExcess obtained by the positive period method in the reference and test cores, respectively, as follows, when the Al plates were substituted for the Pb ones:
(1)
(1) where kExp, AlClean and kExp, PbClean indicate the effective multiplication factors deduced by the experimental excess reactivities obtained in super-critical cores (clean core) before (Al) and after (Pb) substituting Al plates for Pb ones, respectively, under the condition of all the control and safety rods withdrawn.
In the MCNP analyses, numerical sample reactivity ΔρMCNPAl → Pb was deduced by the difference between two excess reactivities ΔρMCNP, AlExcess and ΔρMCNP, PbExcess in the reference and test cores, respectively, as follows, with the same method as that of experimental sample reactivity:
(2)
(2) where kMCNP, AlClean and kMCNP, PbClean indicate the effective multiplication factors in super-critical cores before and after substituting Al plates for Pb ones, respectively. In addition, kMCNP, AlCritical and kMCNP, PbCritical need to be defined as the values of the effective multiplication factors in critical cores before and after substituting Al plates for Pb ones, since these numerical values always are not units.
On the basis of the experimental methodology shown in EquationEquation (1)(1)
(1) , the numerical approach of sample reactivity ΔρCalAl → Pb can be generally expressed as follows, in case of substituting Al plates for Pb ones:
(3)
(3) where kCal, AlClean and kCal, PbClean indicate the effective multiplication factors in super-critical cores.
Numerical sample reactivity ΔρMCNPAl → Pb in EquationEquation (2)(2)
(2) can be rewritten with the use of the concept of EquationEquation (3)
(3)
(3) as follows:
(4)
(4) where ΔMCNPCritical, Al → Pb indicates the difference between inverse values of eigenvalue calculations in the two critical states evaluated by MCNP6.1. Here, the first term in EquationEquation (4)
(4)
(4) is defined as “criticality bias” as follows:
(5)
(5)
By introducing the evaluation methodology of the numerical sample reactivity shown in EquationEquation (3)(3)
(3) , ΔMCNP, Al → PbClean, J40 → xxx, yy − zzz is investigated on the numerical sample reactivity as follows, when the nuclear data libraries and isotopes are varied in the MCNP calculations:
(6)
(6) where J40 indicates the JENDL-4.0 library, All all the related isotopes and xxx a suitable choice of three nuclear data libraries: JENDL-3.3, ENDF/B-VII.0 and JEFF-3.1, yy an isotope and zzz a mass of isotopes.
3. Results and discussion
3.1. Numerical simulations
The numerical analyses were conducted with the use of the Monte Carlo code MCNP6.1 together with the JENDL-3.3, JENDL-4.0, ENDF/B-VII.0 and JEFF-3.1 nuclear data libraries for transport. For actual experimental analyses, the capability of eigenvalue calculations by MCNP6.1 was useful to be discussed with the use of JENDL-4.0 in processing important data analyses. Also, JENDL-4.0, as a reference library, was compared with the other nuclear data libraries to reveal its uncertainty.
In the reference core shown in , criticality was reached by adjusting the position of control rod C2 and withdrawing control rods C1 and C3, and safety rods S4, S5 and S6 from the core, and excess reactivity was deduced with the combined use of control rod worth (C2) by the rod drop method and its calibration curve by the positive period method. The measured excess reactivity was within an uncertainty of 5%, and compared with the numerical one as shown in . The numerical excess reactivity was obtained by the MCNP6.1 eigenvalue calculations with JENDL-4.0 within a statistical error of 2 pcm through 2,000 active cycles of 25,000 histories and estimated with the use of the two eigenvalue calculations in critical and super-critical states. From a comparison between measured and calculated results shown in , the C/E (calculation/experiment) value revealed good agreement with a relative difference of 7%.
Table 3. Comparison between the results of measured and calculated excess reactivities in reference core, as shown in
Furthermore, in the reference core, the measured control rod worth was compared with calculated one, using the same method as for the excess reactivity. The results are as shown in . The MCNP eigenvalue calculations reproduced the experimental results of control rod worth accurately, with the C/E values within 2%, ranging between 350 and 1000 pcm, regardless of the kind of control rod used ().
Table 4. Comparison between the results of measured and calculated control rod worth in reference core, as shown in
3.2. Eigenvalue bias
On the basis of EquationEquation (2)(2)
(2) , numerical analyses of sample reactivity were conducted by the MCNP6.1 eigenvalue calculations with nuclear data libraries as in Section 3.1. In numerical simulations, sample reactivity was obtained by the two eigenvalue calculations in both critical and super-critical states: the difference between the inverse values of eigenvalue calculations in the two states. The calculated results of the sample reactivity were obtained by varying the nuclear data libraries, as shown in (comparison between EquationEquations (1)
(1)
(1) and (Equation2
(2)
(2) )), and, through an estimation of C/E values, were compared at a high-accuracy with the experimental results in almost all cases, regardless of the kind of nuclear data libraries used. From the calculated results in through , the precision of MCNP6.1 with JENDL-4.0 was considered fairly good in the eigenvalue calculations, and JENDL-4.0 was found reliable as a reference nuclear data library, comparing it with JENDL-3.3.
Table 5. Comparison between the results of measured and calculated sample reactivities evaluated by EquationEquations (1)(1)
(1) and (Equation2
(2)
(2) ), respectively, by substituting Al plates for Pb ones, as shown in
Prior to the MCNP numerical analyses, in the experiment results, interesting discussions were provided from two aspects. First, the positive reactivity effect was found in the sample reactivity experiments substituting Al plates for Pb ones, and was mainly attributable to the difference between the values of moderating ratio (ξΣs / Σa: ξ, Σs and Σa indicate the average logarithmic energy decrement, macro cross-sections of scattering and absorption, respectively.) in Al and Pb. Second, while the number of substitution of fuel rods was the same as in both Cases 1 and 2, a significant difference between sample reactivities was involved in the forward and adjoint functions of reactivity defined in the First-order perturbation theory with the variation of core sizes in horizontal and vertical directions shown in
3.3. Criticality bias
As discussed in Section 3.2, the ability of MCNP6.1 calculations was confirmed in terms of the general definition of sample reactivity by the MCNP approach. Here, the main objective of this study was to compare the experimental and numerical sample reactivities defined in EquationEquations (1)(1)
(1) and (Equation3
(3)
(3) ), respectively. By comparing EquationEquations (1)
(1)
(1) and (Equation3
(3)
(3) ), as shown in , considering the uncertainties of C/E values, the accuracy of the numerical analyses by MCNP6.1 with JENDL-4.0 demonstrated a relative difference of about 5% and an overestimation by more than 50% with JENDL-3.3. By comparing JENDL-3.3 and JENDL-4.0, the calculated values with JENDL-4.0 improved more with a high-accuracy of 30% in the C/E values than with the values calculated with JENDL-3.3. Regarding libraries ENDF/B-VII.0 and JEFF-3.1, the calculated sample reactivities were considered well within the relative difference of 10% as shown in
Table 6. Comparison between the results of measured and calculated sample reactivities evaluated by EquationEquations (1)(1)
(1) and (Equation3
(3)
(3) ), respectively, by substituting Al plates for Pb ones, as shown in
Mention should be made, however, of the accuracy of the numerical analyses obtained by MCNP with the four libraries, especially the absolute values by JENDL-4.0, as shown in . Further investigation was needed to find the reason for the discrepancy of unit C/E values with JENDL-4.0. Consequently, in addition to the concept of eigenvalue bias mentioned in Section 3.2, a different evaluation, here termed “criticality bias,” of sample reactivity was introduced to investigate C/E discrepancy, when the formulation of sample reactivity by MCNP6.1 defined in EquationEquation (2)(2)
(2) is changed to that in EquationEquation (4)
(4)
(4) . As shown in EquationEquation (5)
(5)
(5) , the criticality bias ΔMCNPCritical, Al → Pb by the MCNP approach was obtained by the difference between reactivity-like criticalities in critical cores by substituting of Al plates for Pb ones, and interpreted as a bias of reactivity induced by the difference between the experiments and the eigenvalue calculations. By the introduction of criticality bias in EquationEquation (5)
(5)
(5) , a small discrepancy in C/E values () was found in the numerical simulations.
On the basis of EquationEquation (5)(5)
(5) , criticality bias ΔMCNPCritical, Al → Pb was compared with each nuclear data library, as shown in and . JENDL-4.0 revealed the bias around 20 pcm; JENDL-3.3 a further bias ranging between 50 and 100 pcm; ENDF/B-VII.0 a relatively small bias less than 20 pcm, compared with the JENDL libraries. Among the four libraries, JEFF-3.1 compared favorably with a small bias around 10 pcm, and resulted in a markedly high accuracy of C/E values, as shown in
Figure 6. Comparison between the values of criticality bias in critical states evaluated by EquationEquation (5)(5)
(5) .
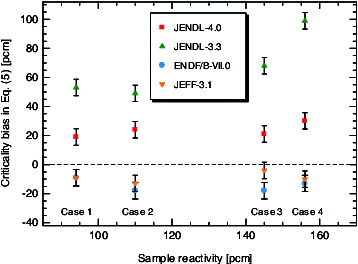
Table 7. Comparison between the results of criticality bias by four nuclear data libraries corresponding to
3.4. Discussion
Special attention was paid to the second term in EquationEquation (4)(4)
(4) to investigate the difference between JENDL libraries mentioned in Section 3.3. The term was significantly demonstrated in actual sample reactivity by the MCNP analyses, as well as by experiments, and bias between JENDL-4.0 and the other libraries was studied with a new definition, as shown in EquationEquation (6)
(6)
(6) : contribution of individual isotope to sample reactivity. In the analyses of differences defined in EquationEquation (6)
(6)
(6) , JENDL-4.0 was selected as the reference library, and the sample reactivities in clean cores were obtained, as shown in (a) through 7(d) along with four cases in , respectively, when the libraries and isotopes were varied separately: core composition materials of Pb isotopes, 27Al, 235U and 238U in the fuel rod of the core.
Figure 7. Comparison between the values of difference in EquationEquation (6)(6)
(6) in JENDL-4.0 and other nuclear data libraries: (a) Case 1, (b) Case 2, (c) Case 3, and (d) Case 4.
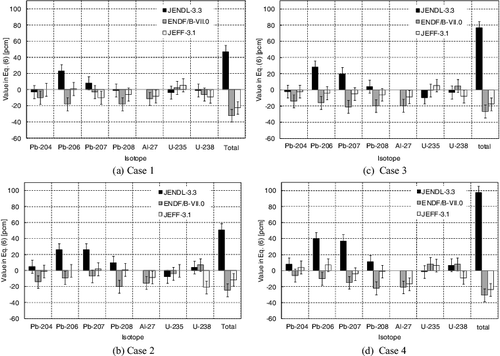
A comparison between the two JENDL libraries showed a significant effect on the reactivity resulting from large differences among all Pb isotopes (204Pb, 206Pb, 207Pb and 208Pb), regardless of the magnitude of sample reactivity: especially from those of 206Pb and 207Pb; contrary to that among the others (27Al, 235U and 238U). Regarding the discussion between the two JENDL libraries, the reason for total difference was attributable mainly to those of all Pb isotopes through the analyses of differences in EquationEquation (6)(6)
(6) . As discussed in previous studies [Citation13,Citation15], this fact provided valuable knowledge that an improvement of the inelastic scattering cross-sections around a few MeV neutron energy region of 206Pb and 207Pb had been pointed out importantly in the difference between JENDL-3.3 and JENDL-4.0 libraries through the analyses of the Pb void reactivity in the JAEA ADS model [Citation18] and of the Pb reflector effect on SEG experiments through JENDL-4.0 benchmarks [Citation15]. From the results of ENDF/B-VII.0, a small effect of the difference compared inversely with that in JENDL-4.0 about 20 pcm in all cases, with regard to Pb isotopes and 27Al, but not to 235U and 238U, although the total difference between JENDL-4.0 and ENDF/B-VII.0 was slight. Furthermore, while a difference about 20 pcm was found in 238U and 27Al of Cases 2 and 4, respectively, the difference between JENDL-4.0 and JEFF-3.1 was considered notably minor within the allowance of relative errors.
On the basis of these observations, a library update from JENDL-3.3 to JENDL-4.0 was demonstrated by the fact that the difference between Pb isotopes of the two JENDL libraries was dominant in the comparative study, through the numerical analyses of sample reactivity by the MCNP approach. Moreover, JENDL-4.0 revealed a slight difference from ENDF/B-VII.0 in all the Pb isotopes and 27 Al, and from JEFF-3.1 in 238U and 27Al.
4. Conclusions
The sample reactivity experiments were carried out at KUCA to examine the uncertainties of cross-sections of Pb and other isotopes. The comparison between the experiments and the calculations by MCNP6.1 with JENDL-3.3, JENDL-4.0, ENDF/B-VII.0 and JEFF-3.1 libraries revealed as follows: The library update from JENDL-3.3 to JENDL-4.0 demonstrated that the difference between Pb isotopes was dominant in the comparative study, through the experimental analyses of sample reactivity by the MCNP approach. Moreover, JENDL-4.0 revealed a slight difference from ENDF/B-VII.0 in all the Pb isotopes and 27 Al, and from JEFF-3.1 in 238U and 27Al.
These results indicated that further sample reactivity experiments are needed for experimental analyses as follows: sensitivity analyses of reactions and energy of target isotopes in nuclear data libraries. Also, the same analyses can be applied to sample reactivity experiments by a substitution of Al plates for Pb–Bi or Bi ones for investigating uncertainties of Bi isotopes.
Acknowledgements
This study is supported by “Research and Development for Engineering Resolution of Nuclear Transmutation Cycle by Accelerator-Driven System” [grant number 131101] under the Innovative Nuclear Research and Development Program by the Ministry of Education, Culture, Sports, Science and Technology of Japan. The authors are grateful to all the technical staff at KUCA for their assistance during the experiments.
Disclosure statement
No potential conflict of interest was reported by the authors.
References
- Pyeon CH, Misawa T, Lim JY, et al. First injection of spallation neutrons generated by high-energy protons into the Kyoto University Critical Assembly. J Nucl Sci Technol. 2009;46:1091–1093.
- Lim JY, Pyeon CH, Yagi T, et al. Subcritical multiplication parameters of the accelerator-driven system with 100 MeV protons at the Kyoto University Critical Assembly. Sci Technol Nucl Install. 2012;395878:9.
- Pyeon CH, Azuma T, Takemoto Y, et al. Experimental analyses of external neutron source generated by 100 MeV protons at the Kyoto University Critical Assembly. Nucl Eng Technol. 2013;45:81–88.
- Pyeon CH, Nakano H, Yamanaka M, et al. Neutron characteristics of solid targets in accelerator-driven system with 100 MeV protons at Kyoto University Critical Assembly. Nucl Technol. 2015. (in press).
- Lagrange JB, Planche T, Yamakawa E, et al. Straight scaling FFAG beam line. Nucl Instrum Methods A. 2013;691:55–63.
- Yamakawa E, Uesugi T, Lagrange JB, et al. Serpentine acceleration in zero-chromatic FFAG accelerators. Nucl Instrum Methods A. 2013;716:46–53.
- Tsujimoto K, Sasa T, Nishihara K, et al. Neutronics design for lead-bismuth cooled accelerator-driven system for transmutation of minor actinide. J Nucl Sci Technol. 2004;41:21–36.
- Nishihara K, Iwamoto H, Tsujimoto K, et al. Neutronics design of accelerator-driven system for power flattening and beam current reduction. J Nucl Sci Technol. 2008;45:812–822.
- Sugawara T, Nishihara K, Tsujimoto K, et al. Analytical validation of uncertainty in reactor physics parameters for nuclear transmutation systems. J Nucl Sci Technol. 2010;47:521–530.
- Abderrahim HA, D'hondt P. MYRRAHA: a European experimental ADS for R&D applications status at mid-2005 and prospective towards implementation. J Nucl Sci Technol. 2007;44:491–498.
- Mansani L, Artioli C, Schikorr M, et al. The European lead-cooled EFIT plant: an industrial-scale accelerator-driven system for minor actinide transmutation. Nucl Technol. 2012;180:241–263.
- Rimpault G, Darde P, Mellier F, et al. The issue of accelerator beam trips for efficient ADS operation. Nucl Technol. 2013;184:249–260.
- Tsiboulia A, Khomyakov Y, Koscheev V, et al. Validation of nuclear data for Pb and Bi using critical experiments. J Nucl Sci Technol. 2002;suppl. 2:1010–1012.
- Lone MA, Muir DW. Report of the IAEA nuclear data section to the international nuclear data committee for the period January–December 1999. IAEA, INDC (NDS)-414; 2000.
- Chiba G, Okumura K, Sugino K, et al. JENDL-4.0 benchmarking for fission reactor applications. J Nucl Sci Technol. 2011;48:172–187.
- Shibata K, Kawano T, Nakagawa T, et al. Japanese evaluated nuclear data library version 3 revision-3: JENDL-3.3. J Nucl Sci Technol. 2002;39:1125–1136.
- Shibata K, Iwamoto O, Nakagawa T, et al. JENDL-4.0: A new library for nuclear science and technology. J Nucl Sci Technol. 2011;48:1–30.
- Iwamoto H, Nishihara K, Sugawara T, et al. Sensitivity and uncertainty analysis for an accelerator-driven system with JENDL-4.0. J Nucl Sci Technol. 2013;50:856–862.
- Goorley JT, James MR, Booth TE, et al. Initial MCNP6 release overview – MCNP6 version 1.0. LANL, LA-UR-13-22934; 2013.
- Chadwick MB, Oblozinsky P, Herman M, et al. ENDF/V-II.0: next generation evaluated nuclear data library for nuclear science and technology. Nucl Data Sheet. 2006;107:2931–3060.
- Koning A, Forrest R, Kellett M, et al. The JEFF-3.1 nuclear data library – JEFF report 21. OECD/NEA, No. 6190; 2006.