ABSTRACT
Titanium dioxide (TiO2) treatment coupled with ultraviolet irradiation was selected as a corrosion mitigation technique for Type 304 stainless steel (SS) in high-temperature pure water with the presence of hydrogen peroxide (H2O2). Type 304 SS specimens were pre-oxidized in oxygenated pure water at 288 °C and then coated with TiO2 nanoparticles by hydrothermal deposition. Electrochemical polarization analyses were conducted to investigate the corrosion behavior of both TiO2-treated and pre-oxidized specimens in 288 °C pure water with 300 ppb H2O2. Ultraviolet (UV) irradiation was then imposed upon the TiO2-treated specimens to examine if there was any distinct photoelectric effect on the corrosion behavior of the treated samples. It was found that the electrochemical corrosion potentials of the TiO2 treated specimens under UV irradiation were 10–20 mV lower than those without UV. In addition, the corrosion current densities of the treated specimens were also lower in the presence of UV radiation. Without UV radiation, however, no significant differences were observed between the TiO2 treated and untreated specimens. These results indicate that the TiO2 treatment in combination with UV radiation would reduce the corrosion rate of Type 304 SS in H2O2-rich, high-temperature pure water.
1. Introduction
Intergranular stress corrosion cracking (IGSCC) on stainless steel (SS) and nickel-base alloy components has been a major material degradation issue for decades for all boiling water reactors (BWRs) around the world. The IGSCC susceptibility of structural materials in BWRs has been well known to influence by the electrochemical corrosion potential (ECP), which is mainly determined by the concentrations of all redox species (i.e. H2, O2, and H2O2) in the reactor coolant. Utilities around the world have adopted the technology of hydrogen water chemistry (HWC) in BWRs for mitigating the problem of IGSCC in the structural components. The HWC technology is deemed effective when the ECP in the primary coolant circuit of a BWR at a specific feedwater hydrogen concentration ([H2]FW) is reduced to below −0.23 VSHE that signifies immunity of structural components to IGSCC [Citation1]. Due to the adverse effects of HWC in elevating the operational and shutdown dose rates in a BWR, novel technologies such as catalytic coatings [Citation2,Citation3] and inhibitive coatings [Citation4,Citation5] have been developed to enhance the effectiveness of HWC in lowering hydrogen consumption and expanding protection areas.
To further maximize the efficiency of coatings, a technique involving deliberate photoexcitation on coating materials has also been studied [Citation6–9]. Among the potential coating materials, titanium dioxide (TiO2) is an n-type semiconductor that can be excited by photon irradiation to generate photoelectric currents. TiO2 has a characteristic to generate electron-hole pairs in its space charge layer under UV irradiation. The excited electrons in the conduction band are then transferred to the coated metal surface, leading to decreases in both the corrosion potential and the oxidation reaction rate of the metal.
For the two major oxidizing species (O2 and H2O2) in the BWR coolant, the electrochemical characteristic of H2O2 on the metal surface is relatively more complicated than that of O2. In theory, H2O2 could act not only as an oxidizing agent but also as a reducing one in high temperature water. Furthermore, H2O2 could easily decompose into O2 and water at elevated temperature. As a result, it is difficult to quantitatively evaluate the corrosion mitigation effectiveness of TiO2 treatment in H2O2 containing environments.
In the past several years, we have studied the impact of TiO2 treatment on the corrosion behavior of Type 304 SS in high temperature water of various oxygen concentrations [Citation10]. The aim of this study was to explore the electrochemical characteristics of H2O2 and TiO2 treated Type 304 SS under UV irradiation in high-temperature pure water. TiO2 was deposited by hydrothermal deposition treatment on 304 SS specimens at various temperatures. Electrochemical polarization analyses were carried out under a designated water chemistry condition of 300 ppb H2O2. Experimental results on variations in corrosion potential and corrosion current density of both untreated and TiO2-treated specimens under UV irradiation are reported and discussed.
2. Experimental
2.1. Specimen preparation
A commercial grade ASTM SUS304 SS plate was used in this study. shows the chemical composition of this material as measured by a glow discharge spectrometer. For electrochemical polarization tests, the SS plate was machined into square disks with a dimension of 20 mm×20 mm×1 mm, and a small hole of 1 mm in diameter was drilled at one corner of each disk specimen. All specimens were then sealed inside a quartz tube in vacuum and thermally sensitized in a chamber furnace at 650 °C for 24 h. After the sensitization process, both sides of the specimens were mechanically polished by abrasive paper starting from 80 grit up to 1200 grit. Each specimen was then ultrasonically cleaned in acetone (CH3COCH3), dried, and rinsed in distilled water for 10 minutes. The degree of sensitization of a specimen was determined by the double-loop electrochemical potentiokinetic reactivation method, and all specimens were found heavily sensitized.
Table 1. The composition (wt%) of 304 SS used in this work.
2.2. Pre-oxidation treatment
To produce stable oxide films on the specimens, a pre-oxidation treatment on each specimen was conducted in pure water under a high-temperature (288 °C) and high-pressure (8.3 MPa) condition in a circulation loop system. The coolant flow rate at the outlet of the loop (diameter of 1/4 inch) was measured at approximately 30 mL/min. A low conductivity of 0.06 μS/cm of the coolant was measured using a GLI conductivity meter. shows a conceptual schematic of the loop system. The specimens were exposed to the foregoing environment with a dissolved oxygen concentration ([O2]dis) of 300 ppb for 14 days. These specimens were designated as Prefilm ones. The surface morphologies of the specimens were examined by field emission scanning electron microscopy (SEM) and energy dispersive X-ray spectroscopy (EDX) of JEOL JSM-6330F.
2.3. TiO2 treatment by hydrothermal deposition
TiO2 (Anatase-type) particles with an average size of 38 nm in diameter were used in this study. These particles were deposited on the 304 SS specimens by hydrothermal deposition at different temperatures of 150 °C and 280 °C with a treating time of 96 h. The concentrations of TiO2 were maintained at 100 and 10 ppm during the deposition treatment. The treated specimens were designated as N-TiX-Y, where X and Y are the deposition temperature and the TiO2 concentration, respectively. The surface of the treated specimen was analyzed by SEM, and the TiO2 coating was characterized by EDX and X-ray diffraction pattern analyses. The TiO2 loading was estimated after the inductively coupled plasma mass spectrometry (ICP-MS) analysis. shows a schematic view of the TiO2 deposition loop. Preparation conditions of all specimens used in this study were summarized in
Table 2. Preparation conditions of the specimens.
2.4. ECP and polarization measurements
ECP measurements and potentiodynamic polarization analyses were conducted in a high-temperature pure water circulation loop, coupled with an autoclave that could accommodate the testing specimens. The water chemistry in the loop could be controlled by properly adjusting the injection rates of O2, H2, and Ar gases into the reservoir tank. The flow rate at the outlet of the autoclave was measured at approximately 30 mL/min. On the other hand, since H2O2 is unstable in high temperature water due to its easy decomposition into O2 and water, bulk H2O2 solution preserved in a dark glass tank was directly injected into the autoclave through a Teflon-lined tube by a high pressure metering pump. The H2O2 concentration in the autoclave was determined by the mixing ratio of the injected H2O2 and the circulating coolant. shows the cross-sectional view of the specimens and electrodes setup in the autoclave for ECP measurements and polarization tests. A three-electrode system, including an Ag/AgCl reference electrode, a platinum counter electrode, and pre-oxidized or TiO2 treated specimens as working electrodes, was used in this study. Heat-shrinkable PTFE tubes were used for electrical insulation between the connecting wires and the coolant. The electrodes were then mounted in a CONAX fitting secured at the top lid of the autoclave and inserted into the coolant region inside the autoclave. The Ag/AgCl electrode was installed in parallel with the platinum electrode and the specimens. The measured ECPs were converted to the standard hydrogen electrode (SHE) scale. The body of autoclave was deliberately machined with a sapphire-sealed hole, serving as a viewing window, through which the specimen could be irradiated by UV. This experiment used a 250–365 nm UV light source, which was sufficient to excite the anatase type TiO2. The intensity of the UV light was set at 4500 mW/cm2 with a wavelength of 365 nm, and the intensity of the UV light irradiating onto the metal surface was calculated to be 17 mW/cm2.
Figure 3. Arrangement of the specimen and other electrodes in the autoclave for ECP and polarization tests.
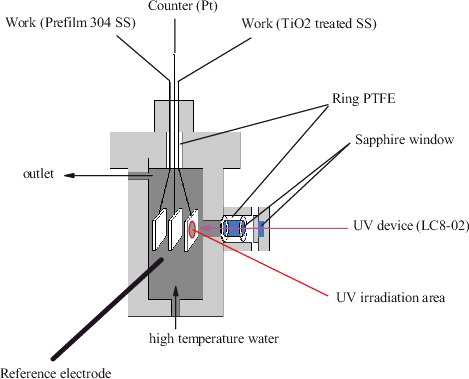
The concentration of H2O2 was controlled by using a metering pump to inject a proper amount of H2O2 directly into the autoclave and was maintained at 300 ppb. A Gamry PC4/300 electrochemical analyzer was adopted to perform the polarization analyses. The ECP for each specimen under the designated water chemistry condition was obtained prior to each polarization test. The potential increment or decrement was set at 30 mV for every 10 minutes for the polarization analyses. The cathodic polarization range was 0.3 V below the corrosion potential (Ecorr), and the anodic polarization range was 0.3 V above the Ecorr. The positive-feedback loops in the electrochemical analyzer were activated to compensate for the ohmic resistance experienced by the testing system.
3. Results and discussion
3.1. Morphologies and properties of the surface oxides and TiO2 coatings
The surface morphology of the Prefilm specimen after the pre-oxidation treatment is shown in . The oxide particles were uniformly distributed on the surface of the specimen, and the oxide particle size ranged from 0.5 to 1 µm in diameter. It was also observed that the surface oxides exhibited a two-layer structure. EDX analyses revealed that the O/Fe ratios in the outer layer and the inner layer of the oxides were different, as shown in
shows the SEM micrographs and EDX mapping results of the four TiO2-treated 304 SS specimens, namely, N-Ti150-100, N-Ti150-10, N-Ti280-100, and N-Ti280-10. The SEM images show that the TiO2 nanoparticles were deposited on the oxide surfaces in an uneven and discontinuous manner. The TiO2 coatings could be either thick or relatively thin, as characterized by the Ti signal mapping. In spite of uneven deposition and discontinuous distribution, the TiO2 loadings on specimens N-Ti280-100 and N-Ti280-10 were found higher than those on specimens N-Ti150-100 and N-Ti150-10, as verified by ICP-MS loading estimations. The data are summarized in . The TiO2 loadings on the specimens increased with both increasing treating temperature and increasing TiO2 concentration.
Table 3. TiO2 loadings on the TiO2-treated specimens.
3.2. Electrochemical polarization analyses
shows ECP variations on the specimens exposed to 288 °C pure water at the designated concentration ([H2O2]) of 300 ppb before and after UV irradiation. Upon UV irradiation, the ECPs of N-Ti150-100 and N-Ti280-100 decreased promptly by around 12 to 15 mV and returned to their original values when the UV light was off. The ECP decreases on N-Ti150-10 and N-Ti280-10 upon UV irradiation were about 5 mV and 7 mV, respectively. The difference between the ECPs without and with UV was greater on the specimen treated with a higher TiO2 concentration at a higher temperature.
Figure 6. ECP variations of the TiO2-treated specimens in 288 °C pure water at 300 ppb H2O2 under interrupted UV irradiation.
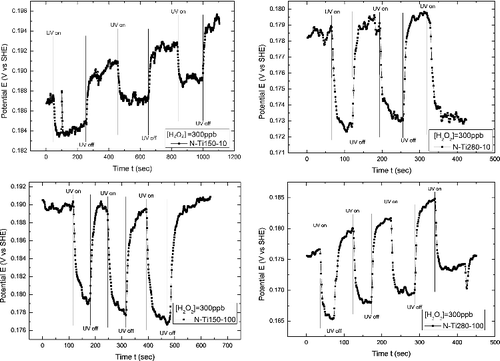
It should be noted that prior to each single polarization test the specimen was immersed in the test environment for a period of time for the ECP to stabilize. shows the polarization curves of the untreated and TiO2-treated specimens with and without UV irradiation in the high temperature water containing 300 ppb H2O2. The Tafel extrapolation method was used to calculate the icorr. A small linear Tafel region on each of the cathodic polarization curve could be observed starting from 50 mV below the Ecorr, which is also the second data point below Ecorr. The linearity was not maintained over a large range of the scanned potential, and only from second data to the fourth data points were used to construct the Tafel line. The intersection of the Tafel line and the Ecorr was the icorr. The ECP and corrosion current density (icorr) data are summarized in . In the absence of the UV light, there was no significant difference between the untreated and treated specimens in terms of anodic current density. Slight decreases in the cathodic current densities of the four treated specimens were observed. Among them, a comparatively more significant decrease in cathodic current density on the N-Ti280-100 specimen was recorded, and the icorr of this specimen was 23.3 μA/cm2, which was much lower than that of the Prefilm specimen at 37.2 μA/cm2. This outcome was attributed to the higher Ti loading and thus, the greater inhibiting efficiency on the cathodic reaction. In general, the icorrs and the ECPs of all TiO2-treated 304 SS specimens were reduced in pure water with 300 ppb H2O2 in the absence of UV radiation, and the specimens treated at a higher TiO2 concentration resulted in more effective reductions in icorr and ECP.
Figure 7. Polarization curves of the Prefilm and TiO2-treated specimens in 288 °C water at 300 ppb [H2O2].
![Figure 7. Polarization curves of the Prefilm and TiO2-treated specimens in 288 °C water at 300 ppb [H2O2].](/cms/asset/c66d74fe-35aa-4839-8944-9801dba4fcdd/tnst_a_1120692_f0007_oc.jpg)
Table 4. ECPs and icorrs of Prefilm and TiO2-treated specimens at 300 ppb H2O2.
In the presence of UV radiation, photo-catalytic reactions occurred on the surfaces of all TiO2-treated 304 SS specimens and generated anodic currents to reduce the ECPs of the treated specimens. For the N-Ti150-10 and N-Ti280-10 specimens, slightly lower ECPs by 10 and 12 mV than the corresponding Prefilm specimens were observed under UV irradiation at 300 ppb H2O2. The ECP of N-Ti-150-100 with UV was lower than that of Prefilm by 19 mV. In the meantime, the ECP of N-Ti280-100 with UV was 162 mVSHE, which was lower than that of Prefilm by 22 mV. The results were consistent with what the theory predicted in that the n-type TiO2 coating would generate excess electrons under UV irradiation, leading to a lower potential during the polarization analysis. Accordingly, the corresponding icorrs of the four treated specimens were also reduced. Most distinctly, the icorr of N-Ti280-100 with UV was 7.7 μA/cm2, significantly lower than that of Prefilm at 37.2 μA/cm2. The polarization data under UV irradiation for all specimens are also summarized in . The outcome clearly showed that the degree of reduction in icorr of the TiO2-treated specimen could be as high as 80% in 288 °C water with 300 ppb H2O2, signifying the effectiveness of TiO2 coatings coupled with UV on corrosion mitigation of treated Type 304 SS.
3. Conclusions
The technique of TiO2 treatment coupled with UV irradiation could be adopted as a corrosion mitigation measure for Type 304 SS in high-temperature pure water in the presence of H2O2. TiO2 coatings could be obtained on pre-oxidized Type 304 SS specimens by hydrothermal deposition. A higher TiO2 loading could be achieved at a deposition condition of a higher TiO2 concentration and a higher temperature. In high-temperature pure water with 300 ppb H2O2, slight decreases in ECP and icorr were observed on TiO2-treated specimens even in the absence of UV irradiation. In the presence of UV irradiation, the photo-catalytic reaction on the surface of the treated 304 SS specimen would further enhance the corrosion mitigation efficiency of TiO2 coatings, and the degree of reduction in icorr of the TiO2-treated specimen could be as high as 80%. Although the TiO2 treatment in combination with UV radiation could not reduce the ECP below the Ecrit (−0.23 VSHE), the corrosion rate of TiO2-treated specimens under UV irradiation would be effectively reduced in H2O2-rich, high-temperature pure water.
Acknowledgments
The authors wish to express sincere gratitude to Taiwan Power Company for the financial support [contract number TPC-056-98-00023]. We also acknowledge the technical information supported from Ms Ching Chang of Taiwan Power Company.
Disclosure statement
No potential conflict of interest was reported by the authors.
References
- BWR Vessel and Internals Project, Volume 1. BWR water chemistry guidelines – mandatory, needed, and good practice guidance and volume 2: BWR water chemistry guidelines – technical basis. USA: EPRI; 2014. BWRVIP-190 Revision 1.
- Macdonald DD, Yeh T K, Motta AT. Simultaneous radiolysis, ECP, and crack growth modeling of components in BWR coolant systems: corrosion 95. Orlando (FL): NACE; 1995.
- Hettiarachchi S., Law RJ, Diaz TP, et al. The first in-plant demonstration of noble metal chemical addition technology for IGSCC mitigation of BWR internal. In: Proceedings of the 8th International Symposium on Environmental Degradation of Materials in Nuclear Power Systems—Water Reactors; 1997 August 10–14. Amelia Island (FL): ANS.
- Kim YJ, Andresen PL. Application of insulated protective coatings for reduction of corrosion potential in high temperature water. Corrosion. 1998;54(12):1012–1017.
- Yeh TK, Liu TC, Tsai CH. The influence of ZrO2 treatment on the electrochemical behavior of oxygen and hydrogen on type 304 stainless steels in high temperature water. J Nucl Sci Technol. 2005;42(9):809–815.
- Zhou ZF, Chalkova E, Lvov SN, et al. Development of a hydrothermal deposition process for applying zirconia coatings on BWR materials for IGSCC mitigation. Corros Sci. 2007;49:830–843.
- Fukaya Y, Akashi M, Yotsuyanagi T, et al. Photoelectrochemical protection of stainless alloys in BWR primary coolant environment. In: Proceedings of the 10th International Symposium on Environment Degradation of Materials in Nuclear Power Systems –Water Reactors; 2001 August 5–9. Lake Tahoe (Nevada): NACE. (Paper No. 16).
- Okamura M, Osato T, Ichikawa N, et al. Corrosion mitigation of BWR structural materials by the photoelectric methods with TiO2 -laboratory experiments of TiO2 effect on ECP behavior and materials integrity. In: Proceedings of the 12th International Symposium on Environmental Degradation of Materials in Nuclear Power Systems—Water Reactors; 2005 August 14–18. Salt Lake City (UT): TMS.
- Takamori K, Suzuki J, Suzuki S, et al. Development of SCC mitigation method in BWR plant by TiO2 technique. In: Proceedings of the 15th International Conference on Nuclear Engineering; 2007 April 22–26; Nagoya, Aichi, Japan.
- Yeh TK, Huang YJ, Wang MY, et al. Hydrothermal treatments of TiO2 on Type 304 stainless steels for corrosion mitigation in high temperature pure water. Nucl Eng Des. 2013;254:228–236.