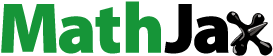
ABSTRACT
In this work, paraffin and paraffin/bitumen composites with additions of boron oxide (B2O3) were prepared to evaluate the viscosity, flexural, and thermal neutron shielding properties for uses as thermal neutron shielding materials. The results showed that the addition of 3 wt% or 9 wt% bitumen to paraffin increased the overall flexural properties with the content of 9 wt% bitumen having the highest values. The improvement in flexural properties made the composites less brittle, stiffer, and longer-lasting. Furthermore, different contents of B2O3 (0, 7, 14, 21, 28, and 35 wt%) were added to paraffin and paraffin/bitumen composites to investigate the effects of the B2O3 contents. The results indicated that an increase in B2O3 contents improved the shielding properties but slightly reduced the flexural properties. Specifically for 5-mm paraffin and 5-mm paraffin/bitumen samples with 35 wt% of B2O3, both samples could reduce neutron flux by more than 70%. The overall results suggested that the paraffin and paraffin/bitumen composites with additions of B2O3 showed improved properties for utilization as effective thermal neutron shielding materials.
1. Introduction
Neutron technologies have become one of the most important tools nowadays in advancing both scientific and non-scientific fields. Examples of applications are the measurement of water film thickness [Citation1], neutron imaging for in situ non-destructive analysis on an operating polymer electrolyte membrane hydrogen fuel cell [Citation2], boron neutron-capture therapy for glioblastoma multifome (GBM) [Citation3], analysis of 12,900-year-old stone artifacts [Citation4], and neutron autoradiography for paintings [Citation5]. As demands for neutron technologies significantly increase, the radiation safety of workers and the general public becomes an issue of great concern for all neutron facilities. Installation of an effective shielding material is one possible method to promote a safe working environment. A number of types and additional properties of neutron shielding materials are available for selection depending on the requirements of individual applications [Citation6,Citation7].
Paraffin (CH
), which is known as a material for candle-making and in waterproofing agents, is one of the materials most notably used in nuclear facilities dating back to the famous experiment of James Chadwick resulting in the discovery of neutrons in 1932 [Citation8]. Properties of paraffin that meet the requirements of a neutron moderator and shield are its high hydrogen content, relatively low cost, moldability, and excellent electrical insulation [Citation9,Citation10]. However, pure paraffin is brittle and has low flexural properties at room temperature, thus presenting great risks of breakage during operations. Paraffin also has low viscosity in a liquid form (∼3 mPa·s at the atmospheric pressure and the temperature of 100 °C [Citation11]), which could possibly create uneven distribution or layers of other additives during the cooling down process. To improve the overall properties of paraffin composites, certain additives are required. One possible additive that could be used is bitumen. Bitumen is a black or dark-colored, amorphous, sticky liquid or semi-solid form of petroleum. The primary uses of bitumen are in paving applications due to its excellent physical and rheological properties [Citation12,Citation13]. Bitumen can strongly bind and hold other pavement components together, resulting in higher stiffness and a more level surface [Citation14]. Bitumen also has relatively high dynamic viscosity (80–320 Pa·s at the atmospheric pressure and the temperature of 60 °C [Citation15]), which could act as an additive to increase the viscosity of pure paraffin. Mixtures of paraffin and bitumen have been studied by Silva et al., in which 6 wt% commercial synthetic paraffin wax was added to bitumen in order to reduce the construction temperatures and dynamic viscosity of bitumen [Citation16]. Thus, it is intriguing to investigate whether bitumen could instead act as an additive to paraffin to increase the viscosity and flexural properties.
The mechanism of paraffin for uses in thermal neutron shields mainly involves elastic scattering between neutrons and their hydrogen nuclei in order to substantially reduce neutron energy. However, the total thermal neutron cross section of hydrogen nuclei is quite low (∼0.33 barns for neutron absorption cross section and ∼82.02 barns for total neutron scattering cross section [Citation17]), which leads to low probabilities of interactions and considerably thick sheets of paraffin are required in order to effectively shield thermal neutrons. To improve the thermal neutron shielding properties and to reduce the thickness required, high-neutron-absorption-cross-section compounds such as boron oxide (B2O3), boron carbide (B4C), or boron nitride (BN) could be introduced into paraffin and paraffin/bitumen composites. Examples of previous works that used boron compounds in neutron shielding applications are composites of ethylene–propylene diene rubber/low density polyethylene/B4C [Citation18], composites of natural rubber–styrene butadiene rubber blends/boron oxide/iron(III) oxide [Citation19], and composites of wood/natural rubber with boric acid or boron oxide [Citation20].
The objectives of the current work were to investigate the density, viscosity, flexural, and thermal neutron shielding properties of paraffin and paraffin/bitumen composites with different addition levels of B2O3 for potential uses as thermal neutron shielding materials. Furthermore, the properties of paraffin and paraffin/bitumen composites were compared to investigate whether bitumen was in fact a suitable additive to improve the overall mechanical properties. Details of the methodology and results are thoroughly discussed in this article.
2. Materials and methods
2.1. Materials and chemicals
The ingredients and their contents used in this work are shown in . The average particle size of B2O3 powders measured using a scanning electron microscope (SEM) was ∼200 m.
Table 1. Material formulations of paraffin and paraffin/bitumen composites.
The semi-refined 58 paraffin used in this work had oil content less than 1.8% with the melting temperature of 58–60 °C, while AC 60/70 bitumen, which was recommended for road construction, had the penetration value at 25 °C of 60–70 mm. The rationales of selecting both types of materials were due to their low costs, wide availability in the market, and reasonable properties for developing thermal neutron shielding materials.
2.2. Preparation of paraffin and paraffin/bitumen composites
For each formulation, 3-kg paraffin was heated at 60–70 °C using a hot plate and a magnetic stirrer for 20 minutes until all the paraffin was in a clear liquid form. Specifically for the paraffin/bitumen composites, 3 and 9 wt% bitumens that were preheated at 80–100 °C were added to the paraffin, respectively, and mixed for another 10 minutes. Different contents of B2O3 powders were then added to the mixtures and further mixed for 5 minutes. Next, the mixtures were poured into separate 30 cm × 30 cm × 5 mm molds and left to cool down for 2 hours before being removed from the molds.
2.3. Density measurement
In order to measure the density of the composites, 1 cm ×1 cm × 1 cm samples from each formulation were weighed using a four decimal point digital scale. Each sample was then submerged into a 100-mL measuring cylinder that was filled with 50 mL of water. Specifically for samples that were not fully submerged, a small pin was used to push the samples into water such that all parts of the samples were fully submerged under the water. Changes in water volume read directly from scales on the measuring cylinder were then used to calculate density of each sample using the equation ρ = m/V, where ρ is the density, m is the mass, and V is the change in water volume after the sample was fully submerged.
2.4. Kinematic viscosity and flexural property measurement
The kinematic viscosity of all composites was evaluated according to the ASTM D445 standard test method for liquid petroleum products, both transparent and opaque. The procedures involved the measurement of the time for a volume of liquid to flow under gravity through a calibrated glass capillary viscometer at the temperature of 40 and 100 °C. Flexural properties consisting of flexural strength, flexural modulus at 100% elongation, and maximum elongation at break were investigated using a universal testing machine (Autohraph AG-1 Shimadzu, Tokyo, Japan) following ASTM D790 (1999) with a 5-kN load cell at a test speed of 1.3 mm/minute. The sizes of paraffin and paraffin/bitumen composites used for flexural tests were 5 mm × 50 mm × 150 mm.
2.5. Scanning electron microscopy (SEM) studies
The cross-sectional surfaces of paraffin and paraffin/bitumen composites were coated with gold using an ion sputtering technique and examined using a Quanta 450 FEI SEM machine at 5-kV accelerating voltage. The main purpose of the SEM studies was to determine the morphology of the paraffin and paraffin/bitumen composites.
2.6. Thermal neutron shielding properties
The thermal neutron transmission ratios for all paraffin and paraffin/bitumen composites were tested at the Thailand Institute of Nuclear Technology using the experimental set-up shown in . The neutron source used in the tests was a 300-mCi 241Am/Be enclosed in a paraffin cylinder, which had the specific gravity of 0.9. A stack of 5-cm high density polyethylene (HDPE) sheets, which had the specific gravity of 0.95, was used as moderators. Counts of thermal neutron detection were measured using a 50.8 mm × 19.8 mm × 140.9 mm rectangular 3He-filled neutron detector, which had the thermal neutron detection efficiency of ∼60%, connected to a set of amplifiers and a timer/counter for data processing. A 30 cm × 30 cm × 5 mm sample was placed between the HDPE sheets and the neutron detector with a space of approximately 5 cm in between them. For each sample, five independent tests were performed and average counts (I) of detection were recorded to calculate the thermal neutron transmission ratio (I/I0), where I0 is the reference count recorded without samples. Furthermore, to examine the relationship between the thickness of the composites and thermal neutron shielding properties, stacks of samples with a total thickness varying from 5 to 40 mm were used to calculate I/I0. It should be noted that, although it was possible for thermal neutrons that initially travelled in the direction of the detector to scatter off hydrogen atoms inside paraffin composites and deviate outside the detector area, which would lower I/I0 and overestimate neutron shielding performances, the opposite phenomena could also occur with comparable probabilities. These two events would offset or reduce effects from angle deviations, causing only small errors in thermal neutron shielding measurement.
3. Results and discussion
3.1. Density
Values of the density of the paraffin and paraffin/bitumen composites are shown in .
Table 2. Densities of paraffin and paraffin/bitumen composites.
As shown in , densities of the composites increased with the addition of 35 wt% B2O3 due to higher density of B2O3 powders compared to the density of paraffin shown in , while the addition of 3 and 9 wt% of bitumens only increased densities of the composites slightly due to comparable densities of paraffin and bitumen.
3.2. Kinematic viscosity
The results of viscosity analysis for the paraffin and paraffin/bitumen composites are shown in .
Table 3. Viscosity of paraffin and paraffin/bitumen composites.
As shown in , the values of kinematic viscosity increased as the bitumen contents were increased from 0 to 9 wt% due to the much higher viscosity of bitumen compared to pure paraffin. The increase in viscosity resulted in a much better distribution of the B2O3 as shown in (d), compared to Figure (b,c), where the B2O3 layers can be clearly seen at the bottom of the samples. This is because, although B2O3 powders were fairly dispersed throughout the mixtures after the mixing, B2O3 molecules were denser than pure paraffin, resulting in the B2O3 powders sinking rapidly to the bottom of the samples during cooling down. However, as the composites became more viscous by adding 9 wt% bitumen, it was more difficult for the B2O3 molecules to gravitate to the bottom of the sample, resulting in a better dispersion of the B2O3 powders.
Figure 2. Cross-sectional images of (a) pure paraffin, (b) paraffin with 35 wt% B2O3, (c) paraffin/3 wt% bitumen with 35 wt% B2O3, and (d) paraffin/9 wt% bitumen with 35 wt% B2O3, with (b) and (c) clearly showing layers of B2O3 at the bottom of the samples, while (d) has a better distribution of B2O3 throughout the cross-sectional area.
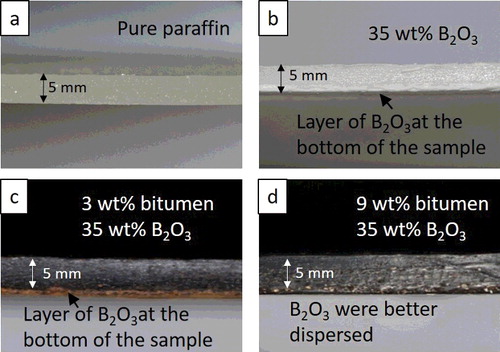
3.3. Flexural properties and SEM studies
As shown in Figures –, all values of flexural properties increased as the bitumen contents were increased from 0 to 3 wt% and 9 wt%, respectively, especially in the maximum elongation at break, where the paraffin samples with 9 wt% bitumen had more than four times higher values than the paraffin samples because the bitumen acted as a reinforcing filler and a binder that strengthened the composites, similar to the role of bitumen in paving applications, in binding all the aggregates together [Citation21]. The effects of bitumen on improving the flexural properties can be seen in , which shows SEM images of paraffin, paraffin/3 wt% bitumen, and paraffin/9 wt% bitumen, taken at the middle of the cross-sectional area. (a) shows a rougher texture and larger air bubbles than (b,c). These air bubbles were formed during manufacturing process when the mixtures of paraffin and paraffin/bitumen composites were poured into colder mold and during cooling down process. However, formation rates and sizes of air bubbles varied with magnitudes of the liquid viscosity, in which higher viscous liquids tend to have lower formation rates and smaller bubbles[Citation22,Citation23]. These effects caused the paraffin to be brittle and less stiff.
Figure 3. Flexural strengths of paraffin and paraffin/bitumen composites with or without additions of 35 wt% B2O3. The error bars shown are the standard deviation (S.D.) of five independent measurements for each formulation.
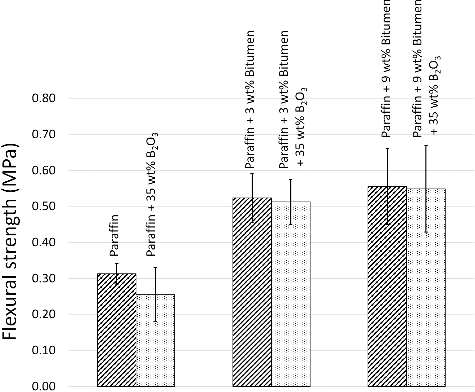
Figure 4. Flexural modulus of paraffin and paraffin/bitumen composites with or without additions of 35 wt% B2O3. The error bars shown are the standard deviation (S.D.) of five independent measurements for each formulation.
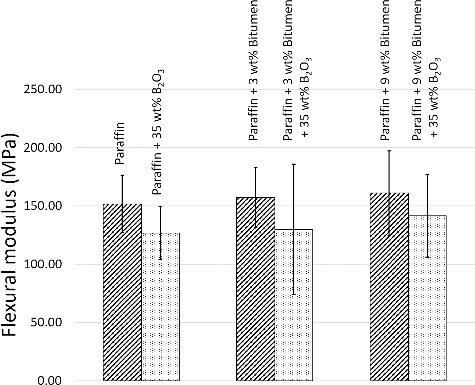
Figure 5. Maximum elongation at break of paraffin and paraffin/bitumen composites with or without additions of 35 wt% B2O3. The error bars shown are the standard deviation (S.D.) of five independent measurements for each formulation.
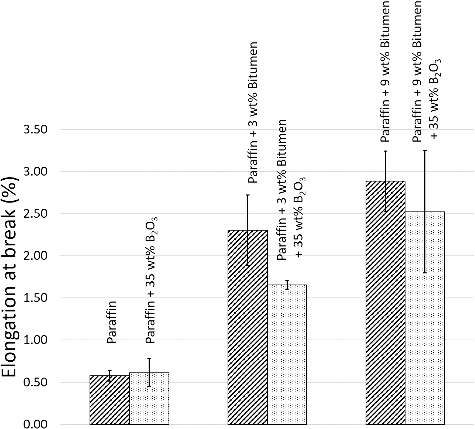
Figure 6. SEM images with a magnification of 300x of (a) paraffin with 35 wt% B2O3, (b) paraffin/3 wt% bitumen with 35 wt% B2O3, and (c) paraffin/9 wt% bitumen with 35 wt% B2O3.
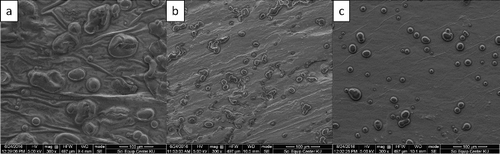
shows the paraffin/bitumen sample during the flexural tests, which illustrates higher stiffness in the sample from adding bitumen. The sample could be bent much further than pure paraffin could without rupturing.
Figure 7. (a) Paraffin/bitumen sample during flexural testing and (b) paraffin/bitumen sample after flexural testing, showing bending without rupture.
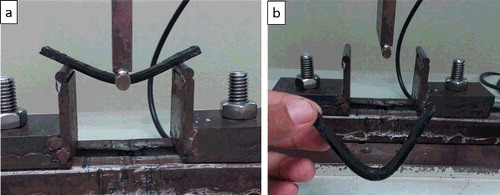
Another interesting result was the comparison between samples with and without B2O3, as the samples with B2O3 had slightly lower flexural properties perhaps because the B2O3 powders and paraffin did not have strong interfacial bonding, which lowered the overall strength of the composites.
3.4. Thermal neutron shielding properties
As shown in , values of thermal neutron transmission ratios continuously decreased from ∼95% to ∼30% as the contents of B2O3 were increased from 0 to 35 wt%, while samples with different contents of bitumen but the same amount of B2O3 did not show significantly different properties. These behaviors could be explained by the fact that the most influential ingredient that could interact with thermal neutrons and convert them to alpha particles (which are much easier to shield) was the boron nuclei in B2O3. The increase in contents of B2O3 led to an increase in the boron nuclei per unit area, which caused more interactions and more thermal neutron absorption.
Figure 8. Thermal neutron transmission ratios (I/I0) of 5-mm paraffin and 5-mm paraffin/bitumen samples with additions of varying contents of B2O3. The error bars shown are the standard deviation (S.D.) of five independent measurements for each formulation.
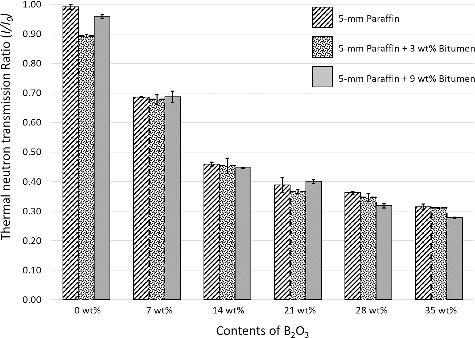
As shown in , thicker samples for the same formulation could more efficiently reduce transmitted thermal neutrons because thicker samples implied more boron nuclei per unit area available for interactions with incoming thermal neutrons. For all three formulations with the addition of 35 wt% B2O3, the half-value layer (HVL) and tenth-value layer (TVL), which indicate the average thickness of material needed to absorb 50% and 90% of incoming thermal neutrons, respectively, were ∼0.3 and ∼2.8 cm. The results were better for pure paraffin that produced an HVL value of ∼4 cm [Citation24] and for a concrete with 25.1% anhydrous borax (Na2B4O7) that had a TVL value of ∼29.64 cm [Citation25].
Figure 9. Thermal neutron transmission ratios (I/I0) of varying thicknesses of paraffin and paraffin/bitumen samples with additions of 35 wt% B2O3. The error bars shown are the standard deviation (S.D.) of five independent measurements for each formulation.
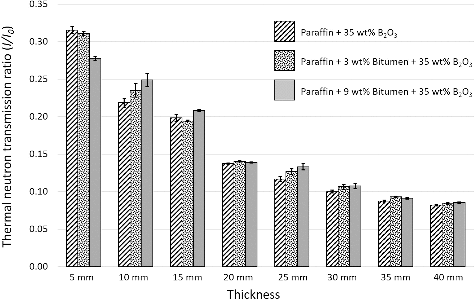
As a result, the paraffin and paraffin/bitumen composites with the addition of B2O3 featured improvements in toughness, durability, distribution of additives, and mechanical/thermal neutron shielding performance. This work could be used as a reference for future research in developing neutron shielding materials.
4. Conclusions
Paraffin and paraffin/bitumen composites with additions of B2O3 were prepared and their density, kinematic viscosity, flexural, morphological, and thermal neutron shielding properties were determined. The results showed that bitumen acted as a reinforcing filler and a binder in the paraffin/bitumen composites, while B2O3 powders worked effectively as an active ingredient to shield thermal neutrons. The results also indicated that the kinematic viscosity and flexural properties of the composites increased as the bitumen contents were increased, while the ability to shield thermal neutrons improved as the B2O3 contents were increased. Furthermore, for a fixed B2O3 content at 35 wt%, thicker layers of both paraffin and paraffin/bitumen composites tended to shield thermal neutrons with better efficiency as more boron nuclei were available for interactions. The HVL and TVL values for both paraffin and paraffin/bitumen composites were approximately 0.3 and 2.8 cm, respectively. Based on the experimental results, it could be concluded that paraffin and paraffin/bitumen composites had efficiently improved properties, making them suitable for use in thermal neutron shielding applications.
Acknowledgments
This research is supported in part by the Graduate Program Scholarship from the Graduate School, Kasetsart University. We would also like to acknowledge supports from the Thailand Institute of Nuclear Technology (TINT); Polymer Processing and Flow (P-PROF) Research Group, Division of Materials Technology, School of Energy, Environment and Materials, King Mongkut's University of Technology Thonburi (KMUTT); Faculty of Science, Kasetsart University.
Disclosure statement
No potential conflict of interest was reported by the authors.
Additional information
Funding
References
- Dupont J, Mignot G, Zboray R, et al. Infrared film thickness measurement: comparison with cold neutron imaging. J Nucl Sci Tech. 2016; 53(5): 673–681.
- Satija R, Jaconson DL, Arif M, et al. In situ neutron imaging technique for evaluation of water management systems in operating PEM fuel cells. J Power Sources. 2004; 129(2): 238–245.
- Chadha M, Capala J, Coderre JA, et al. Boron neutron-capture therapy (BNCT) for glioblastoma multifome (GBM) using the epithermal neutron beam at the Brookhaven National Laboratory. Int J Radiat Oncol Biol Phys. 1998; 40(4): 829–834.
- Boulanger MT, Buchanan B, O’Brien MJ, et al. Neutron activation analysis of 12,900-year-old stone artifacts confirms 450-510+ km Clovis tool-stone acquisition at Paleo Crossing (33ME274), northeast Ohio, U.S.A. J Archaeol. 2015; 53: 550–558.
- Schroder-Smeibidl B, Laurenze-Landsberg C, Schmidt C, et al. BENSC neutron for “cultural heritage” research: neutron autoradiography of paintings. Notiziario thermal neutroni e Luce di Sinchrotrone. 2006; 11: 24–27.
- Sukegawa AM, Anayama Y, Ohnishi S, et al. Development of flexible neutron-shielding resin as an additional shielding material. J Nucl Sci Tech. 2011; 48(4): 585–590.
- Pehlivanovic B, Marinkovic P, Avdic S. The effects of shielding around the source on neutron energy spectra. Radiat Eff Defect. 2011; 166(12): 901–907.
- Chadwick J. Possible existence of a neutron. Nature. 1932; 129: 312.
- da Silva AX, Crispim VR. Moderator-collimator-shielding design for neutron radiography systems using 252Cf. Appl Radiat Isotopes. 2001; 54(2): 217–225.
- Seo KS, Kim CH. Comparison of titanium hydride (TiH2) and paraffin as neutron moderator material in a prompt gamma scanning system. J Korean Phys Soc. 2006; 48(4): 855–858.
- Viscopedia [Internet]. [cited 2016 Sept 16]. Available from: http://www.viscopedia.com/viscosity-tables/substances/paraffin-wax/
- Farias LGAT, Leitinho JL, Amoni BDC, et al. Effects of nanoclay and nanocomposites on bitumen rheological properties. Constr Build Mater. 2016; 125: 873–883.
- Zhang C, Yu J, Xu S, et al. Influence of UV aging on the rheological properties of bitumen modified with surface organic layered double hydroxides. Constr Build Mater. 2016; 123: 574–580.
- Types and Properties of Bituminous Materials [Internet]. [cited 2016 Sept 16]. Available from: http://www.aboutcivil.org/importance-types-of-bituminous-materials.html
- Bitumen [Internet]. [cited 2016 Sept 22]. Available from: https://www.iocl.com/Products/BITUMEN_ Spec.pdf
- Silva H, Oliveria J, Peralta J, et al. Optimization of warm mix asphalts using different blends of binders and synthetic paraffin wax contents. Constr Build Mater. 2010; 24: 1621–1631.
- Sears VF. Neutron scattering lengths and cross sections. Neutron News. 1992; 3(3): 26–37.
- Abdel-Aziz MM, Gwaily SE, Makarious AS, et al. Ethylene-propylene diene rubber/low density polyethylene/boron carbide composites as neutron shields. Polym Degrad Stabil. 1995; 50(2): 235–240.
- Jumpee C, Wongsawaeng D. Innovative neutron shielding materials composed of natural rubber-styrene butadiene rubber blends, boron oxide and iron(III) oxide. J Phys Conf Ser. 2015; 611: 012019.
- Ninyong K, Wimolmala E, Sombatsompop N, et al. Potential use of NR and wood/NR composites as thermal neutron shielding materials. Polym Test. 2017;59;336–343.
- Kendall M, Baker B, Evans P et al, . Foamed bitumen stabilisation [Internet]. [cited 2016 Sept 19]. Available from: http://www.auststab.com.au/pdf/tp26.pdf
- Pancholi K, Stride E, Edirisinghe,. Dynamics of bubble formation in highly viscous liquids. Langmuir. 2008; 24(8): 4388–4393.
- Yamada K, Emori H, Nakazawa K. Time-evolution of bubble formation in a viscous liquid. Earth Planets Space. 2008; 60: 661–679.
- Orak S, Baysoy DY. Neutron shielding properties of concrete with boron and boron containing mineral. Acad Platform J Eng Sci. 2013:15–19. DOI:10.5505/apjes.2013.99609
- Lorente Fillol A, Gallego Diaz EF, Vega-Carrillo HR et al, . Neutron shielding properties of a new high-density concrete, In: Gallego E, Gonzalez A, Amaral E et al, ., editors. Strengthening radiation protection worldwide- highlights, global perspective and future trends. Proceedings of the 12th International Congress of the International Radiation Protection Association, IRPA-12, 2008 October 19-24; Bueno Aires, Vienna: International Atomic Energy Agency; 2010. p. 1–10.