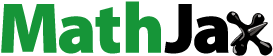
ABSTRACT
The reaction between fission products Mo(VI) and Zr(IV) in hot nitric acid solution during the spent fuel dissolution process leads to the formation of hydrated zirconium molybdate precipitate. This solid can include other metallic cations at the 4th oxidation state (+ IV) inside the crystal such as Pu(IV).
Precipitations in the inactive surrogate system of mixed zirconium-cerium molybdates Mo-Zr(IV)-Ce(IV) were performed all over the cerium molar percentage scale from 0 to 100%, Ce(IV) being used as a surrogate of Pu(IV). The crystal structures were identified from powder X-ray diffraction patterns and complementary investigations by SEM and elemental analyses were carried out. The solid composition pointed out two phases that are ZrMo2O7(OH)2(H2O)2 and Ce3Mo6O24(H2O)4. Each of these compounds was proved being a solid solution within which the ZrIV and CeIV atoms can substitute each other.
Moreover, the influence of the cerium molar fraction on the precipitate solubility was investigated and showed a strong evolution of solubility not only with the nature of the precipitate and the Ce content, but also with the nitric acid concentration.
1. Introduction
There is an increasing interest in study of the transition metal molybdate (AMoO4, A = Co, Ni, Cu…) [Citation1] or rare earth molybdate (R2(MoO4)3, R = Y, Sc,…) [Citation2] compounds due to their promising technological importance for potential applications in the fields of batteries [Citation3], photocatalytic applications [Citation4,Citation5] or ceramic materials to immobilize radioactive waste [Citation6]. In this last context, zirconium molybdate compound can be found during the head-end steps of spent nuclear fuel reprocessing [Citation7–Citation10]. This solid, mainly composed of dissolution fines and metal debris from cladding, and agglomerated with zirconium molybdate, is well-documented in the literature [Citation11]. Published data mainly concern the crystalline precipitate of zirconium molybdate oxide hydrate [Citation12,Citation13], based on the precipitation of the fission products Mo(VI) and Zr(IV) in the fuel dissolution solution, with the formula Mo2ZrO7(OH)2, 2H2O, hereafter referred to as ZM. ZM forms in 3–3.5 mol/L nitric acid solution at 90–100°C and can adhere to surfaces, leading to equipment fouling [Citation14]. Considerable efforts have been made to characterize this precipitate [Citation15] and to understand its formation and dissolution mechanism [Citation16–Citation19], allowing to limit precipitate formation in industrial conditions and operate cleaning campaign of the dissolver equipment.
So far, very few studies have investigated the ability of ZM compounds to incorporate actinide cations such as Pu, Np or other fission products such as Cs. In an attempt to determine the Pu incorporation mechanism, Lloyd [Citation20] studied plutonium losses associated with ZM precipitation and plutonium molybdate precipitation. Fellows [Citation21] and Penneman [Citation22] reported the formation of a ZM with 2–3 wt% of plutonium from Pu-containing solutions. More recently, Cross [Citation23] determined the crystallographic structure of cesium plutonium molybdate. Nevertheless the lack of more available source data suggests the need for further research to gain a better understanding of this specific type of compound.
Surrogate non-radioactive elements, are commonly used to replace radionuclides which present greater issues in research experiments [Citation24]. The substitution of Ce(IV) for Pu(IV) is particularly common. Based on nearly identical ionic radii and a similar valence charge [Citation25], Cross [Citation23] has used this element in studies on plutonium molybdate, although finally he found some differences in the crystallographic structures.
Today, with the development of the fourth generation nuclear reactors, the spent fuel from sodium-cooled fast reactors (SFR) will result in fuel dissolution solutions with higher concentrations of fission products and plutonium. The solution parameters (such as acidity or the Pu/U ratio) are therefore going to change during this fuel’s recycling. It is thus necessary to explore a larger domain of plutonium and fission product concentrations, as well as acid concentrations, in order to ensure that actinide inclusion in the molybdate structure remains under control in future parameter evolutions during this recycling process.
In this study, in order to target subsequent acquisitions with radioactive elements, a full range of non-radioactive ZM precipitates, formed from simulated solutions containing Mo(VI), Zr(IV) and Ce(IV) under different initial conditions, was investigated on the basis of solution composition and of Mo/(Zr+ Ce) ratios at equilibrium. The solutions were characterized using XRD, SEM and elemental analysis. Mixed zirconium-cerium molybdate solubility was thoroughly examined according to the molar fraction of Ce(IV) and nitric acid concentrations.
2. Experimental
2.1. Chemicals
All chemicals were of analytical grade and were obtained from VWR: HNO3 68%, ammonium heptamolybdate tetrahydrate (NH4)6Mo7O24,4H2O Mw = 1235.85 g.mol−1, ammonium Ce(IV) nitrate (NH4)2CeIV(NO3)6 Mw = 548.23 g.mol−1 and Alfa Aesar: zirconium carbonate basic hydrate, Zr(OH)2CO3.ZrO2 Mw = 308.47 g.mol−1.
2.2. Preparation of precipitation solutions
In a nitric acid solution, ZM is produced by the reaction between the molybdate anion Mo(VI) and the zirconium cation Zr(IV). It is possible to include by substitution of Zr(IV) other elements, such as Ce(IV), in the ZM crystal, in order to precipitate mixed zirconium-cerium molybdate. Precipitations were run in a glass reactor with a 100 mL volume. Mo, Zr and Ce(IV) were placed in solution before being heated to 92°C. The solution was maintained under reflux for 5 h, but the first signs of precipitation occurred at 70°C.
2.3. Synthesis
Zirconium, cerium and molybdenum stock solutions were mixed together at room temperature to give simulant solutions. Mixture were then heated up to and maintained at 92°C and were used to study the formation of mixed zirconium/cerium molybdates at different molar ratios of cerium.
The first step of this study started with the synthesis of several ZM precipitates in 3 mol.L−1 HNO3 containing different Ce(IV) molar fractions, expressed as [Ce(IV)]/([Ce(IV)]+[Zr(IV)]), between 0 and 100%. The Mo and the [Zr+ Ce] concentrations are kept constant for all the precipitation tests, respectively at 0.1 mol.L−1 and 0.05 mol.L−1. Depending on the initial desired molar fraction of cerium in solution, the zirconium concentration is adjusted to keep the total [Zr + Ce] concentration constant.
The solids were filtered after 5 h of precipitation at 92°C and washed with a nitric acid solution (1 mol/L) and deionized water. Final precipitates were dried at room temperature and weighed after mass stabilization had been verified.
The determinations of the elemental mole ratios of the metal in the compounds were carried out by total dissolution of the precipitate in concentrated HNO3 solutions in the presence of H202. Mo, Zr and Ce concentrations were measured by ICP-AES. The cerium molar fraction in the solution at equilibrium and the cerium molar fraction in the precipitate were respectively noted XCe eq and XCe.
2.4. Solubility measurement
Solubility measurements were performed at 92°C by dissolution of the previously synthesized powders. The temperature was followed by a thermometer placed in the reactor (precision of ± 0.25°C). A 100 mg aliquot of ZM compound was added to a 70 mL nitric acid solution in a glass reactor. The solution stirring was maintained with a magnetic stirrer. A condenser was connected to the reactor to avoid solution evaporation. To obtain the equilibrium time for the system, an aliquot of the dissolution solution was sampled regularly through a 0.25 µm pore syringe filter, and the composition of the solution was analyzed by ICP-AES. When the dissolution solution composition no longer varied, it was assumed that the system had reached equilibrium. Experimental results indicated that 3 h were sufficient to reach an apparent equilibrium.
2.5. Solid characterization
The X-ray powder diffraction (XRD) diagrams were recorded by a Bruker D8-advance diffractometer using a copper anticathode. The powder sample was placed on a suitably adapted holder and analyzed during around 2 h. The diffractograms were collected from 5–60° (2θ). The unit cell parameters of ZM compounds were refined by pattern matching using the FullProf software [Citation26] or Topas software (Bruker).
SEM micrographs were obtained on a Zeiss SUPRA 55/55VP FEG-SEM apparatus. Samples were deposited on a carbon substrate, first bonded to the sample holder and then metallized with gold.
Lanthanide concentrations in solution were determined by ICP-AES using an Activa-M Horiba Jobin Yvon spectrometer.
3. Results and discussion
3.1. Precipitate composition depending on cerium concentration
shows the dependence of the cerium molar fraction in the solid (XCe) with the initial cerium molar fraction in solution (XCe eq) with the ZM precipitation.
Figure 1. Influence of cerium molar fraction in the solid XCe and in the solution X’Ce on the mixed cerium-zirconium molybdate precipitation.
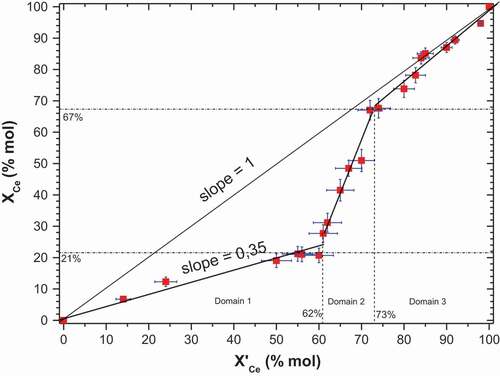
The first domain for the cerium molar fraction in the precipitation solution ranges from 0–62%. Elementary analyses showed that a precipitation plateau was reached for a maximum cerium molar fraction of 21% in the solid. Thereafter, these precipitates were cerium-depleted compared to their molar fractions in the solution.
The second domain was located in a transitional domain for cerium molar fractions between 62 and 73%. In this domain, a considerable increase of cerium molar fractions in the solid was observed between 21 and 67%.
The last part of the graph shows a strong trend to congruence as regards precipitation for cerium molar fractions in the solution between 73 and 100%.
Consequently, the first data collected from elementary analyses seems to show that the precipitation mechanism is different depending on the cerium molar fraction domain. Three domains are distinguished.
3.2. Molar ratio Mo/Zr+ Ce
presents the evolution of molar ratio Mo/(Zr+ Ce) for each precipitate depending on the cerium molar fraction in the solid. The molar stoichiometry ratio Mo/(Zr+ Ce) was calculated by using the Mo, Zr and Ce molar concentrations obtained by elementary analyses of the precipitate. The limits of the domain are surrounded by pure molybdate compounds. At 0% molar fraction, the compound is ZM, while at 100% molar fraction, the compound is Mo6Ce3O24(H2O)4. Pure zirconium molybdate has a theoretical molar ratio of 2, as does cerium molybdate. However, as can be seen in , an over-stoichiometry around 2.5 can be seen for the pure zirconium molybdate, and this ratio progressively decreases to 2 for the following mixed zirconium-cerium compound incorporating up to 45% cerium in the solid, for the precipitation conditions used here. From a 45% cerium molar fraction in the solid, the stoichiometry ratio is quite stable up to the pure cerium molybdate at a value around 2.
Figure 2. Plot of Mo/(Zr+ Ce) molar stoichiometry ratio depending on cerium molar fraction in the solid XCe..
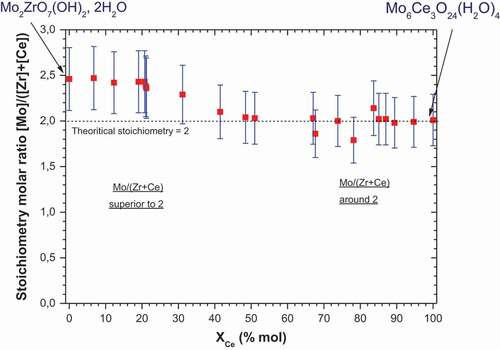
This over-stoichiometry can be explained by a very high kinetic rate caused by strong initial element concentrations in solution, which can induce molar ratio differences compared to a stoichiometric synthesis in less concentrated conditions. The over-stoichiometry could also be due to element (IV) substitution by molybdenum atoms and the creation of cationic vacancies.
3.3. Crystallographic analysis
The crystallographic study was carried out on each ZM precipitate in order to determine their crystalline structures and lattice parameters. refers to XRD patterns chosen among the 22 precipitate analyses, and showing the modification of their crystalline structure with the evolution of the cerium molar fraction in the solid.
Firstly, it is obvious that the structural properties of mixed zirconium-cerium molybdate evolve on the 0–100% cerium molar fraction domain.
At 0% mol Ce content, the pure zirconium molybdate ZM has a tetragonal structure (space group I41cd with a = b = 11,45 ± 0,01 Å, c = 12,49 ± 0,01 Å at 25°C), as determined by Clearfield and Blessing in 1972 [Citation13].
With increasing Ce(IV) content in the solid, the tetragonal structure accommodates Ce(IV) in substitution for Zr(IV) up to around 21%mol. shows the evolution of the peak position at 28.6° (which is characteristic of the tetragonal structure) depending on the cerium molar fraction in the solid. A shift is observed in the peak position towards lower angles when XCe increases from 0 to 21%. This proves the existence of a solid solution in this cerium molar fraction domain, which should be confirmed with a lattice parameter study through Vegard’s law determination [Citation27].
Figure 4. Peak position shifting with increasing cerium content, confirming the existence of a large solid solution domain for zirconium molybdate precipitates.
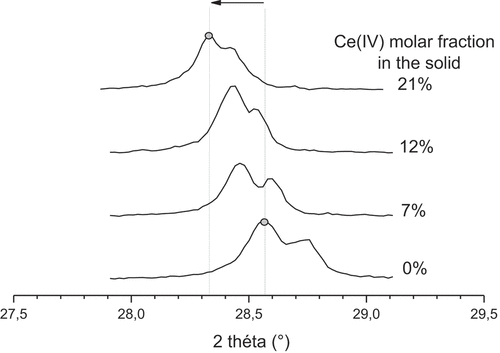
Concerning the pure cerium molybdate compound at 100% mol, in 1973 Brixner proposed a triclinic structure for this compound Ce(MoO4)2 (a = 10.15Å, b = 18.42Å, c = 9.86Å, α = 96.76°, β = 101.9°, γ = 103.96°) [Citation28], but our experimental diffractogram does not give a good match with his paper. Nevertheless, Cross [Citation23] proposed in 2013 the compound Mo6Ce3O24(H2O)4 with a monoclinic structure, which seems to be much more appropriate (space group C2/c with a = 12,7337(7)Å, b = 22,1309(16)Å, c = 7,8392(4)Å, β = 96,591(4)°, density 4.380g.cm−3).
With the increase of the Zr(IV) molar fraction in the solid, the monoclinic structure includes Zr(IV) ions up to 33% (i.e. XCe = 67%). Presence of a solid solution in the 67–100% domain is also to be expected.
For a cerium molar fraction in the solid between 21 and 67%, a mixture of tetragonal and monoclinic phases is visible on the patterns. The tetragonal phase seems to be the major phase, but no quantitative results can be given.
The evolution of the lattice parameters (a and c) of zirconium molybdate with the increase in the cerium molar fraction is linear, at least until 21% mol, as seen in the . This trend is characteristic of a zirconium molybdate solid solution where Ce(IV) replaces Zr(IV) without changing its crystalline structure. The Ce(IV) ion is more voluminous than the Zr(IV) ion (Shannon [Citation29] – ionic radius for n 8-coordination: 0.84 Å for Zr4+ and 0.97 Å for Ce4+) and thus an increase in the cerium content in the crystal leads to an increase in the lattice parameter. Moreover pure zirconium molybdate lattice parameters perfectly match with Clearfield’s study (a = 11.45 ± 0.01 Å, c = 12.49 ± 0.01Å).
Figure 5. Determination of Vegard’s law for mixed zirconium-cerium molybdate tetragonal solid solution, for a precipitation domain between 0 and 21% cerium molar fraction in the solid.
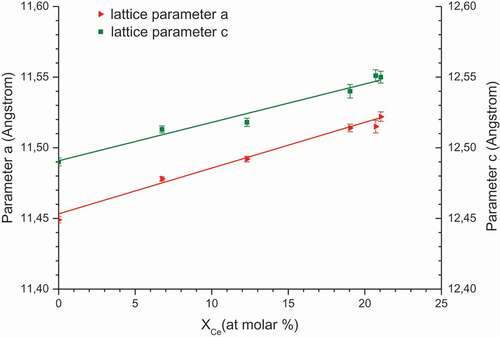
Similarly, a shift in peak position characteristic of the monoclinic structure (between 26.5 and 27°) can be observed towards the higher angles on when XCe decreases from 100 to 67%. In fact, in this domain, smaller Zr(IV) ions substitute for Ce(IV) in the crystal and consequently there is a linear decrease of the lattice parameters observed in the
Figure 6. Peak position shifting of four different precipitates with increasing cerium content, confirming the existence of a large solid solution domain.
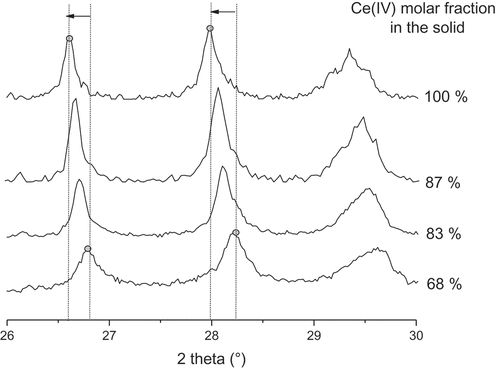
, proving the existence of a second solid solution. However lattice parameters for cerium molybdate domain were refined only from 100 to 85%mol, because of the difficulty of refining. The lattice parameters found for pure cerium molybdate in this study (a = 12.810(1)Å, b = 22.150(2)Å, c = 7.880(1)Å) do not exactly match those found by Cross (a = 12.7337(7)Å, b = 22.1309(16)Å, c = 7.8392(4)Å), but the values remain fairly close. The evolution of the lattice parameters (a,b and c) of cerium molybdate with the increase in the zirconium molar fraction is linear, at least until 78% mol, as seen in the . This trend is characteristic of a cerium molybdate solid solution where Zr(IV) replaces Ce(IV) without changing its crystalline structure. A detailed crystallographic work should be undertaken in future studies to analyze the cerium molybdate phase with more precision.
Figure 7. Determination of Vegard’s law for mixed zirconium-cerium molybdate monoclinic solid solution, for a precipitation domain between 85 and 100% cerium molar fraction in the solid.
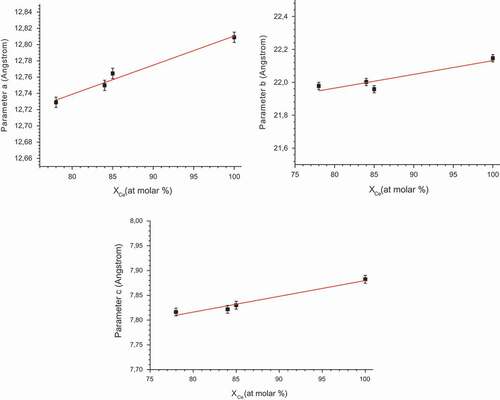
Consequently, this crystallographic study has permitted the presence of two different solid solutions to be highlighted: the tetragonal zirconium molybdate phase with an inclusion of Ce(IV) and the monoclinic cerium molybdate phase with an inclusion of Zr(IV). Nevertheless, these two solid solutions are separated by a gap, where a mixture of phases appears. It should be noted that the different domain limits are coherent with those pointed out by elementary analysis.
3.4. SEM characterization
presents SEM observations on pure and mixed zirconium-cerium molybdate precipitates. The pure ZM crystal (picture A) shows numerous parallelepiped crystals with sides of around 1.5 µm, with a tendency to grow onto the other crystals and to agglomerate. This picture is in agreement with the SEM images presented by Usami [Citation19] and Magnaldo [Citation17]. The cerium inclusion effect appearing on picture B is due to strictly cubic crystals being less and less present. At a 12% cerium molar fraction, the crystals are replaced by more curved and smaller nodules.
Figure 8. SEM observations carried out on A) pure zirconium molybdate, B) mixed zirconium-cerium molybdate at 12%Ce, C) mixed zirconium-cerium molybdate at 67%Ce, D) and E) mixed zirconium-cerium molybdate at 83%Ce, F) pure cerium molybdate.
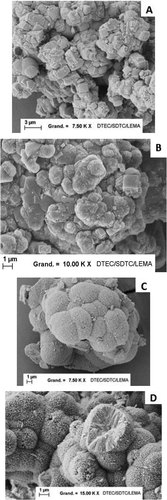
The monoclinic phase has a totally different morphology. Picture F shows a pure cerium molybdate phase with a 1.5 µm long stick structure, resembling a sea anemone. When the Zr content in the solid increases, the sticks are highly compressed and look more like a sphere. The zoom shown in picture D shows a half-destroyed sphere, with sticks starting from the center of the sphere and growing towards the outside. The last picture, C, shows a solid with 67% cerium, with the spheres seeming very compact and agglomerated. It can be deduced that a Zr content increase in a cerium molybdate compound changes the morphology from sticks to a compacted sphere structure.
3.5. Solubility study of mixed zirconium-cerium molybdates
Solubility (noted s) is defined as the dissolved precipitate concentration at the thermodynamic equilibrium, which means the concentration in a saturated solution. It is determined from ion concentration analysis of the solid at the equilibrium.
In this study, for mixed zirconium-cerium molybdate whose general formula is Mo2Zr1-xCexO7(OH)2, the solubility is noted:
It was chosen to use the solubility expression which involves the Mo concentration. Nevertheless as described previously, a Mo over-stoichiometry has been highlighted and consequently the solubility formula should take care to integrate the experimental stoichiometry ratio (shown in ) instead of the theoretical value of 2.
presents the solubilities for mixed zirconium-cerium molybdate compounds with different cerium molar fractions in the solid and for different nitric acid concentrations (3, 4 and 5.5 mol/L) obtained at 92°C.
Figure 9. Mixed zirconium-cerium molybdate solubility depending on the cerium molar fraction in the solid for three different nitric acid concentrations: 3 mol/L (blue), 4 mol/L (green), 5.5 mol/L (red).
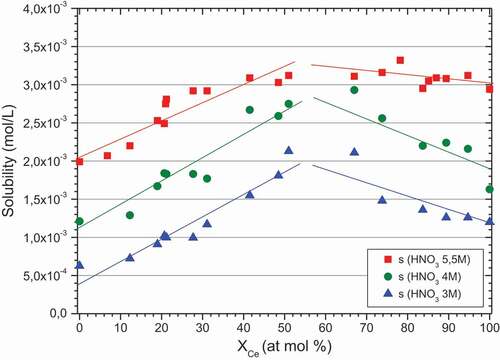
The solubility of end-members (0% and 100%) are not the same at a given acidity: as it can be seen for the three different acidities, cerium molybdate is always more soluble than zirconium molybdate. Moreover, the solubility of zirconium molybdate (0%) at 5.5 mol/L nitric acid concentration (2.10−3M) is 3.3 times higher than at 3 mol/L (6.10−4M). For cerium molybdate, its solubility between 3 and 5.5 nitric acid concentration increases by a ratio of 2.3. Thus increasing the acidity of the dissolution media increases the end-member solubility.
Concerning solubility modification with the cerium content in the solid, two trends clearly appear. First of all, an increase in solubility is highlighted when the cerium content in the solid increases, at least up to the end of the mixed phase (around 50%). However, when the crystalline structure changes to become the monoclinic phase, the solubility of the mixed zirconium-cerium molybdate decreases up to the pure cerium end-member. This phenomenon is observed for the three different acidities.
This parametric study, performed at different cerium fractions, shows the effect of crystalline structure modification on the solubility but also points out the effect of the acidity on all the mixed molybdate formation domains.
4. Conclusion
During the dissolution of spent nuclear fuel, adverse precipitation involving fission products such as molybdenum and zirconium may occur and are described worldwide as a major concern. In fact, zirconium molybdate compounds are able to include fractions of +(IV) elements (such as Pu(IV)) by substitution of Zr(IV). Understanding of the precipitation mechanisms and appropriate management of the radioactive precipitate require a detailed knowledge of the solid phases formed. This work was aimed at deepening the structural study of the mixed zirconium and cerium molybdates in order to take into account the possible influence of tetravalent elements on the composition of the precipitates.
The structural study was conducted by precipitation of a wide range of mixed zirconium-cerium molybdate where cerium can be considered as a chemical analogue of plutonium. The solid composition strongly depends on the tetravalent element initially contained in solution.
Lattice parameters and crystallographic structure were determined for each precipitate by XRD. The results have shown the predominance of two phases that are ZrMo2O7(OH)2(H2O)2 and Ce3Mo6O24(H2O)4. Each of these compounds was proved being a solid solution within which the ZrIV and CeIV atoms can substitute each other. SEM micrographs also confirmed the presence of two different crystal morphologies depending on the solid solution domains. Moreover, elemental analysis also proved the existence of an over-stoichiometry ratio Mo/(Zr+ Ce) higher than the theoretical ratio of 2 in the tetragonal domain, and whose origin has not yet been determined.
Finally, solubility data on mixed zirconium-cerium molybdate have shown a parabolic evolution depending on the two solid solution domains. The influence of nitric acid concentration in the dissolution media was also investigated showing a solubility increase at high acidity. From an industrial point of view, the preliminary results of this study show the advantage of increased acidity on molybdate solubility, which can be a way to avoid the fouling process. These results should soon be validated by targeted zirconium molybdate syntheses with zirconium substitution by plutonium.
Acknowledgments
This research was financially supported by AREVA NC, France. We thank Anne Tribout-Maurizi and Emilie Bossé, AREVA NC, France, for encouraging this work and for fruitful discussions.
Thanks must also be addressed to Serge Caron (DEN/MAR/DTEC/SDTC/LEMA) for the SEM observations.
Disclosure statement
No potential conflict of interest was reported by the authors.
References
- Sleight AW, Chamberland BL. Transition metal molybdates of the type AMoO4. Inorg Chem. 1968;7(8):1672–1675.
- Brixner LH, Berkley JR, Jeitschko W. Chapter 30 Rare earth molybdates (VI). Vol. 3. In: Handbook on the physics and chemistry of rare earths. Elsevier; 1979. p. 609–654. doi:https://doi.org/10.1080/00223131.2018.1492468
- Begam KM, Michael MS, Prabaharan SRS. Enhanced cycling properties of transition metal molybdates, Li x M2(MoO4)3 {0 ≤ x < 3} [M = Co,Ni]: a nanocomposite approach for lithium batteries. Ionics. 2007;13:467–471.
- Namvar F, Beshkar F, Salavati-Niasari M, et al. Mater Sci: Mater Electron. 2017;28:7962–7968.
- Segundo Sena M, Medeiros de Santana e Silva M, Gomes Dos Santos A, et al. Synthesis and characterization of cerium molybdate semiconductor nanoparticles. Mater Res. 2017;20:485–491.
- Vereshchagina TA, Fomenko EV, Vasilieva NG, et al. A novel layered zirconium molybdate as a precursor to a ceramic zirconomolybdate host for lanthanide bearing radioactive waste. J Mater Chem. 2011;21:12001.
- Kubota M, Fukase T. Formation of precipitate in high-level liquid waste from nuclear fuel reprocessing. J Nucl Sci Technol. 1980;17:783–790.
- Izumida T, Kawamura F. Precipitates formation behavior in simulated high level liquid waste of fuel reprocessing. J Nucl Sci Technol. 1990;27:267–274.
- Kondo Y, Kubota M. Formation and filtration characteristics of solids generated in a high level liquid waste treatment process. J Radioanalytical Nucl Chem. 1997;221:45–52.
- Bossé E, Tribout-Maurizi A, Cochin F, et al. 2012. Improvement of performances in La Hague head facility. In: E. Bossé, A. Tribout-Maurizi, F. Cochin, C. Quenault (AREVA RecyclingBU), P. Blanc (CEA DEN/DRCP, Marcoule), E. Delisle (AREVA E&P, St Quentin-en-Yvelines); ATALANTE 2012; 2012 Sep 6; Montpellier.
- Pradel P. Treatment and recycling of spent nuclear fuel: actinide partitioning - Application to waste management. Saclay (France): CEA and Editions du Moniteur; 2008. 177 p.
- Khonina IV, Lumpov AA, Shadrin AY, et al. Formation of molybdenum and zirconium precipitates in concentrated uranyl nitrate solutions. Radiochemistry. 2010;52:175–179.
- Clearfield A, Blessing RH. The preparation and crystal structure of a basic zirconium molybdate and its relationship to ion exchange gels. J Inorg Nucl Chem. 1972;34:2643–2663.
- Zhang L, Takeuchi M, Koizumi T, et al. Evaluation of precipitation behavior of zirconium molybdate hydrate. Front Chem Sci Eng. 2013;7:65–71.
- Paul N, Biggs S, Edmondson M, et al. Characterising highly active nuclear waste simulants. Chem Eng Res Des. 2013;91:742–751.
- Doucet FJ, Goddard DT, Taylor CM, et al. The formation of hydrated zirconium molybdate in simulated spent nuclear fuel reprocessing solutions. Phys Chem Chem Phys. 2002;4:3491–3499.
- Magnaldo A, Masson M, Champion R. Nucleation and crystal growth of zirconium molybdate hydrate in nitric acid. Chem Eng Sci. 2007;62:766–774.
- Esbelin E, Gareil P, Masson M, et al. Determination of Mo(VI)–Zr(IV) interaction parameters in highly acidic solutions by UV absorbance spectrophotometry. Anal Chim Acta. 2001;442:171–182.
- Usami T, Tsukada T, Inoue T, et al. Formation of zirconium molybdate sludge from an irradiated fuel and its dissolution into mixture of nitric acid and hydrogen peroxide. J Nucl Mater. 2010;402:130–135.
- Lloyd MH Proc. top. meet. plutonium fuel cycle; 1977: Paper VI. 5.
- Fellows RL, Newman DH, Lloyd MH. Consolidated fuel reprocessing program: plutonium losses due to solution instability and solids formation. 1978. Chapter 2.1.4. ORNL / TM-6836 (Oak Ridge National Laboratory); 1979 Jan 1–Mar 31.
- Penneman RA, Haire RG, Lloyd MH. In actinide separations: polymolybdates as plutonium (IV) hosts. Am Chem Soc. 1980;117:571–581.
- Cross JN, Duncan PM, Villa EM, et al. From yellow to black: dramatic changes between cerium(IV) and plutonium(IV) molybdates. J Am Chem Soc. 2013;135:2769–2775.
- Seaborg GT. Radiochim Acta. 1993;61:115.
- Kim CW, Wronkiewicz DJ, Finch RJ, et al. Incorporation of cerium and neodymium in uranyl phases. J Nucl Mater. 2006;353:147–157.
- Rodriguez-Carvajal J, Fernandez-Diaz MT, Martinez JL. J Phys: Condensed Matter. 1991;3:3215.
- Denton AR, Ashcroft NW. Vegard’s law. Phys Rev A. 1991;43:3161.
- Brixner LH, Whitney JF, Licis Kay MS. Lattice parameters and space group of Ce(MoO4)2. J Solid State Chem. 1973;6:550–552.
- Shannon R. Revised effective ionic radii and systematic studies of interatomic distances in halides and chalcogenides. Acta Crystallographica Section A. 1976;32:751–767.