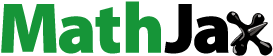
ABSTRACT
The extraction of Ce(III) nitrate has been investigated by using N, N′-dimethyl-N, N′-dioctyl-diglycolamide (DMDODGA = L) as extractant with 1-Butyl-3-methylimidazolium hexafluorophosphate ([Bmim][PF6]) as diluent in comparison with 40% octanol/kerosene. DMDODGA in two different diluents shows good extraction ability for Ce(III), but the trends are quite different depending on the concentrations of the ligand in solvent phase and nitric acid in aqueous phase. The slope analysis method is used to obtain the stoichiometric ratio of the extracted complexes. 1:3 complex of Ce(III) to DMDODGA may be formed in both systems, while 1:2 complex may be also formed in DMDODGA-40% octanol/kerosene system. The extraction of Ce(III) is by the cation exchange mechanism in DMDODGA-[Bmim][PF6] system. Unlike the expected positively charged 1:3 complex of Ce(III) formed in the DMDODGA-40% octanol/kerosene system, the 1:2 complex is assumed to be a neutral complex with two DMDODGA molecules bonding to Ce(III). In addition, the stronger interaction between the C = O groups of L and Ce(III) in the DMDODGA-[Bmim][PF6] system than in the DMDODGA-40% octanol/kerosene system is confirmed by IR spectroscopy.
1 Introduction
Nuclear waste is one of the most important concerns about the rapidly increasing utilization of nuclear energy. The major high-level radioactive nuclear waste is generated from spent nuclear fuel reprocessing. Typical spent nuclear fuel discharged from light water cooled reactors consists of mainly unused uranium (~96%), fission products (3%), plutonium (1%), and very small amount of minor actinides (Ans). Among the fission products, lanthanides (Lns) are a major component and a very harmful neutron poison, and their accumulation in nuclear fuel interferes with the nuclear chain reaction. The minor actinides contribute to long-term radiotoxicity of nuclear waste. The development of advanced nuclear fuel recycling would improve the utilization of the limited uranium resource by recovering the unused uranium and the by-produced plutonium to make new fuel. Furthermore, the separation of Lns and Ans would reduce the volume and toxicity of the nuclear waste and save the very costly capacity of geological repository [Citation1].
The separation of Lns and actinides from the high-level radioactive waste has been an important research area in developing advanced nuclear fuel recycling system. Ionic liquids (ILs) have unique properties, such as low vapor pressure, nonflammability, high thermal stability, high radiation stability, wide liquid range, wide electrical window, and tunable hydrophobicity, which is regarded as a new generation of green solvents in spent nuclear fuel reprocessing [Citation2]. Compared with the limited extractability [Citation2,Citation3], ILs have been considered as good diluent to replace the organic solvents used in traditional solvent extraction systems of spent fuel reprocessing [Citation4–Citation6].
ILs are composed of cations and anions, and their melting points are generally below 100°C [Citation7]. Wilkes et al. developed water-stable ILs composed of organic cations and inorganic or organic anions [Citation8]. Since their inception, these ILs have been studied widely because of their stability in air and water. For instance, [C4mim][PF6] was used in extraction of benzene derivatives in 1998 [Citation9] and very high extraction efficiency was observed in an ILs-based extraction [Citation10]. Cation exchange mechanism in ILs was proved for the extraction of metal ions in some systems using ILs, such as the extraction mechanisms of Pu(IV) by BenzoDODA in [C8mim][Tf2N] [Citation11] and TBP in [Cnmim][Tf2N] (n = 4, 6, 8) [Citation12], Sc(III) by Cyanex 935 in [C8mim][PF6] [Citation4], X(III)(X = Ce, Eu, Y) by CMPO in [Bmim][PF6] [Citation5], Eu(III) and Y(III) by DODGAA in [Cnmim][Tf2N] (n = 4, 8, 12) [Citation6].
During the past decade, diglycolamides (DGAs, containing ether linkages between two amide groups) have received particular attention in Lns and Ans partitioning [Citation13]. DGAs not only have extraction capability and selectivity (high affinity for trivalent metal ions compared to hexavalent metal ions), but can also be completely incinerated (its secondary waste was mostly none). The extraction behaviors of Ans and Lns using DGAs with different alkyl groups have been studied to some extent [Citation14]. The extraction of metal ions including uranium nitrate with N, N’-dimethyl-N, N’-didodecyl-diglycolamide (DMDDDGA) [Citation15], strontium with N, N, N’ N’-tetra-isobutyl diglycolamide (TiBDGA) [Citation16], 233U(IV), 241Am(III), (85+89)Sr(II) with DEHDODGA in n-dodecane [Citation17], americium with TODGA and TEHDGA in HNO3, HClO4, and HCl [Citation18] have been investigated by research groups in countries around the world. Additionally, the extraction behavior and mechanism of HNO3 with various DGAs have been studied in varying diluents, such as TEHDGA [Citation18], TODGA [Citation19], DMDODGA in chloroform [Citation20] and 40% octanol/kerosene [Citation21].
In last a few years, an unsymmetric DGA, N, N’-dimethyl-N, N’-dioctyl-diglycolamide (DMDODGA = L) (the structure showing in ) has been paid more attention. The extracted complexes of some Ln(III) ions and Am(III) with L-40% octanol/kerosene system have been investigated by correlating their fluorescence/absorption spectra with those of some structurally related complexes. The formation of 1:3 extracted complexes of Ln(III) ions and Am(III) were confirmed in the L-40% octanol/kerosene system [Citation22,Citation23]. The extraction of Lns nitrates with L in chloroform presented different trends for light and heavy Ln(III) ions depending on the nitric acid concentration [Citation20]. [Bmim][PF6] (the structure showing in ) has shown excellent solubility to organic substances, excellent hydrophobicity and radiation stability [Citation24]. The main physical characteristics are summarized as follows: the melting point Tf = 6.5°C; the boiling point Tb = 340°C; the density ρ = 1.38 g/mL (20°C). To further get insight into the extraction of Lns and Ans using L as extractant, we start here with the extraction of Ce(III) using [Bmim][PF6] as diluent compared with 40% octanol/kerosene. The purpose of choosing Ce(III) as the subject is twofold. At first, Ce has a relatively high concentration in the high-level radioactive waste, which will cause more trouble in the high-level radioactive waste disposal. Secondly, Ce is the second element in the 4f series, which could be a good representative of the fission products of light Lns in the spent fuel.
2 Experimental
2.1 Material
DMDODGA was supplied by China Institute of Atomic Energy with the purity above 98%. All other reagents used were of A. R. Grade purchased from Sinopharm Chemical Reagent Co. Ltd (China) and used without further purification.
2.2 Methods
The extraction phase and the water phase were mixed with Whirlpool mixer (QL-861, Qilinbeier, China) for 7 min at 25 ± 0.5°C. Then the mixture was settled and centrifuged at 3500 rpm for 8 min with a centrifuge (LG16B, Leiboer, China). The initial Ce(III) concentration in all the aqueous solutions was 3.4 mM. After phase separation with centrifugation, an aliquot of raffinate was taken from the aqueous phase, and the concentration of Ce(III) was determined with inductively coupled plasma mass spectrometry (ICP-MS, X-II, Thermo Fisher Scientific, USA). IR spectra of the extracted complexes were recorded with resolution of 4 cm−1 and scan-time of 32 on a Fourier Transform Infrared Spectrometer (FTIR, WQF-510A, Reili, Beijing, China). The samples were prepared as liquid film on KBr plates.
The volume fraction of L in the ILs phase was 1% (0.024 M), except for the experiments involving the dependence of the extraction on L concentration. The volume fraction of L in the organic phase was 4% (0.106 M) with exception for examining the dependence of the extraction on the L concentration. The HNO3 concentration was 1 M in all experiments, except for observing the effect of HNO3 concentration.
The distribution ratio, DCe, is defined and calculated from the following Equationequation (1)(1)
(1) :
where Cini is the initial Ce(III) concentration in the aqueous phase; Caq and Co are the Ce(III) concentrations in aqueous and extraction phase (ILs phase or organic phase) after the extraction, respectively; Vaq and Vo represent the volumes of extraction phase (L in ILs or 40% octanol/kerosene). In this paper, the extraction experiments were conducted by contacting 1 mL of ILs phase with 4 mL of aqueous phase (phase ratio: Vo: Vaq = 1:4), or by contacting 1 mL of organic phase with 2 mL of aqueous phase (phase ratio: Vo: Vaq = 1:2).
3. Results and discussion
3.1 Effect of nitric acid concentration
As shown in , DCe significantly depends on the acid concentration in aqueous solution for both systems. The trend of DCe changing with the nitric acid concentration is similar as the results of Lns extraction by DGAs in other imidazolium-based ILs [Citation14]. Briefly, DCe at low acid concentrations is much higher than that at high acid concentrations for L-[Bmim][PF6] system (black circles in ). It sharply drops about two orders of magnitude as the acid concentration increases from 0.1 to 0.5 M, and then it decreases insignificantly and reaches the lowest point while HNO3 concentration increases to 4 M. As the concentration of HNO3 increases to more than 4 M, DCe increases slowly with the increasing acid concentration. In contrast, for the L-40% octanol/kerosene system DCe first increases then decreases with the increasing acid concentration (black squares in ), and the maximum value is observed at acid concentration of about 2 M HNO3. The results show that [Bmim][PF6] can obviously enhance the extractability of L compared to that of using 40% octanol/kerosene as diluent. This could be attributed to extraordinary solvating capability [Citation11] of ILs as a polar molecule in organic phase, which seems to facilitate the metal ion mass transfer. In addition, there is a low and steady DCe with increasing HNO3 concentration in pure [Bmim][PF6] system (black triangles in ). Thus, the contribution from [Bmim][PF6] is much smaller than the contribution from L to the Ce(III) extraction.
3.2 Effect of initial [Bmim]+, H +, and NO3− concentration
It is well known that for most of the cases, the extraction of metal ions using ILs as diluent could be explained by the ion-exchange mechanisms [Citation7]. In order to verify the cation exchange mechanism, [Bmim]Cl and NaCl were added into the aqueous phase for the L-[Bmim][PF6] system to provide extra changeable cation. As shown in , DCe decreases with the increase of [Bmim]Cl concentration in the aqueous phase, but it does not change significantly while NaCl is added instead of [Bmim]Cl. The increasing [Bmim]+ in the aqueous solution make the exchange process of Ce(III) and [Bmim]+ more difficult between the two phases. In addition, it was found that in the ILs phase there was no nitrate after the extraction. These results clearly indicate that the extraction is going through the cation exchange mechanism. The extraction equation of Ce(III) from HNO3 solution with L-[Bmim][PF6] system can be described as the following Equationequation (2)(2)
(2) :
Figure 3. Effect of the initial concentrations of [Bmim]Cl and NaCl on the extraction of Ce(III) by L-[Bmim][PF6] system. ([L]ILs = 0.030 M, [Ce(III)]aq = 3.4 × 10–3 M, [HNO3] = 1 M).
![Figure 3. Effect of the initial concentrations of [Bmim]Cl and NaCl on the extraction of Ce(III) by L-[Bmim][PF6] system. ([L]ILs = 0.030 M, [Ce(III)]aq = 3.4 × 10–3 M, [HNO3] = 1 M).](/cms/asset/f3e63a0e-6a00-4f14-b66e-eff9114155d6/tnst_a_1604270_f0003_b.gif)
Moreover, the effect of the H+ and NO3− concentration on the extraction of Ce(III) by L-40% octanol/kerosene system was studied in . The concentration of H+ or NO3− was maintained at 2 M by HNO3 and NaNO3. By the comparison of the two curves in , DCe increases with the decreasing of H+ concentration and increasing of NO3− concentration. It indicates that: at lower acidity, the salting-out effect of NO3− can promote and dominate the extraction of Ce(III); at higher acidity, the competitive extraction of H+ dominates and decreases the extraction of Ce(III), by H+ integrating with L to decrease of the free L concentration in the organic phase. However, in our previous study, we found that in L-ILs system, NO3− doesn’t participate in the extraction process as a salting-out agent, but H+ will compete with metal ions to exchange with imidazolium cation when ILs as diluent [Citation21]. The counterion is NO3− in the L-40% octanol/kerosene system, but the counterion is the anion of ILs in the L-ILs system. This clearly showed that the mechanism of Ce(III) extraction by L in L-[Bmim][PF6] system is completely different compared to L-40% octanol/kerosene system, which may be mainly attributed to that the type of diluent would affect the combination of counterion with complex.
3.3 Dependency of Ce(iii) extraction on DMDODGA concentration
Based on the cation exchange mechanism, the number of L molecules in each extracted Ce(III) complex could be obtained with slope analysis method.
According to Equationequation (2)(2)
(2) , the conditional extraction constant (Kex) is given as shown in the following Equationequation (3)
(3)
(3) :
From Equationequations (1)(1)
(1) and (Equation3
(3)
(3) ), the relationship between DCe and Kex is described as the following Equationequation (4)
(4)
(4) and (Equation5
(5)
(5) ):
The free L concentration in the ILs phase is given as shown in the following Equationequation (6)(6)
(6) :
where [Ce3+]aq and [Bmim+]aq are the concentration of Ce(III) and [Bmim]+ in the extraction raffinate, respectively; [Ce3+]ILs and [Bmim+]ILs are the concentration of Ce(III) and [Bmim]+ in the ILs phase, respectively; [L]ILs and [L]ini represent the concentration of free L and initial L, respectively; [L-HNO3]ILs and [Ce3+·nL]ILs represent the concentration of and the L-HNO3 and Ce(III)-L complex in the ILs phase, respectively. And ‘n’ denotes the number of L molecules bonding to Ce(III) in the complex.
shows the dependency of Ce(III) extraction on the concentration of L in L-[Bmim][PF6] system and L-40% octanol/kerosene system. The plot of logDCe against log[L]ILs gives a straight line of slope 2.8, very close to 3, it is indicated that there is one Ce(III) extracted and complex formed in the ILs phase under the experimental conditions. The plot fitted by straight line with a slope of close to 3 should originate from the 1:3 complex, CeL33+, formed in the region of relatively low Ce(III) but high L concentrations. Accordingly, the extraction equation of Ce(III) in the L-[Bmim][PF6] system could be represented by following Equationequation (7)(7)
(7) :
Figure 5. Slope analysis of Ce(III) extraction as a function of the concentration of L-[Bmim][PF6] system and L-40% octanol/kerosene system. ([Ce(III)]aq = 3.4 × 10–3 M, [HNO3] = 1 M).
![Figure 5. Slope analysis of Ce(III) extraction as a function of the concentration of L-[Bmim][PF6] system and L-40% octanol/kerosene system. ([Ce(III)]aq = 3.4 × 10–3 M, [HNO3] = 1 M).](/cms/asset/f6fd6d58-f5cf-4ce6-952c-497bd3fc2355/tnst_a_1604270_f0005_b.gif)
Using similar procedure, the dependency of Ce(III) extraction in the L-40% octanol/kerosene system on L concentration was studied too. Like from the L-[Bmim][PF6] system giving a straight line, the logDCe against log[L] presents a straight line as well. The slope of 2.9, close to 3, indicates that only one Ce(III) complex species of 1:3 was formed in the L-40% octanol/kerosene system under the experimental conditions. Because in the organic phase there is no exchangeable cation, three NO3− have to be coextracted with Ce(III) into the organic phase to neutralize the positive charge of Ce(III). Hence, the extraction can be described as the following Equationequation (8)(8)
(8) :
3.4 Loading capacity
To obtain more information about the extracted complexes in these two systems, the loading capacity for Ce(III) was also examined. The extraction experiments for the loading capacity study were conducted by contacting 1 mL ILs phase with 4 mL aqueous phase (as shown in black circles in ) and 1 mL organic phase with 2 mL aqueous phase (as shown in black squares in ), respectively. After the extraction and phase separation, the aqueous phase was taken out, and the extraction phase was contacted with another aliquot of fresh aqueous phase. The performance was repeated several times until the concentration of Ce(III) in the extraction raffinate remained constant. The total Ce(III) finally loaded in the extraction phase was determined with ICP-MS after the samples were digested by nitric acid with microwave-oven. As shown in , the molar ratio of L to Ce(III) in [Bmim][PF6] gradually reaches constant about 3.1, which is consistent with the value of 3 for the extraction constant by the slope analysis in Equationequation (7)(7)
(7) . Compared with the slope analysis, 1:3 Ce(III) complex is not been influenced by the L saturation in [Bmim][PF6] system.
Figure 6. Ce(III) loading capacity of L-[Bmim][PF6] and L-40% octanol/kerosene system. (L-[Bmim][PF6] system: [L]ILs = 0.026 M, [Ce(III)]aq = 3.5 × 10–3 M, [HNO3] = 0.1 M; L-40% octanol/kerosene system: [L]o = 0.106 M, [Ce(III)]aq = 3.4 × 10–3 M, [HNO3] = 1 M).
![Figure 6. Ce(III) loading capacity of L-[Bmim][PF6] and L-40% octanol/kerosene system. (L-[Bmim][PF6] system: [L]ILs = 0.026 M, [Ce(III)]aq = 3.5 × 10–3 M, [HNO3] = 0.1 M; L-40% octanol/kerosene system: [L]o = 0.106 M, [Ce(III)]aq = 3.4 × 10–3 M, [HNO3] = 1 M).](/cms/asset/f9f9f95c-a41d-45f9-9a08-e3ac008e8953/tnst_a_1604270_f0006_b.gif)
Surprisingly, the molar ratio of L to Ce(III) in the L-40% octanol/kerosene system dropped to a relatively low value of 2.1, which is inconsistent with the result of forming 1:3 Ce(III) complex from the slope analysis under the conditions of low Ce(III) but high L concentrations. It should be pointed out that the number 2 for the molecules bonding directly to Ce(III) in the complex is different from most observation for Lns with similar DGAs ligands in various systems. Usually, 1:3 complex of Lns to DGAs were reported in previous studies in various systems. Taking the coordination number of Ce(III) varied from 9 to 12 into account, three L molecules coordinated to Ce(III) with three NO3− anions as counterion by Equationequation (8)(8)
(8) .
However, some unusual observation complexes were observed. One phenomenon of 1:1 complex was observed for the extraction of Pu(IV) with a DGAs ligand, N, N, N’, N’-tetra-isobutyl-diglycolamide (TiBDGA). In our previous study, we found that in TiBDGA-40% octanol/kerosene system, a neutral complex species of Pu(IV) was formed as Pu(NO3)4(TiBDGA) in which the four NO3− anions might directly bond to Pu(IV) as well as TiBDGA [Citation25]. Further, another similar phenomenon was observed for the extraction of Am(III) with a DGAs ligands, N, N, N’, N’-tetramethyl-3-oxa-gutaramide (TMOGA) [Citation23]. In the study, three successive complex species, 1:1, 1:2, and 1:3 Am(III)-TMOGA complexes, have been identified. And the ratio of Am(III) to TMOGA in the complex is determined by the relative molar concentration of Lns and ligand. Therefore, the unusual observation of the 1:2 complex of Ce(III)-L probably exist, which may be attributed to the excess of Ce(III) and relatively low L concentration in the extraction phase after several repeating extraction. These assumptions should be further verified with additional investigation using other helpful techniques.
3.5 IR spectra of the extracted complexes
To further elucidate the difference in forming complexes between the two systems, IR spectra of the complexes were collected in comparison with the extraction phase before Ce(III) extracted. The last samples for the loading capacity experiments were recorded and listed in . As shown in the spectra listed in , the most significant difference in the spectra is the shifting of the C = O stretching vibration band. The characteristic band at 1650 cm−1 for C = O of L is shifted to 1623 cm−1 while Ce(III) is loaded in the ILs phase (spectrum e), indicating there is a strong interaction between the C = O groups and Ce(III). For the L-40% octanol/kerosene system, with the loading of Ce(III), the peak at 1650 cm−1 becomes broad with a shoulder on the lower wave-number side. The difference in the shifting of the band at 1650 cm−1 in these two systems might have originated from the formation of the cationic complex species in the ILs phase and the neutral complex species in the organic phase. In the ionic phase, more negative charge is attracted to the oxygen atom of C = O by the positively charged Ce(III). However, in the organic phase, the charge interaction between the oxygen atoms of C = O groups and Ce(III) is much weaker, and much less negative charge is attracted to the oxygen atoms. Therefore, with the formation of the complex the shift of the band at 1650 cm−1 for C = O of L in the L-[Bmim][PF6] system is more significant than in the L-40% octanol/kerosene system. With more negative charge is attracted to the oxygen atom, the double C = O bond becomes weaker and the band shifts to lower wavenumber [Citation20].
Figure 7. IR spectra of different systems. (a) L; (b) Ce(III)-L in 40% octanol/kerosene system; (c) [Bmim][PF6]; (d) L-[Bmim][PF6]; and (e) Ce(III)-L-[Bmim][PF6]. ([L]ILs = 0.024 M, [Ce(III)]aq = 3.4 × 10–3 M, [HNO3] = 1 M).
![Figure 7. IR spectra of different systems. (a) L; (b) Ce(III)-L in 40% octanol/kerosene system; (c) [Bmim][PF6]; (d) L-[Bmim][PF6]; and (e) Ce(III)-L-[Bmim][PF6]. ([L]ILs = 0.024 M, [Ce(III)]aq = 3.4 × 10–3 M, [HNO3] = 1 M).](/cms/asset/0d0ea491-ffa2-4d6a-b2aa-fdd067e79fea/tnst_a_1604270_f0007_b.gif)
Based on all above observation, the extraction of Ce(III) with L-[Bmim][PF6] from aqueous solution of low nitric acid concentration is proposed and illustrated with .
4. Conclusion
The extraction of Ce(III) with L-[Bmim][PF6] system and L-40% octanol/kerosene system has been studied. The L extraction shows a strong ability for Ce (III) under appropriate conditions. At low nitric acid concentration, the extraction of Ce(III) undergoes the cation exchange mechanism with the number of 3 for L in the 1:3 complex in L-[Bmim][PF6] system. In contrast, the extraction of Ce(III) in the L-40% octanol/kerosene system is assumed to form same 1:3 complex under relatively low Ce(III) but high L concentration; and to form a neutral 1:2 complex under conditions of relatively high Ce(III) but low L concentration.
Interest statement
We declare that we have no competing interests.
Acknowledgements
This work was supported by the [National Natural Science Foundation of China under Grant] (Nos. 21876035, 51104050); [Natural Science Foundation of Heilongjiang Province] under Grant (No. E201709); [Scientific Research Foundation for the Returned Overseas Scholars of Heilongjiang Province] under Grant (No. 159150130002); and [Postdoctoral Scientific Research Developmental Fund of Heilongjiang Province] under Grant (LBH-3236310448); Spent Fuel Reprocessing Project.
Disclosure statement
No potential conflict of interest was reported by the authors.
References
- Silva RBE, Kazimi MS, Hejzlar P. Nuclear Fuel Recycling: national and Regional Options for the US Nuclear Energy System. Energy Environ Sci. 2010;3(8):996–1010.
- Zuo Y, Liu Y, Chen J, et al. The separation of cerium(IV) from nitric acid solutions containing thorium(IV) and Lanthanides(III) using pure [C8mim]PF6 as Extracting Phase. Ind Eng Chem Res. 2008;47(7):2349–2355.
- Nakashima K, Kubota F, Maruyama T, et al. Ionic liquids as a novel solvent for lanthanide extraction. Anal Sci Int J Jpn Soc Anal Chem. 2003;19(8):1097–1098.
- Sun X, Wu D, Chen J, et al. Separation of Scandium(III) from Lanthanides(III) with room temperature ionic liquid based extraction containing Cyanex 925. J Chem Technol Biotechnol Biotechnol. 2007;82(3):267–272.
- Nakashima K, Kubota F, Tatsuo M, et al. Feasibility of ionic liquids as alternative separation media for industrial solvent extraction processes. Chem Prod Process Model. 2015;44(12):4368–4372.
- Kubota F, Shimobori Y, Baba Y, et al. Application of ionic liquids to extraction separation of rare earth metals with an effective diglycol amic acid extractant. J Chem Eng Jpn. 2011;44(5):307–312.
- Sun X, Luo H, Dai S. Ionic liquids-based extraction: a promising strategy for the advanced nuclear fuel cycle. Chem Rev. 2012;112(4):2100–2128.
- Wilkes JS, Zaworotko MJ. ChemInform abstract: air and water stable 1- ethyl- 3-methylimidazolium based ionic liquids. Chem Rev. 1992;23(43):166.
- Huddleston JG, Rogers RD. Room temperature ionic liquids as novel media for ‘clean’ liquid–liquid extraction. Chem Comm. 1998;16(16):1765–1766.
- Dai S, Ju YH, Barnes CE. Solvent extraction of strontium nitrate by a crown ether using room-temperature ionic liquids†. JChem Soc Dalton Trans. 1999;8(8):1201–1202.
- Panja S, Ruhela R, Tripathi SC, et al. Effect of room temperature ionic liquid on the extraction behavior of plutonium (IV) using a novel reagent, Bis-(2-Ethylhexyl) carbamoyl methoxy phenoxy-Bis-(2-Ethylhexyl) acetamide [Benzodioxodiamide, BenzoDODA]. Sep Purif Technol. 2015;151:139–146.
- Panja S, Tripathi SC, Dhami PS, et al. Solvent extraction of Pu(IV) using TBP: a comparative study of n-dodecane and a room temperature ionic liquid. Sep Purif Technol. 2015;50(15):2335–2341.
- Ansari SA, Pathak P, Mohapatra PK, et al. Chemistry of diglycolamides: promising extractants for actinide partitioning. Chem Rev. 2012;112(3):1751–1772.
- Sasaki Y, Sugo Y, Suzuki S, et al. The novel extractants, diglycolamides, for the extraction of lanthanides and actinides in HNO3-n-DODECANE system. Solvent Extr Ion Exch. 2001;19(1):91–103.
- Sun G, Cai X, Han J, et al. Extraction of uranium nitrate by novel unsymmetrical N, N ’-Dimethyl- N, N ’-didodecyl diglycolamide. J Radioanal Nucl Chem. 2016;308(2):753–757.
- Sd D, Yang T, Liu N, et al. Extraction behavior of strontium from nitric acid solution with N, N, N′, N′-Tetraisobutyl Diglycolamide. J Iran Chem Soc. 2010;7(3):545–553.
- Ravi J, Suneesh AS, Prathibha T, et al. Extraction behavior of some actinides and fission products from nitric acid medium by a new unsymmetrical diglycolamide. Solvent Extr Ion Exch. 2011;29(1):86–105.
- Gujar RB, Ansari SA, Murali MS, et al. Comparative evaluation of two substituted diglycolamide extractants for ‘actinide partitioning’. J Radioanal Nucl Chem. 2010;284(2):377–385.
- Bell K, Geist F, Mclachlan F, et al. Nitric acid extraction into TODGA. Procedia Chem. 2012;7(1):152–159.
- Guoxin S, Min L, Yu C, et al. Synthesis of N, N′-Dimethyl-N, N′-Dioctyl-3-oxadiglycolamide and its extraction properties for lanthanides. Solvent Extr Ion Exch. 2010;28(4):482–494.
- Xu Y, Gao Y, Zhou Y, et al. Extraction behavior of strontium from nitric acid medium with N, N’-Dimethyl-N, N’-dioctyldiglcolamide. Solvent Extr Ion Exch. 2017;35(7):507–518.
- Kou F, Yang S, Qian H, et al. A fluorescence study on the complexation of Sm(III), Eu(III) and Tb(III) with tetraalkyldiglycolamides (TRDGA) in aqueous solution, in solid state, and in solvent extraction. Dalton Trans. 2016;45(46):18484–18493.
- Tian G, Shuh DK, Beavers CM, et al. A structural and spectrophotometric study on the complexation of Am(III) with TMOGA in comparison with the extracted complex of DMDOOGA. Dalton Trans. 2015;44(42):18469–18474.
- Shkrob IA, Marin TW, Chemerisov SD, et al. Radiation and radical chemistry of NO3−, HNO3, and dialkylphosphoric acids in room-temperature ionic liquids. J Phys Chem B. 2011;115(37):10927–10942.
- Tian G, Zhang P, Wang J, et al. Extraction of Actinide(III, IV, V, VI) Ions and TcOby N, N, N′, N′- Tetraisobutyl-3-Oxa-Glutaramide. Solvent Extr Ion Exch. 2005;23(5):631–643.