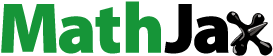
Abstract
This review systematically surveys the limiting apparent molar volume, limiting apparent molar volume of transfer, Hepler’s constant, hydration number, viscosity B-coefficients, and temperature derivative of B-coefficients, dB/dT of amino acids in aqueous solution of various drugs at different temperatures as these data are quite important for interpreting the hydration and other properties of proteins. The effect of drug on the volumetric and viscometric properties of amino acids has been discussed in terms of various physicochemical interactions, e.g. ion-ion, ion-hydrophilic, hydrophilic–hydrophilic, hydrophilic–hydrophobic and hydrophobic–hydrophobic interactions. These are also discussed in terms of structure-making/structure-breaking tendency of the amino acids in presence of various drugs. The comparison of these effects on the amino acid hydration enables us to have a better understanding of the influence of drugs on the protein stabilization.
GRAPHICAL ABSTRACT
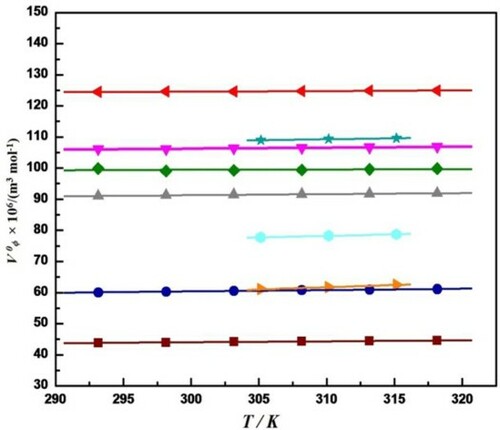
1. Introduction
Globular proteins form a class of macromolecules which have well defined physicochemical properties and functions in biological systems. They have a marginally stable native structure that results from a fine balance among various non-covalent forces: ionic and dipolar interactions, hydrogen bonding, hydrophobic forces, etc [Citation1,Citation2]. The process of denaturation of a globular protein in aqueous solutions involves a change from the native state, in which the protein adopts its characteristic folded conformation, to the denaturated state where the protein is predominantly in an extended form [Citation3,Citation4]. Upon unfolding, the interactions between protein groups within the core are disrupted and replaced with the interactions of these groups with the solvent, thus leading to change in protein solvation. The study of these protein solvent interactions is difficult because of the complexity of the interactions in such a large molecule. However, one useful approach, which can be helpful in understanding these interactions, is to study simple compounds such as amino acids, which model some specific aspects of the protein structure. The interactions between solvent and various constituent groups of proteins such as amino acid side chain and the peptide backbone group play a central role in the structure, the conformation and the function of proteins in aqueous solutions [Citation5,Citation6].
Drugs play an important role in human health and livestock rearing. Physicochemical properties of drugs are of great benefit to understand the drug action at the molecular level. The pharmadynamics and pharmakinetics such as transmission, diffusion, distribution and absorption of the drug in vivo, must be regarded as the ultimate consequence of physicochemical interactions between the drug and functionally important molecules in the living organism [Citation7]. The interactions may include ionic, covalent, charge transfer, hydrogen bonding, ion-dipole or hydrophobic ones [Citation8]. The drug interactions occurring outside the body are categorized as physical or chemical and may occur during formulation, storage as well as while mixing ingredients [Citation9]. Several experimental investigations have been carried out to understand the action of drugs in aqueous solution as they exert their activity by interaction with biological membrane [Citation10]. Moreover, the unabsorbed drug or its metabolites may enter the water via urine, fecal excretion, and so forth [Citation11], which will contaminate the water and soil. In biophysical chemistry, interaction of drug with different biologically important compounds is an important phenomenon involving complex mechanism [Citation12]. These interactions provide idea about the activity of drug in biological systems. A full knowledge of the mechanism of these interactions is required before the actual application of drugs in human body, and is also necessary for drawing some conclusion of drug’s delivery and physiological actions. The drugs that enter the human tend to stimulate certain receptors, ion channels, act on enzymes or transporter proteins. As a result, they cause the human body to react in a specific way. Once the receptors are activated, they either trigger a particular response directly on the body, or they trigger the release of hormones and/or other endogenous drugs in the body to stimulate a particular response. Drugs interact with receptors by bonding at specific binding sites. Most receptors are made up of proteins, and the drugs can, therefore, interact with the amino acids to change the conformation of the receptor proteins [Citation13,Citation14]. When certain molecules are added to protein solutions, they transmit stabilization or destabilization to the proteins [Citation15,Citation16]. Sometimes protein conformation is affected by these added co-solutes due to the solvent effects or their direct binding. However, due to the complicated structure of proteins containing many miscellaneous functional groups, the studies of protein-drug interactions are somewhat difficult. Therefore, for a better understanding of the behavior of proteins, a useful approach is to study simpler model compounds like amino acids and small peptides [Citation17–19]. The molecular interaction based upon thermodynamic study of drug-water system and its temperature dependence plays an important role in medicinal and pharmaceutical chemistry for understanding action, absorption and transport of drug across the biological membranes [Citation10].
The viscosity B-coefficients and standard partial molar volumes of amino acids in water at different temperature have been systematically reviewed by Hua Zhao. But to the best of our knowledge, no review on the volumetric and viscometric studies of amino acids in mixed solvent i.e. in aqueous solution of drug have been reported. Meanwhile, with the increasing application of drugs, studies on their binding with the receptor proteins, there is also an urgent need of summarizing the volumetric and viscometric properties of amino acids in various drugs, and interpreting their effect on the protein (enzyme) stabilization.
2.0. Volumetric properties
Volumetric properties are regarded as a sensitive tool for understanding the interactions in solutions. From the volumetric data, apparent molar volumes, limiting apparent molar volumes, transfer volumes at infinite dilution of solute from aqueous solutions to mixed aqueous solvent solutions, Hepler’s constant, hydration number, etc. at various temperatures can be calculated.
2.1. Limiting apparent molar volume
Limiting thermodynamic properties provide information about the solute-cosolute interaction, because at infinite dilution the interactions between individual solute molecules are negligible. Hence limiting apparent molar volume, V0ϕ which is the partial molar volume at infinite dilution is free from solute-solute interactions and provides valuable information about solute-cosolute interactions. The limiting apparent molar volume is calculated from apparent molar volume. Therefore apparent molar volume can be calculated using accurate density data through the following equation [Citation2]:
(1)
(1) where m is the molality (mol kg−1) of solute and M is the molar mass (kg mol−1) of solute, ρ0 and ρ are densities (kg m−3) of solvent and solution, respectively.
In the cases where the molality dependence of Vϕ was found to be either negligible or having no definite trend, the value of limiting apparent molar volume or partial molar volume at infinite dilution, V0ϕ, was evaluated by taking an average of all the data points. Limiting value of apparent molar volume can be calculated by least square fitting of apparent molar volume by following relation:
(2)
(2) where V0ϕ is the limiting apparent molar volume or partial molar volume at infinite dilution and Sv is the experimental slope which is considered to be a pair wise interaction, volumetric virial coefficient. The sign of Sν indicates the nature of the solute–solute interactions, while V0ϕ reflects the presence of solute–solvent interactions.
The above volumetric properties of amino acids in aqueous solution of various drugs have been investigated and reported by many researchers in the literature. In this project work, we are compiling the work in the form of review which have been reported by different authors for amino acids in various drugs in the literature.
By using Equation (2) many authors calculated limiting apparent molar volume for amino acids in different aqueous drugs at different temperatures. These values of limiting apparent molar volume for different amino acids at particular concentration at various temperatures for different drugs are summarized in Table .
Table 1. Literature reported data of limiting apparent molar volume, V 0ϕ, for different amino acids in various drugs at different temperatures.
Kumar et al. [Citation20] studied the interactions of L-serine and L-threonine with drug metformin hydrochloride (Metformin-HCl) by using the volumetric and acoustic methods as a function of temperature. Later, Chauhan et al. [Citation21] studied the interaction of amino acids L-glutamine and L-histidine in aqueous solutions of same drug i.e. metformin hydrochloride (0.01, 0.07 and 0.13 mol kg−1) in the concentration range (0.02-0.20 mol kg−1) at 293.15, 298.15, 303.15, 308.15 and 313.15 K. They found that the V0ϕ values increase with an increase in temperature for all these amino acids which they explained on the basis that the release of some solvation molecules from the loose solvation layers of the solutes in solution. This explained by considering the size of primary and secondary solvation layers around zwitterions. At higher temperatures, the solvent from the secondary solvation layers of amino acid zwitterions is released into the bulk of the solvent due to weaking of intermolecular interactions which results into the expansion of solution. In simple terms, an increase in temperature reduces the electrostriction and hence V0ϕ increases.
Later, Rajagopal et al. [Citation22] also reported the values for L-proline in the same drug i.e. aqueous metformin hydrochloride solutions for temperature range 298.15-318.15 K. In this case, they found that the values of V0ϕ increase with increase in concentration of drug but decrease with increase in temperature. They suggested that the increase in V0ϕ values with the increase in concentration of solutes may be related to the reduction in the electrostriction at the terminals.
The values of limiting apparent molar volume at different temperatures for all these above amino acids in aqueous metformin hydrochloride are graphically represented in Figure . These values are found to be different for different amino acids at different temperatures. From graph it has been observed that the values of limiting apparent molar volume are highest for L-glutamine and lowest for L-serine. It has also observed that the values of V0ϕ increase with increase in temperature for all these amino acids except L-proline in which values of V0ϕ decrease with increase in temperature.
Figure 1. Plot of Limiting apparent molar volume, V0ϕ, versus temperature, T, for different amino acid in aqueous solution of metformin hydrochloride at different concentrations (a)




![Figure 1. Plot of Limiting apparent molar volume, V0ϕ, versus temperature, T, for different amino acid in aqueous solution of metformin hydrochloride at different concentrations (a) Display full size, L-serine in 0.09 mol kg−1 Metformin hydrochloride [Citation20], (b) Display full size, L-threonine in 0.09 mol kg−1 metformin hydrochloride [Citation20], (c) Display full size, L-histidine in 0.07 mol kg−1 metformin hydrochloride [Citation21], (d) Display full size, L-glutamine in 0.07 mol kg−1 metformin hydrochloride [Citation21], and (c) Display full size, L-proline in 0.10 mol kg−1 metformin hydrochloride [Citation22].](/cms/asset/8810cdb4-b80e-488f-93cc-dd2dff799ff3/tmph_a_1992029_f0001_oc.jpg)
Nain and Lather [Citation23,Citation24] reported limiting apparent molar volume for L-histidine, L-arginine, glycine, L-alanine, L-valine and L-isoleucine in water and in aqueous-streptomycin sulphate (1% and 2% streptomycin sulphate in water) at different temperatures in their two different publications. They observed that the values of limiting apparent molar volume increase with increase in temperature as well as increase in concentration of streptomycin sulfate for all these amino acids. They explained the increase in V0ϕ values with increase in temperature for these amino acids by considering the size of primary and secondary solvation layers around the zwitterions. Later on, Rani et al. [Citation24] also reported the positive values of limiting apparent molar volume for L-serine, L-threonine and L-leucine in same drug solvent (aqueous streptomycin sulfate) at different temperatures and at atmospheric pressure. They suggested that presence of ion-hydrophilic interactions, which dominate over ion-hydrophobic and hydrophobic-hydrophobic interactions. They also revealed that the increase in V0ϕ values with increase in temperature for all amino acids may also be due to release of some solvation molecules from the loose solvation layers of the solute in solution resulting into the expansion of solution. These values of limiting apparent molar volume at different temperatures for all these above amino acids in aqueous streptomycin sulfate are also graphically represented in Figure . From graph it has been observed that the values of limiting apparent molar volume are highest for L-arginine and lowest for glycine due to the increase in hydrophobic character of the side chain of L-arginine. It has also observed that the values of V0ϕ increase with increase in temperature for all these amino acids in aqueous streptomycin sulfate.
Figure 2. Plot of Limiting apparent molar volume, V0ϕ, versus temperature, T, for different amino acid in aqueous solution of Streptomycin sulphate at different concentrations (a)








![Figure 2. Plot of Limiting apparent molar volume, V0ϕ, versus temperature, T, for different amino acid in aqueous solution of Streptomycin sulphate at different concentrations (a) Display full size, Glycine in 1% Streptomycin sulphate [Citation2], (b) Display full size, L-alanine in 01% Streptomycin sulphate [Citation2], (c) Display full size, L-valine in 1% Streptomycin sulphate [Citation2], (d) Display full size, L-isoleucine in 1% Streptomycin sulphate [Citation2], and (e) Display full size, L-histidine in 1% Streptomycin sulphate [Citation23], (f) Display full size, L-arginine in 1% Streptomycin sulphate [Citation23], (g) Display full size, L-serine in 0.02 mol kg−1 Streptomycin sulphate [Citation24], (h) Display full size,L-threonine in 0.02 mol kg−1 Streptomycin sulphate [Citation24], and (i) Display full size, L-leucine in 0.02 mol kg−1 Streptomycin sulphate [Citation24].](/cms/asset/8b42f749-a38a-4fd8-a71b-b9e0cc0ccc1f/tmph_a_1992029_f0002_oc.jpg)
Sharma et al. [Citation25] reported the limiting apparent molar volume V0ϕ of glycine, L-alanine and L-valine in 0.05, 0.15, 0.25 and 0.35 mol kg−1 sodium ibuprofen at temperatures (288.15, 293.15, 298.15, 303.15 and 308.15) K. They found the values to be positive for all these three amino acids which increase with the increase in temperature, indicating the presence of strong solute-solvent interactions. They explained that the increase in V0ϕ with temperature may be attributed to the loosening of water molecules from the solvation layers of amino acids in aqueous Na-IBP medium, releasing some of solvent molecules to the bulk. They noticed that the values increase from L-glycine to L-valine in the order glycine < L-alanine < L-valine which is attributed to the increase in hydrophobic character of the side chain.
Kumar and Kaur [Citation26] calculated limiting apparent molar volume, V0ϕ for L-alanine, L-valine and L-leucine in aqueous solution of ampicillin at different temperatures and atmospheric pressure. Later on, Sahwney et al. [Citation27] used the same drug and calculated limiting apparent molar volume, V0ϕ for L-histidine in different concentrations of ampicillin over a wide range of temperatures (293.15, 298.15, 303.15, 308.15 and 313.15) K and at atmospheric pressure. They found the values to be positive for all the amino acids (L-alanine, L-valine and L-leucine and L-histidine) which indicate the presence of solute-solvent interactions and these interactions increases with increase in the ampicillin concentration and temperature. They explained that at higher temperature might favour the release of some solvent molecules from the secondary solvation layer of L-histidine into the bulk of the solvent, rather than binding with charged end groups, which results expansion in volume of the solution. From these values of V0ϕ, it has been found that these values are highest for L-leucine and lowest for L-alanine} which may be due to increase in chain length of alkyl part of L-leucine.
Arsule et.al. [Citation28] calculated limiting apparent molar volume, V0ϕ for Glycine, L-serine, L-proline, L-asparagine and L-arginine in 0.001, 0.005 and 0.010 mol kg−1 aqueous solutions of acetylsalicylic acid drug at different temperatures which were found to be positive. They suggested that the positive values of V0ϕ signify effective solute-solvent interaction.
Yan et al. [Citation29] reported the interactions of four α-amino acids (Glycine, L-alanine, L-valine and L-leucine) and three glycyl dipeptides namely Glycylglycine, Glycyl L-valine and Glycyl L-leucine with drug domiphen bromide (DB) at different temperatures. They noticed the positive values of partial molar volumes at infinite dilution which indicates the presence of strong solute-solvent interactions (hydrophilic-hydrophilic interactions). These positive values increase with rise in concentration of DB as well as temperature for both amino acids and dipeptides. They observed that values of V0ϕ follow the trend: glycyl-L-leucine > glycyl-L-valine > L-leucine > L-valine > glycylglycine > L-alanine > glycine. The higher values are due to increase in chain length of alkyl part of solute.
Singla et.al [Citation30] reported limiting apparent molar volume, V0ϕ for glycine, L-alanine, L-valine and L-Leucine in aqueous solutions of antibacterial drug amoxicillin (AMX) at different temperatures. They found that the values of limiting apparent molar volume, V0ϕ to be positive which increase with increase in the AMX concentration and temperature. They observed that at each temperature, the values increase with increase in chain length of alkyl part from glycine to L-alanine to L-valine to L-leucine.
Later on Kumar and Kaur [Citation31] also calculated limiting apparent molar volume for L-serine with drugs ampicillin (AMP) and amoxicillin (AMX) at different temperatures. From the results, they observed that the apparent molar volume of L-serine increases with the increase in temperature as well as increase in concentration of AMP and AMX. From these two reported data of amoxicillin [Citation30,Citation31], it has been concluded that the limiting apparent molar volume follow the trend: glycine < L-alanine < L-serine < L-valine < L-leucine.
Pal and Chauhan [Citation32] reported the interactions of amino acids and peptides with the drug pentoxifylline in aqueous solution at various temperatures. They found that the limiting apparent molar volume at infinite dilution increase with increase in temperature for all the amino acids in aqueous drug solution which may be due to increase in solute-solvent interactions. These values follow the order: glycylglycylglycine > L-valine > glycylglycine > Lalanine > glycine.
Wang et.al [Citation33] studied the volumetric and viscometric properties of amino acids (Glyine, L-alanine, L-valine and L Phenalanine) with aqueous solutions of hydroxypropyl-b-cyclodextrin (HP-b-CD) at different temperatures. They found that the values of limiting apparent molar, V0ϕ were positive for amino acids in aqueous HP-b-CD solutions at all temperatures indicating the presence of strong solute-solvent interactions in these systems. They explained the increase in V0ϕ values with increase in temperature for amino acids in aqueous HP-b-CD solutions by considering the size of primary and secondary solvation layers around the zwitterions. These values of V0ϕ increase in the order: Glycine < L-alanine < L-valine < L- phenyalanine due to increase in alkyl chain length from from Glycine to L-phenyalanine.
Kumar et.al [Citation34] calculated limiting apparent molar volume for L-histidine (0.015–0.135) mol kg−1 in aqueous ketorolac tromethamine solutions (0.1–0.7) mol kg−1 at various temperatures which they found to be positive implying the existence of strong solute-solvent interactions in the system. The V0ϕ values also increases with increase in temperature. They explained this increase in V0ϕ values with temperature by considering the size of primary and secondary solvation layers around zwitterions of L-histidine in aqueous ketorolac tromethamine.
Gupta and Nain [Citation35] reported the V0ϕ for glycine, L-alanine, L-valine and L-isoleucine in aqueous semicarbazide hydrochloride (1 wt % and 2 wt % semicarbazide hydrochloride in water) solvent at different temperatures. Later, they studied the interactions of L-arginine and L-histidine in same solvent i.e. semicarbazide hydrochloride [Citation36]. They found that the values of V0ϕ increase with increase in compositions of hydrotropic agents for all these amino acids. The values of V0ϕ also increase with increase in temperature which may be interpreted by considering the size of solvation layers surrounding the zwitterions. They explained that this may be due to the discharge of solvent from secondary solvation layers of amino acid into the bulk of solvent which results into the expansion of the solution. From these two reported data of semicarbazide hydrochloride, it has been concluded that the limiting apparent molar volume follow the trend: glycine < L-alanine < L-serine < L-valine < L-histidine < L-isoleucine < L-arginine.
Sharma et.al [Citation37] investigated the molecular interaction of some amino acids (glycine, L-alanine and L-valine) with the drug levofloxacin in aqueous solution at different temperatures. They found the value of limiting apparent molar volume are positive for amino acid present in drug which shows that there is strong solute-solvent interaction. These values increase from glycine to L-alanine and from L-alanine to L-valine due to increase in hydrophobicity of the chain from glycine to L-valine.
Nain [Citation38] reported the limiting apparent molar volume, V0ϕ for L-arginine/L-histidine in aqueous gentamicin sulphate at different temperatures. He found the V0ϕ values were positive and increase with arising compositions of gentamicin sulphate which signify the presence of strong solute-solvent interactions for L-arginine/L-histidine in aqueous-gentamicin sulphate solutions. He suggested that the gentamicin sulphate molecules interact constructively with water to strengthen H-bond network in the solvent and these values of V0ϕ increase from L-histidine to L-arginine due to increase in chain length of alkyl part of L-arginine as compared to L-histidine. Sawhney et.al [Citation39] investigated the thermodynamic properties of L-alanine and L-valine in aqueous solutions of drug dolonex by combination of volumetric and acoustic methods at various temperatures and found the positive values of limiting apparent molar volume which increases with increase in temperature and also increases with increase in number of alkyl groups from L-alanine to L-valine.
Shirvali et.al [Citation40] reported temperature dependent volumetric and acoustic properties of L-histidine, L-valine and Glycine in aqueous solutions of an antidepression drug amitriptyline at different concentrations and temperature. In volumetric properties, they found the positive limiting apparent molar volume which indicates the presence of strong solute- solvent interaction in aqueous solution of drug and increase with increase in temperature and concentration. These values increase from glycine to L-valine amd from L-valine to L-histidine.
2.2. Limiting apparent molar volume of transfer
Limiting thermodynamic transfer properties provide information about the solute-cosolute interaction, because at infinitesimal concentration the interactions between individual solute molecules are negligible. Hence, limiting apparent molar volume of transfer, ΔtrV0ϕ, is free from solute-solute interactions and provides valuable information about solute-solvent (solute-cosolute) interactions. The transfer volume, ΔtrV0ϕ, of amino acids from water to aqueous drug solutions at infinite dilution can be calculated by using the equation [Citation20]:
(3)
(3) where V0ϕ (in water) is the limiting values of apparent molar volume of amino acids in aqueous medium.
By using Equation (3) many authors calculated limiting apparent molar volume of transfer, ΔtrV0ϕ, for different amino acids in various drugs at different temperatures which are summarized in Table .
Table 2. Literature reported data of limiting apparent molar volume of transfer, ΔtrV0ϕ, for different amino acids in various drugs at different temperatures.
The transfer volume for L-serine and L-threonine in aqueous solution of Metformin-HCl has been calculated by Kumar et al. [Citation20] whereas the transfer volume for L-glutamine and L-histidine in same drug has been calculated by Chauhan et.al [Citation21] and for L-proline by Rajagopal et al. [Citation22]. From these published data, the values of transfer volume found to be positive. They suggested that the ion-hydrophilic interactions between zwitterionic centres of amino acids and polar groups of metformin-HCl and hydrophilic-hydrophilic interactions between polar groups of amino acids and polar groups of metformin-HCl dominate over ion-hydrophobic interactions between zwitterionic centres of amino acids and non-polar groups of metformin-HCl and hydrophobic-hydrophobic interactions between non-polar groups of amino acids and non-polar groups of metformin-HCl. It has been observed from data of these three publications that the values of transfer volume follow the order: L-histidine > L-glutamine > L-proline > L-threonine > L- serine at all experimental conditions which may be due to dominating of ion-ion interactions in case of L-histidine as a consequence of the presence of extra positive charge on its imidazole ring in aqueous solutions.
Nain and Lather [Citation23] reported the positive values of limiting apparent molar volume of transfer for L-histidine and L-arginine in aqueous streptomycin sulphate solution. Thereafter, Nain et al. [Citation2] have used the same solvent i.e. streptomycin sulfate and calculated transfer volume of glycine, L-alanine, L-valine and L-isoleucine. In both the publications, they found that values of transfer volumes, ΔtrV0ϕ to be positive. They suggested that the positive values indicate the dominance of the hydrophilic-ionic interaction between -OH and -NH groups of streptomycin sulphate and zwitterions groups of amino acids over hydrophilic–hydrophobic interaction between the -OH and -NH groups of streptomycin sulphate molecule and non-polar (-CH2) side chain of amino acids and hydrophobic-hydrophobic group interactions between the non-polar groups of streptomycin sulphate and non-polar -CH2 in side chain of amino acids. They also observed that the ΔtrV0ϕ values are larger in case of L-arginine than L-histidine solutions due to more hydrophilic-hydrophilic interactions which is due to more hydrophilic groups present in L-arginine.
Later on, the positive values of transfer volume for L-serine, L-threonine and L-leucine in aqueous streptomycin sulfate solution have been reported by Rani et al. [Citation24]. They also explained that ionic-hydrophilic interactions between zwitterionic centers of amino acids and polar groups of streptomycin sulfate, and hydrophilic-hydrophilic interactions between polar groups of amino acids and polar groups of streptomycin sulfate dominate over ion-hydrophobic and hydrophobic-hydrophobic interactions. They also noticed the lower value of ΔtrV0ϕ for L-leucine as compared to L-threonine and L-serine which may be due to the presence of extra hydrophobic group in l-leucine.
Sharma et al. [Citation25] noticed the positive values of transfer volume for all the amino acids except less negative values for L-valine in 0.15 mol kg−1 Na-IBP at lower temperatures which suggest that hydrophilic-hydrophilic interactions between Na-IBP and amino acids dominate over hydrophilic–hydrophobic and hydrophobic-hydrophobic interactions between amino acids and Na-IBP.
Kumar and Kaur [Citation26] reported the positive values of transfer volume, ΔtrV0ϕ for L-alanine, L-valine and L-leucine in aqueous ampicillin solution. Later on, the positive volume of transfer for L-histidine in same solvent i.e. aqueous ampicillin sodium has also been reported by Sawhney et.al [Citation27]. They explained that the positive values indicate that ionic-hydrophillic interactions between polar groups of ampicillin sodium and zwitter-ions of amino acid and hydrophilic-hydrophillic interactions between polar groups of ampicillin sodium and polar groups of amino acid predominate over ionic-hydrophobic interaction between ionic group of ampicillin sodium/amino acid and non polar group of amino acid/ampicillin sodium and hydrophobic-hydrophobic interaction between non-polar group of ampicillin sodium and non polar group of amino acid.
Arsule et.al [Citation28] found the positive volume of transfer for glycine, L-serine, L-proline, L-asparagine monohydrate except L-arginine in acetylsalicylic acid drug which suggested ion-hydrophilic and hydrophilic-hydrophilic interactions dominates over the ion-hydrophobic and hydrophobic-hydrophobic interactions between amino acids and acetylsalicylic acid which imply the transfer of H+ to L-arginine from acetylsalicylic acid drug.
Yan et al. [Citation29] calculated the values of transfer volume for alpha-amino acids (Glycine, L-alanine, L-valine and L-leucine) and three glycyl dipeptides (Glycylglycine, Glycyl L-valine and Glycyl L-leucine) in domiphen bromide and found to be positive values for all these solutes which indicates that the hydrophilic-hydrophilic interaction between ions of the DB and charged head groups (-NH3+, COO-) of amino acids/dipeptides as well as the peptide bond (-CONH) group of dipeptides predominate over hydrophilic–hydrophobic interaction between zwitterionic centers of amino acids/dipeptides and non-polar groups of DB, as well as between the ions of DB and the hydrophobic side chain of amino acids/dipeptides and hydrophobic-hydrophobic interaction between the alkyl chain of the DB and the hydrophobic group of the amino acids/dipeptides.
Singla et al. [Citation30] observed the positive value of transfer volume for glycine, L-alanine, L-valine and L-leucine in aqueous solutions of amoxicillin and they suggested that ion-hydrophilic interactions between zwitterionic centers of amino acids and polar groups of amoxicillin and hydrophilic-hydrophilic interactions between polar groups of amino acids and polar groups of amoxicillin dominated over ion-hydrophobic interactions between zwitterionic centers of amino acids and non-polar groups of amoxicillin and hydrophobic-hydrophobic interactions between non-polar groups of amino acids and non-polar groups of amoxicillin in (amino acid + water + amoxicillin) mixtures.
The transfer volume of amino acids and peptides with the drug pentoxifylline was calculated by Pal and Chauhan [Citation32] which they found to be positive indicated the dominance of ion-hydrophilic and ion-hydrophobic interactions.
Wang et al. [Citation33] calculated the values of transfer volume for glycine and phenylaninine in aqueous hydroxypropyl-b-cyclodextrin and found that these values are negative. They explained that this suggest that the ion-hydrophobic and hydrophobic-hydrophobic group interactions predominate over the ion-hydrophilic and hydrophilic-hydrophilic groups interactions in the ternary solutions (amino acid + HP-β-CD + water).
The positive values of transfer volume for L-histidine in aqueous ketorolac tromethamine have been reported by Kumar et al. [Citation34] which increases with increase in concentration of ketorolac tromethamine. They explained that the positive volume of transfer indicates the ionic-hydrophillic interactions between polar groups of ketorolac tromethamine and zwitter-ions of L-histidine and hydrophilic-hydrophillic interactions between polar groups of ketorolac tromethamine and polar group of L-histidine predominate over ionic-hydrophobic interaction between ionic group of ketorolac tromethamine/L-histidine and non polar group of L-histidine / ketorolac tromethamine and hydrophobic-hydrophobic interaction between non polar group of ketorolac tromethamine and non polar group of L-Histidine.
Gupta and Nain found positive values of transfer volumes for glycine, L-alanine, L-valine and L-isoleucine in aqueous semicarbazide hydrochloride in one of their publication [Citation35] and for L-arginine and L-histidine in aqueous semicarbazide hydrochloride in another publication [Citation36]. From the results of both of their work, they suggested the predominance of the hydrophilic-ionic/hydrophilic interaction between -OH groups of semicarbazide hydrochloride and zwitterions of amino acids over hydrophilic–hydrophobic interaction between the -OH groups of semicarbazide hydrochloride molecule and non-polar (–CH2) in side chain of amino acids and hydrophobic-hydrophobic group interactions between the non-polar groups of semicarbazide hydrochloride and non-polar (–CH2) in side chain of amino acids.
The positive values of the limiting molar volume of transfer for glycine, L-alanine and L-valine in aqueous levofloxacin have been reported by Sharma et al. [Citation37]. They suggested ion-ion interactions between the zwitterionic end groups (NH+/ COO−) of amino acids and cationic (NH+) /anionic (COO−) side of levofloxacin predominates over ion-hydrophobic interactions between ionic groups (NH+/ COO−) of amino acids and hydrophobic side chains -CH3 of levofloxacin and between (NH+/COO−) and hydrophobic side chains of amino acids and hydrophobic-hydrophobic interactions.
Nain [Citation38] observed the values of volume of transfer to be positive for both L-arginine and L-histidine in gentamicin sulphate indicating the predominance of the ‘hydrophilic-ionic interaction between polar groups of gentamicin sulphate and COO− group of amino acids’ and the ‘hydrophilic-ionic interaction between polar groups of gentamicin sulphate and NH+ group of amino acids’ over ‘hydrophilic–hydrophobic interaction between polar groups of gentamicin sulphate molecule and non-polar (-CH2) side chain of amino acids’, and ‘hydrophobic-hydrophobic group interactions between the non-polar groups of gentamicin sulphate and non-polar (-CH2) side chain of amino acids’.
Sawhney et.al [Citation39] noticed the positive value of transfer volume and explained that ion-ion interactions between polar group of dolonex and zwitter-ionic groups of amino acid and hydrophilic-hydrophilic interactions between polar groups of dolonex and polar group of amino acid prevails over ionic-hydrophobic interaction between ionic group of dolonex/amino acid and non polar groups of amino acid/dolonex and non-polar-non-polar group interaction between hydrophobic group of dolonex and non polar group of amino acid.
2.3. Temperature dependent limiting apparent molar volume
The variation of limiting apparent molar volume at infinite dilution with the temperature can be expressed by the following general polynomial Equation [Citation20]:
(4)
(4) where T is temperature in Kelvin; a, b and c are empirical constants. The limiting apparent molar expansivity can be evaluated as follows [Citation20]:
(5)
(5) These values of limiting apparent molar expansivity, E0ϕ for different amino acids in various drugs at particular concentrations of drugs are reported in Table . These values of E0ϕ are considered to be a useful measure of solute-cosolute interactions in the solution. From Table , it has been found that that values for all the amino acids in various drugs are positive and increase with increase in temperature. They explained that the increase in values of E0ϕ, with temperature may occur due to phenomenon of packing or caging effect. The temperature affects outer hydrated water molecules which may give rise to higher values of E0ϕ with increase in temperature.
Table 3. Literature reported data of limiting apparent exapansivity, E 0ϕ, for different amino acids in various drugs at different temperatures.
Since E0ϕ is only a qualitative parameter to measure the solute-cosolute interactions,
Hepler [Citation41] developed a general thermodynamic expression to show whether a particular solute will behave as structure maker or structure breaker in mixed solvent system.
(6)
(6) The values of Hepler’s constant, (∂E0ϕ /∂T)P, for different amino acids in various drugs at different temperatures have been reported by many authors by using Equation (6) in the literature which are also summarised in Table .
Table 4. Literature reported data of Hepler’s constant, (∂E0ϕ /∂T)P, for different amino acids in various drugs.
Kumar et al. [Citation20] calculated Hepler’s constant and found positive and small negative values for mixtures of amino acids (L-serine and L-threonine) which indicated the structure making ability of amino acids in all aqueous metformin-HCl solutions. Thereafter, Chauhan et al. [Citation21] reported the positive values of Hepler’s constant for both L-histidine and L-arginine in same solvent i.e. aqueous metformin hydrochloride. Later, Hepler’s constant calculated by Rajagopal et al.[Citation22] for L-proline in same metformin hydrochloride was found to be positive for all concentrations (except for 0.20 mol.kg−1) of metformin hydrochloride. They suggested the structure-making ability of L-proline in aqueous metformin hydrochloride solutions.
Rani et al. [Citation24] calculated the Hepler’s constant whose values for L-serine and L-threonine were found to be positive which indicated that these act as structure maker while for leucine, the values were found to be negative which indicated that it acts as structure breaker in aqueous streptomycin sulfate solutions.
Sharma et al. [Citation25] calculated Hepler’s constant for all the amino acid which were found to be negative (and very small positive for L-valine in lower concentration region of Na-IBP) indicate that these act as structure breaker in aqueous Na-IBP solutions.
Kumar and Kaur calculated [Citation26] Hepler’s constant for L-alanine, L-valine and L-leucine in aqueous solution of ampicillin at different temperatures and atmospheric pressure. They found the values were positive for L-alanine while negative for L-valine and L-leucine at all concentrations except for L-valine at concentration of 0.004 mol kg−1 ampicillin whereas it shows positive value. These results indicated that L-alanine acts as structure maker whereas L-valine and L-leucine acts as structure breaker in aqueous ampicillin sodium solutions. Later, Sahwney et al. [Citation27] used the same drug and calculated Hepler's constant for L-histidine and found that the values were positive for L-histidine which indicated that L-histidine acts as structure maker in presence of aqueous ampicillin.
Yan et al. [Citation29] calculated Hepler’s constant for Glycine, L-alanine, L-valine and L-leucine, glycyl dipeptides Glycylglycine, Glycyl L-valine and Glycyl L-leucineGlycine, in drug domiphen bromide and found their positive values which suggested that these behave as structure maker in aqueous domiphen bromide solution.
Singla et al. [Citation30] calculated Hepler’s constant for Glycine, L-alanine, L-valine and L-leucine in amoxyillin and found the values to be positive for these amino acids which indicated the structure making ability of all these amino acids in aqueous amoxyillin solutions.
Pal and Chauhan [Citation32] calculated Hepler’s constant for amino acids and found the negative value which revealed that amino acids acts as structure breaker in presence of Pentoxifylline drug.
Wang et al. [Citation33] calculated Hepler’s constant for glycine and phenylaninine in aqueous hydroxypropyl-b-cyclodextrin and found the transfer volume to be positive indicated that these amino acid as structure maker in all experimental solutions.
Kumar et al. [Citation34] noticed positive values of Hepler's constant suggested that L-histidine shows structure maker behaviour in aqueous ketorolac tromethamine solutions.
Sharma et.al [Citation37] calculated the Hepler's constant for Glycine, L-alanine and L-valine and observed the negative and small positive values for these amino acids which conclude that amino acids act as structure-breaker (chaotropic effect) in aqueous levofloxacin solution.
Sawhney et.al [Citation39] observed negative values of Hepler's constant for both L-alanine and L-valine which shows chaotropic behaviour (structure-breaker) of L-alanine /L-valine in aqueous dolonex solutions.
2.4. Hydration number
In case of amino acids, hydration number is calculated by using following relations: The partial molar volume of the amino acids can be studied by a simple model given by following equation:
(7)
(7) where V0ϕ (elect.) is the electrostriction partial molar volume due to the hydration of amino acid and can be calculated from experimentally measured values of V0ϕ (amino acid), and V0ϕ (int.) is the intrinsic partial molar volume of the amino acid and has been calculated from the following equation:
(8)
(8) where V0ϕ (cryst.) is the crystal molar volume, dcryst. is the densities of L-amino acids in the dry state (anhydrous), 0.7 is the packing density for molecules in organic crystals and 0.634 is the packing density for random packing spheres. The value of V0ϕ (int.) for amino acid can be estimated from above equation using dcryst values for the amino acids taken from the work of Berlin and Pallansch [Citation42].
V0ϕ (elect.) can be calculated from experimentally measured values of V0ϕ (amino acid):
(9)
(9) The change in volume due to elecrostriction can be related to the number of water molecules, nH, hydrated to the amino acid by following equation:
(10)
(10) where V0ϕ,e is the molar volume of electrostricted water, V0ϕ,b is the molar volume of bulk water and (V0ϕ,e - V0ϕ,b) = −3.1, −3.3, −3.7, −4.0 and −4.2 cm3 mol−1 [Citation43,Citation44] at T = (293.15, 298.15, 303.15, 308.15 and 313.15) K, respectively.
By using Equation (10) the values of hydration for different amino acids in various drugs have been reported by many authors in the literature. Some of these values are given in Table .
Table 5. Literature reported data of Hydration number, nH for different amino acids in various drugs at different temperatures.
Nain et al. [Citation2] calculated the hydration number, nH, for glycine, L-alanine, L-valine and L-isoleucine in aqueous-streptomycin sulfate solution at T = 298.15 K and they found that hydration number decrease with increase in concentration of streptomycin sulfate which they suggested that the number of water molecules hydrated to amino acids decrease with increase in concentration of streptomycin sulfate.
Sharma et al. [Citation25] calculated the hydration number for glycine, L-alanine and L-valine in aqueous solutions of sodium ibuprofen and observed that the values for the studied amino acids are less in the presence of Na-IBP as compared to their values in water and their magnitude is lowest in higher concentration region of Na-IBP. This indicates the increase in solute-co-solute interactions.
Rajagopal et al. [Citation22] calculated hydration number at different temperatures and observed that the hydration number of L-proline in aqueous metformin hydrochloride decreases with increasing concentration of metformin hydrochloride and increases with temperature, which substantiates the predominance of solute-co-solute interactions. This establishes the fact that metformin hydrochloride has a dehydration effect on the amino acid.
Sahwney et al. [Citation27] observed that the hydration number, nH decreases with increase in concentration of ampicillin sodium and also decreases with increase in temperature. The nH values for L-histidine in presence of ampicillin sodium are less than that in water, this suggests that with the increase in concentration of ampicillin sodium there occurs a strong interaction between ions of ampicillin sodium and charged end groups of L-histidine.
Yan et al. [Citation29] found that the hydration number values for amino acids/dipeptides in aqueous DB solutions are larger in 0.04 mol kg−1 DB solutions than those in 0.08 mol kg−1 DB solutions, which indicates the increase in solute-cosolute (amino acid/ dipeptide-DB) interaction with the increase in concentration of DB in the solution.
Wang et al. [Citation33] calculated the hydration number for the amino acids in aqueous HP-b-CD solutions and observed that they vary in the order: L-phenyalanine > L-valine > L-alanine > L-glycine. They explained that though the hydrophobicity of amino acids also increases as the alkyl chain lengthens, the electrostriction around the zwitter ionic end groups increases leading to a higher value of the hydration number.
The values of hydration number for L-histidine in aqueous ketorolac tromethamine have been reported by Kumar et al. [Citation34] and these values decrease with increase in concentration of ketorolac tromethamine which indicated that the number of water molecules hydrated to amino acids decrease with increase in concentration of ketorolac tromethamine. In other words, ketorolac tromethamine has a dehydration effect on these amino acids.
Gupta and Nain [Citation35] calculated hydration number at T = 298.15 K and found that the hydration number decreases with increasing concentration of semicarbazide hydrochloride. Further this establishes the fact that the drug has a dehydrating effect on the hydration sphere of glycine, L-alanine, L-valine and L-isoleucine in solutions. The higher drug concentration makes more drug molecules to replace water molecules surrounding amino acid molecules, leading to weakening of hydration and enhancement of solvation. This indicates the increase in solute-cosolute interactions.
Sharma et al. [Citation37] found that the hydration number of amino acids at different temperatures decreases with increasing concentration of levofloxacin with some exception, which again substantiate the increase in solute-co-solute interactions. Further this establishes the fact that levofloxacin has a dehydration effect on the amino acids.
3.0. Viscometric properties
The study of viscous behavior of biomolecules in solutions is important in understanding the mechanism of transport processes. Viscosity and its derived parameters provide valuable information regarding the shape and size of the molecules [Citation45]. The variation of relative viscosity, ηr, for solute in aqueous solvent can be represented by Jones-Dole equation [Citation46].
(11)
(11) where η and η0 are the viscosities of the solution and solvent, respectively, while A and B are the Falkenhagen [Citation47,Citation48] and Jones-Dole [Citation46] or viscosity B- coefficients, respectively. The first parameter represents interactions between solute-solute [Citation49] while the later is a measure of structural modification induced by solute-solvent interaction [Citation50–52]. Equation. (11) is valid for electrolyte. But in case of non-electrolytes, A is negligible [Citation53], therefore, Jones-Dole equation can be reduced to:
(12)
(12) where m is the molaity and C is the concentration in moles per unit volume (molarity). The conversion of molality ‘m’ to molarity ‘C’ is done by using our density values.
3.1. Viscosity B-coefficients
The least-squares fitting of relative viscosity to Equation (12) gives the values of viscosity B-coefficients. The above viscometric properties of amino acids in aqueous solution of various drugs have also been investigated and reported by many researchers in the literature. Therefore, by using Equations (11) and (12), the values of viscosity B-coefficients for amino acids in various drugs have been reported by many authors in the literature which are given in Table .
Table 6. Literature reported data of Viscosity B-cofficient, B, for different amino acids in various drugs at different temperatures.
From viscometric data, Nain et al. [Citation2] determined the viscosity B-coefficients values for glycine, L-alanine, L-valine and L-leucine in 1% and 2% aqueous-streptomycin sulfate and found that these values are larger in 2% aqueous-streptomycin sulfate than in the 1% aqueous-streptomycin sulfate solvents due to greater hydrophilic–ionic group interactions.
Chauhan et al. [Citation21] determined the viscosity B-coefficient for L-glutamine and L-histidine in metformine hydrochloride and found that the values were positive for both the amino acids which are indicative of kosmotropes, since amino acids are the strongly hydrated solutes and, therefore, exhibit a larger change in the viscosity of the solution with concentration. Thus, the viscosity B-coefficient in all cases suggested the presence of strong solute-solvent interactions. They explained that the solute-solvent interactions are more effective in the presence of L-histidine as reflected by its higher viscosity B-coefficient compared to those in L-glutamine.
Nain and Lather [Citation23] observed the positive values of viscosity B-coefficient for L-histidine and L-arginine in aqueous streptomycin sulphate which increase with increasing concentration of streptomycin sulphate and suggested that the friction increases to prevent water flow at increased streptomycin sulphate concentration. Later, the values of viscosity B-coefficient have been calculated by Rani et al. [Citation24] and they observed that the values of viscosity B-coefficients are positive for L-serine, L-threonine and L-leucine and increase with increase in molality of streptomycin sulfate, implying the existence of strong solute-solvent interactions in presence of streptomycin sulfate. They also found that the values of B-coefficients follows the order L-leucine > L-threonine > L-serine.
Sawhney et.al [Citation27] observed the positive values of viscosity B-coefficient for L-histidine which increases with increase in concentration of ampicillin sodium and decreases with increase in temperature. They explained the existence of strong solute-solvent interaction with increase in concentration of ampicillin sodium.
The values of the viscosity B-coefficients for glycine, L-alanine, L-valine and L-phenylalanine with aqueous solutions of hydroxypropyl-b-cyclodextrin reported by Wang et.al [Citation33] at different temperatures are found to be positive which increase with rise in temperature indicating increased solute-solvent interactions at higher temperatures in these systems.
Kumar et al. [Citation34] observed that the values of viscosity B-coefficient for L-histidine increases with increase in concentration of ketorolac tromethamine which indicated the existence of strong solute-solvent interactions in these solutions. They suggested that the increase in viscosity B-coefficient with increase in concentration of co-solute (ketorolac tromethamine) may be due to the increase in friction that prevents water flow at increased ketorolac tromethamine concentration.
Gupta and Nain [Citation35] noticed positive viscosity B-coefficients values for glycine, L-alanine, L-valine and L-isoleucine in aqueous semicarbazide hydrochloride which indicated the presence of strong solute-solvent interactions in these solutions. After that the positive viscosity B-coefficients values of L-arginine and L-histidine have been reported by Gupta and Nain [Citation36] for same drug. i.e. semicarbazide hydrochloride. They explained that the large and positive viscosity B-coefficients values, which increase with increasing concentration of semicarbazide hydrochloride at all experimental temperatures, suggest the structure making effect of the drug, i.e. contraction of solvent molecules around solute (amino acid molecules). They found that the values of viscosity B-coefficient in 1 wt % aqueous-semicarbazide hydrochloride solution are smaller than those in 2 wt% aqueous semicarbazide hydrochloride solution. They suggested that in higher composition of hydrotropic agents, the increase in viscosity B-coefficient might be due to formation of clathrates by liquid aggregates of water + semicarbazide hydrochloride solvent around amino acid molecules. This brings about a strong association of the molecules and growth of vander Waals forces into significant factors which lead to the formation of clathrate.
3.2. Temperature derivative of viscosity B-cofficient
Temperature derivative of viscosity B-cofficient i.e. dB/dT provides important information regarding the structure-making and structure breaking ability of the solute in the solvent media [Citation49] rather than simply the viscosity B-coefficients. The positive dB/dT values indicate structure breaker characteristics while negative dB/dT values indicate structure maker characteristics. These values of dB/dT for amino acids in various drugs were also reported in the literature.
Chauhan et al. [Citation21] observed the negative values of dB/dT which suggested the structure promoting tendency of L-histidine and L-glutamine in aqueous metformin-HCl.
Nain et al. [Citation2] found the positive dB/dT values for glycine, L-alanine, L-valine and L-isoleucine in aqueous-streptomycin sulfate solvents which indicate that these amino acids act as structure breaker in aqueous-streptomycin sulfate solvents under study. After that, Nain and Lather [Citation23] observed the negative dB/dT values for the amino acids (L-histidine and L-arginine) in same solvent i.e. aqueous-streptomycin sulphate solutions and they suggested that both the amino acids (L-histidine and L-arginine) act as structure makers in aqueous-streptomycin sulphate solvents.
Later on, the values of dB/dT were also reported by Rani et al. [Citation24] for three amino acids (L-serine, L-threonine and L-leucine) in the same solvent i.e. aqueous streptomycin sulphate and they found the values were negative for all these amino acids which indicated the structure-making behavior of these amino acid in aqueous solutions of streptomycin sulfate.
Sawhney et al. [Citation27] determined the dB/dT values and observed the values to be negative for L-histidine in water as well as in aqueous ampicillin sodium solution suggesting the structure making behaviour of L-histidine.
Kumar et al. [Citation34] determined the values of dB⁄dT and found that these values are negative which indicates that L-histidine shows structure making behaviour in water as well as in aqueous solutions of ketorolac tromethamine.
Gupta and Nain [Citation35] calculated dB/dT values for glycine, L-alanine, L-valine and L-isoleucine in semicarbazide hydrochloride and observed the negative values which specify that all these amino acids act as structure-maker in these aqueous semicarbazide hydrochloride.
Thereafter, Gupta and Nain [Citation36] determined the positive dB/dT values for L-arginine/L-histidine by the use of same solvent i.e. semicarbazide hydrochloride which specify that these amino acids act as structure-breaker in the aqueous-semicarbazide hydrochloride solvents.
Disclosure statement
No potential conflict of interest was reported by the author(s).
REFERENCES
- M.J. Iqbal, S. Mahrukh and J. Braz, Chem. Soc. 17, 851–858 (2006).
- A.K. Nain, R. Pal and P. Droliya, J. Chem. Thermodyn. 95, 77–98 (2016). doi:https://doi.org/10.1016/j.jct.2015.11.015
- A.N. Manin, L.E. Shmukler, L.P. Safonova and G.L. Perlovich, J. Chem. Thermodyn. 42, 429–435 (2010). doi:https://doi.org/10.1016/j.jct.2009.10.002
- Z. Yan, J. Wang, W. Kong and J. Lu, Fluid Phase Equilib. 215, 143–150 (2004). doi:https://doi.org/10.1016/j.fluid.2003.07.001
- P.L. Privalov, Adv. Proteins Chem. 33, 167–241 (1979). doi:https://doi.org/10.1016/S0065-3233(08)60460-X
- S. Lapanje, Physicochemical Aspects of Protein Denaturation (Wiley, New York, 1978).
- H. Zhao, Biophys. Chem. 122, 157–183 (2006). doi:https://doi.org/10.1016/j.bpc.2006.03.008
- F. Franks, in Biochemical Thermodynamics, edited by M.N. Jones (Elsevier, Amsterdam, 1979). pp. 164–184.
- L.V. Guschina and A.G. Gabdulkhakov, Biophys. Chem. 139, 106–115 (2009). doi:https://doi.org/10.1016/j.bpc.2008.10.011
- M.S. Santosh, D.K. Bhat and A.S. Bhat, J. Chem. Eng. Data. 54, 2813–2818 (2009). doi:https://doi.org/10.1021/je800732f
- P. Ramasami, J. Chem. Eng. Data. 47, 1164–1166 (2002). doi:https://doi.org/10.1021/je025503u
- R. Bhat and J.C. Ahluwalia, J. Phys. Chem. 89, 1099–1105 (1985). doi:https://doi.org/10.1021/j100253a011
- R. Bhat and J.C. Ahluwalia, Int. J. Peptide Protein Res. 30, 145–152 (1987). doi:https://doi.org/10.1111/j.1399-3011.1987.tb03323.x
- G.R. Hedwig, J. Chem. Soc. Faraday Trans. 89, 2761–2768 (1993). doi:https://doi.org/10.1039/ft9938902761.
- G.R. Hedwig and H. Hoiland, J. Chem. Thermodyn. 23, 1029–1035 (1991). doi:https://doi.org/10.1016/S0021-9614(05)80108-9
- J.F. Reading and G.R. Hedwig, J. Chem. Soc. Faraday Trans. 86, 3117–3123 (1990). doi:https://doi.org/10.1039/FT9908603117
- Z. Yan, X. Wang, R. Xing and J. Wang, J. Chem. Eng. Data. 54, 2813–2818 (2009). doi:https://doi.org/10.1021/je800732f
- J.L. Liu, A.W. Hakin and G.R. Hedwig, J. Chem. Thermodyn. 38, 1640–1650 (2006). doi:https://doi.org/10.1016/j.jct.2006.03.015
- R.T. Altamash, J. Chem. Eng. Data. 54, 3133–3139 (2009). doi:https://doi.org/10.1021/je900199j
- H. Kumar, I. Behal and M. Singla, J. Chem. Thermodyn. 95, 1–14 (2016). doi:https://doi.org/10.1016/j.jct.2015.11.020
- S. Chauhan, K. Singh, K. Kumar, S.C. Neelakantan and G. Kumar, J. Chem. Eng. Data. 61, 788–796 (2016).
- K. Rajagopal, M.M. Roshan, S. Shailajha and G.R.R. Renold, J. Chem. Thermodyn. 133, 312–319 (2019). doi:https://doi.org/10.1016/j.jct.2019.02.012
- A.K. Nain and M. Lather, J. Mol. Liq. 211, 178–186 (2015). doi:https://doi.org/10.1016/j.molliq.2015.07.018
- R. Rani, A. Kumar and R.K. Bamezai, J Mol. Liq. 224, 1142–1153 (2016). doi:https://doi.org/10.1016/j.molliq.2016.10.063
- S.K. Sharma, G. Singh, H. Kumar and R. Kataria, J. Chem. Thermodyn. 98, 214–230 (2016). doi:https://doi.org/10.1016/j.jct.2016.03.016
- H. Kumar and K. Kaur, J. Mol. Liq. 173, 130–136 (2012). doi:https://doi.org/10.1016/j.molliq.2012.07.008
- N. Sawhney, M. Kumar, A.K. Sharma and M. Sharma, J. Chem. Thermodyn. 115, 156–170 (2017). doi:https://doi.org/10.1016/j.jct.2017.07.040
- A.D. Arsule, R.T. Sawale and S.D. Deosarkar, J.Mol. Liq. 275, 478–490 (2019). doi:https://doi.org/10.1016/j.molliq.2018.10.122
- Z. Yan, X. Wen, Y. Kang and W. Chu, J. Chem. Thermodyn. 101, 300–307 (2016). doi:https://doi.org/10.1016/j.jct.2016.06.018
- M. Singla, H. Kumar and R. Jindal, J. Chem. Thermodynamics. 76, 100–115 (2014). doi:https://doi.org/10.1016/j.jct.2014.03.015
- H. Kumar and K. Kaur, J. Chem. Eng. Data. 58, 203–208 (2013). doi:https://doi.org/10.1021/je3006425
- A. Pal and N. Chauhan, J. Chem. Thermodyn. 54, 288–292 (2012). doi:https://doi.org/10.1016/j.jct.2012.05.009
- X. Wang, G. Li, Y. Guo, Q. Zheng, W. Fang, P. Bian and L. Zhang, J. Chem. Thermodyn. 78, 128–133 (2014). doi:https://doi.org/10.1016/j.jct.2014.06.016
- M. Kumar, N. Sawhney, A.K. Sharma and M. Sharma, J. Mol. Liq. 243, 41–51 (2017). doi:https://doi.org/10.1016/j.molliq.2017.08.001
- J. Gupta and A.K. Nain, J. Chem. Thermodyn. 135, 9–26 (2019). doi:https://doi.org/10.1016/j.jct.2019.03.011
- J. Gupta and A.K. Nain, J. Chem. Thermodyn. 144, 1–14 (2020). doi:https://doi.org/10.1016/j.jct.2020.106067
- S.K. Sharma, G. Singh, R. Kataria, H. Kumar and S. Sharma, J. Chem. Thermodyn. 105, 94–104 (2017). doi:https://doi.org/10.1016/j.jct.2016.09.027
- A.K. Nain, J. Mol. Liq. 315, 1–9 (2020). doi:https://doi.org/10.1016/j.molliq.2020.113736
- N. Sawhney, M. Kumar, A.K. Sharma and M. Sharma, J. Chem. Thermodyn. 123, 22–31 (2018). doi:https://doi.org/10.1016/j.jct.2018.03.022
- S. Shirvali, H. Iloukhani and K. Khanlarzadeh, J. Mol. Liq. 295, 1–11 (2019). doi:https://doi.org/10.1016/j.molliq.2019.111651
- G. Hepler, Can. J. Chem. 47, 4613–4617 (1969). doi:https://doi.org/10.1139/v69-762
- E. Berlin and M.J. Pallansch, J. Phys. Chem. 72, 1887–1889 (1968). doi:https://doi.org/10.1021/j100852a004
- Z. Yan, X. Sun, W.W. Li, Y. Li and J. Wang, J. Chem. Thermodyn. 43, 1468–1474 (2011). doi:https://doi.org/10.1016/j.jct.2011.04.020
- F.J. Millero, A.L. Surdo and C. Shin, J. Phys. Chem. 82, 784–792 (1978). doi:https://doi.org/10.1021/j100496a007
- R.H. Stokes and R. Mills, Viscosity of Electrolytes and Related Property (Pergamon, London, 1965).
- G. Jones and M. Dole, J. Am. Chem. Soc. 51, 2950–2964 (1929). doi:https://doi.org/10.1021/ja01385a012
- H. Falkenhagen and M. Dole, Z. Phys. 30, 611–616 (1929).
- H. Falkenhagen and E.L. Vernon, Z. Phys. 33, 140–145 (1932).
- D. Feakins, D.J. Freemantle and K.G. Lawrence, J. Chem. Soc. Faraday Trans. 70, 795–806 (1974). doi:https://doi.org/10.1039/f19747000795
- A. Ali, R. Patel, S. Khan, V. Bhushan and Z. Naturforsch, A 64, 758–764 (2009).
- A.K. Nain and D. Chand, J. Chem. Thermodyn. 41, 243–249 (2009). doi:https://doi.org/10.1016/j.jct.2008.09.008
- S.C. Bai and G.B. Yan, Carbohydr. Res. 338, 2921–2927 (2003). doi:https://doi.org/10.1016/j.carres.2003.08.006
- R.L. Kay, T. Vituccio, C. Zawoyski and D.F. Evans, J. Phys. Chem. 70, 2336–2341 (1966). doi:https://doi.org/10.1021/j100879a041