ABSTRACT
Pecan scab, caused by Venturia effusa, is the most prevalent disease of pecan in the southeastern United States. Recent characterization of the mating type (MAT) distribution of V. effusa revealed that the MAT idiomorphs are in equilibrium at various spatial scales, indicative of regular sexual recombination. However, the occurrence of the sexual stage of V. effusa has never been observed, and the pathogen was previously considered to rely entirely on asexual reproduction. We were able to generate the sexual stage by pairing isolates of opposite mating types on oatmeal culture media. Cultures were incubated at 24 C for 2 mo to allow hyphae from isolates of each mating type to interact. Culture plates were then incubated at 4 C for 4 mo, after which immature pseudothecia were observed. Following exposure to a 12-h photoperiod for 2 wk at 24 C, asci and ascospores readily developed. Pseudothecium and ascospore production was optimal when incubated for 4 mo at 4 C. We utilized progeny from a cross of an albino isolate and wild-type (melanized) isolates to determine that recombination had occurred. Multilocus genotyping using 32 microsatellite markers confirmed that progeny were the result of recombination, which was further supported by segregation of mating types and culture pigmentation. Albino progeny were all confirmed to contain the same mutation in the polyketide synthase (PKS1) melanin biosynthesis gene as the albino parent. The results of this study demonstrate the heterothallic nature of V. effusa. The impact of determining the source of the overwintering ascostroma will aid in management decisions to reduce the primary inoculum in the disease cycle.
INTRODUCTION
Pecan scab, caused by Venturia effusa (G. Winter) Rossman & W.C. Allen (Dothideomycetes, Venturiaceae, previously Fusicladium effusum G. Winter) (Rossman et al. Citation2016), is the most important biotic, yield-limiting issue impacting pecan production in the southeastern United States. Pecan (Carya illinoinensis) is one of the few trees native to North America that have developed into a significant and lucrative specialty crop (Wood et al. Citation1990). Pecan trees can live and bear edible fruit for over a hundred years, if well managed (Wells Citation2017). The value of the pecan crop in the United States in 2017 was estimated at $709,218,000 (based on reports found at National Agricultural Statistics Service [NASS] 2017, www.nass.usda.gov). The estimated impact of scab can be measured in two ways—firstly, the direct impact of the disease reducing yield, and secondly, the cost of fungicides to manage it. For example, in an average scab year such as 2014, statewide production in Georgia was valued at $313,313,250, the yield loss to the disease was estimated at $3.1 million, and the cost of fungicides for control was estimated at $22.6 million (Brock and Brenneman Citation2014). In severe epidemic years, both costs are elevated (Bock et al. Citation2017a).
Venturia effusa was first described in 1885 on mockernut (Carya tomentosa) in Illinois (Winter Citation1885). The pathogen has been considered to perpetuate purely through asexual reproduction by repeated production of conidia throughout its life cycle. The conidia are spread by wind and splash dispersal and can infect under humid, moist conditions; they are a key component of the polycyclic nature of this disease (Gottwald and Bertrand Citation1983; Gottwald Citation1985). The asexual lifecycle can be completed in as little as 7–10 d, and epidemics develop as inoculum builds up throughout the season under favorable environmental conditions during sporulation, dispersal, germination, infection, and lesion development (Gottwald Citation1982; Gottwald and Bertrand Citation1982). When severe, the epidemics may affect the number and quality of harvested nuts (Gottwald and Bertrand Citation1983; Stevenson and Bertrand Citation2001).
The population genetic structure and diversity of a pathogen provides valuable information and insight into the epidemiology of a plant disease. Asexual and sexual reproduction influence the population structure, and ultimately the pathogen’s ability to adapt to different selection pressures (McDonald and Linde Citation2002; McDonald and Mundt Citation2016). Understanding the spatial distribution and occurrence of clones provides insight into the modes of reproduction of a pathogen (McDonald and McDermott Citation1993; Milgroom Citation1996; Bock et al. Citation2016a). Sexual reproduction introduces recombination and diversity into a population. Pathogens with mixed reproduction systems (asexual and sexual) are proposed to have the greatest likelihood of evolution, allowing the introduction of new genotypes through recombination and subsequent distribution of these genotypes through clonal propagation (McDonald and Linde Citation2002). Pathogens exhibiting these characteristics are considered high risk and capable of evading major resistance genes or evolving rapidly to overcome control methods such as specific fungicide types.
Recent sequencing efforts (Bock et al. Citation2016b) have been used to facilitate insight into the population genetic structure and dynamics of V. effusa in the southeastern United States. Evaluation of hierarchically collected isolates from multiple orchards using microsatellite markers has shown substantial and evenly distributed genetic diversity among orchard populations (Bock et al. Citation2017b). Such distribution and nature of the genetic diversity are characteristic of a sexually reproducing species (McDonald et al. Citation1995; Linde et al. Citation2002; Gladieux et al. Citation2008, Citation2010). In addition, V. effusa is proposed to have a heterothallic mating system, as isolates were observed to contain either the MAT1-1-1 or MAT1-2-1 mating type idiomorphs, which also were found to be in equilibrium within the orchards examined (Bock et al. Citation2018; Young et al. Citation2018). Based on these observations, we propose that V. effusa must have a functional sexual stage that is yet to be observed in the field. Closely related species of Venturia (Schubert et al. Citation2003), including V. inaequalis on apple, V. nashicola on pear, and V. carpophila on peach and apricot, have characterized sexual stages in the field in at least some areas (Fisher Citation1961; Umemoto Citation1990; MacHardy Citation1996; González-Domínguez et al. Citation2017). Ascospores of V. nashicola may play a bigger role in epidemics of pear scab than previously thought (Lian et al. Citation2006, Citation2007). In the case of V. inaequalis, the sexual stage is often the primary source for the epidemic (MacHardy Citation1996), although conidia may also contribute (Passey et al. Citation2017). Control measures for apple scab in some areas are aimed at preventing the primary inoculum by ascospores to reduce impact of early infection on apple (Holb Citation2006).
Current management practices for scab on pecan rely on the use of fungicides (Bock et al. Citation2017a). Fungicide resistance is already documented for V. effusa to benomyl (Littrell Citation1976) and the demethylation inhibitors (DMIs) (Reynolds et al. Citation1997). More recent work indicates that there is reduced sensitivity to tin-based products (Standish et al. Citation2018), and a risk of resistance development to quinone outside inhibitors (QoIs) through specific mutations other than the more common G143A substitution (glycine to alanine substitution at position 143) may exist (Standish et al. Citation2016). Thus, there is reason for serious concern that fungicide resistance may become an increasingly greater issue based on what we currently know about the genetic diversity of V. effusa populations. In order to develop effective disease management strategies for pecan scab, the disease cycle must be well understood. The genetic diversity (Bock et al. Citation2017b) and the equilibrium of the mating type idiomorphs (Bock et al. Citation2018; Young et al. Citation2018) suggest that sexual reproduction occurs in populations of V. effusa across the southeastern United States; therefore, we postulate a role for ascospores in the epidemiology of V. effusa. Knowing the timing of production and infection process of ascospores and their role in the epidemiology of pecan scab may prove decisive in aiding management of this devastating disease.
In this study, we present in vitro evidence of the sexual stage of V. effusa. We describe the methodology to produce the sexual stage and utilize microscopy to demonstrate the developmental stages of the pseudothecium. In addition, we show viability of ascospores and evidence of recombination through sexual reproduction by evaluating progeny for segregating inheritance of a phenotypic marker, mating type, and microsatellite markers.
MATERIALS AND METHODS
Isolates
Sixteen isolates of V. effusa were used in this study (). Seven isolates were previously characterized in a population study (Bock et al. Citation2017b). One albino isolate (FRT5LL7-Albino, a natural variant selected as an albino sector from a wild-type, melanized isolate, FRT5LL7) was isolated from a commercial orchard of the pecan cultivar Desirable near southwest Albany, Georgia, and the remaining eight isolates were sampled from scab-diseased leaves or stems of pecan from orchards in Oklahoma and Texas. Methods for isolation have been previously described in detail (Bock et al. Citation2017b). Briefly, monoconidial isolates were obtained from individual lesions by spreading a conidial suspension on water agar (WA) amended with antibiotics (lactic acid [0.50 mL/L], streptomycin [0.20 g/L], tetracycline [0.05 g/L], and chloramphenicol [0.05 g/L]) and incubating for 1 d at 25 C. Under a microscope (50×), a single germinating conidium was carefully removed and transferred to antibiotic amended (as for WA) potato dextrose agar (PDA; HiMedia, Mumbai, India). Isolates were maintained on both PDA and oatmeal agar (OMA; 30 g Gerber’s organic baby oatmeal and 20 g agar per liter) at 25 C with a 12-h photoperiod; suspensions were prepared for long-term storage on silica at −20 C (Sinclair and Dhingra Citation1985).
Table 1. Venturia effusa isolates used in this study.
DNA extraction and PCR
Mycelium was scraped from a 2–3-wk-old colony of V. effusa growing on PDA and DNA was extracted using the Quick-DNA Fungal/Bacterial Miniprep Kit (Zymo Research, Irvine, California) according to the manufacturer’s instructions. Primers for mating type (MAT1-1 and MAT1-2) and the housekeeping gene (TUB2) were multiplexed as previously described (Young et al. Citation2018) to identify the mating type for each isolate.
Mating assay
Isolates of opposite mating types, and self and non-self-same mating types, were paired on OMA and PDA on 9-cm quadrant Petri plates (). Preliminary pairings were established by placing adjacent plugs of agar with mycelium of each mating type on OMA (). Once the sexual stage had been observed, the preliminary methodology was improved for future pairings using ground inoculum. The ground inoculum was prepared by scraping mycelium from a 2–3-wk-old colony of V. effusa grown on PDA and placed in a 2-mL tube with three 3-mm glass beads and 500 µL of sterile potato dextrose broth. The samples were subject to grinding using a Qiagen TissueLyser (Qiagen, Valencia, California) at 30 Hz for 6–9 min. Three inoculation points for each isolate per quadrant were made by pipetting 2 µL of inoculum onto the OMA (). The preliminary pairings using plugs of agar were placed in a 24 C incubator with a 12-h photoperiod for 4–8 wk. When using the ground-mycelium drop inoculation methodology, the pairings were placed in the 24 C incubator for 2 wk. Once the paired isolates had sufficiently grown for hyphal overlap and interactions, the plates were wrapped in aluminum foil and placed at 4 C for 4 mo. After the 4-mo cold treatment, the interaction zone between the paired isolates was checked for any evidence of sexual fruiting structures, and the plates were placed in an incubator at 24 C with a 12-h photoperiod. The plates were checked for evidence of sexual fruiting structures every few days. Pseudothecia were observed using a dissecting microscope and randomly selected from the zone between opposite mating types. To observe maturation status, pseudothecia were observed with a Leica CME microscope at 40× magnification (Leica, Buffalo Grove, Illinois). Slight pressure was applied using a cotton swab to split the pseudothecium to evaluate the maturity of the ascocarp (immature = no asci; partially mature = contents contain some asci with and without ascospores; fully mature = contents contain many asci with ascospores). A graphic timeline of the experimental conditions required for in vitro sexual reproduction in V. effusa is presented ().
Table 2. Crosses of Venturia effusa.
Effect of time on pseudothecial development
Pairings were repeated to observe the effect of the length of cold treatment. Pairings were exposed to 1, 2, 3, or 4 mo of cold treatment at 4 C, before transfer to an incubator at 24 C with a 12-h photoperiod for 2 wk when they were observed for pseudothecial development. Pseudothecia were examined, and ascospore maturation was assessed by squash as described above.
Sectioning for microscopy
Agar blocks (ca. 5 × 7 mm) containing pseudothecia were taken and frozen in liquid nitrogen. The block was mounted and embedded in Tissue-Tek O.C.T. Compound (Electron Microscopy Sciences, Hatfield, Pennsylvania). Twenty-micrometer sections were cut on a Leica CM1850 cryostat (Leica Biosystems, Buffalo Grove, Illinois) and mounted on VWR microscope slides (VWR International, Radnor, Pennsylvania). The sections were stored at −20 C until processing when they were stained with 0.05% Toluidine Blue O (Sigma, St. Louis, Missouri) in distilled water for 3–5 min. Cross- and longitudinal sections were observed under an Olympus BX41 microscope using LUC Plan FL N 40×/0.60 NA (numerical aperture) and Plan N 100×/1.25 NA (oil) objectives. Images were captured using an Olympus DP71 CCD camera with DP Controller software (Olympus America, Center Valley, Pennsylvania). Subsequently, selected sections were observed using Zeiss Axio Imager M2 upright microscopes (Carl Zeiss, Jena, Germany) with Plan-Neofluar 40×/0.75 NA and Plan-Neofluar 100×/1.30 NA (oil) objectives equipped with differential interference contrast (DIC) optics. Images were captured using an Axiocam 503 mono camera (Carl Zeiss) with ZEN 2 (Blue edition) image acquisition and processing software.
Isolation of ascospore progeny
Three crosses were chosen for ascospore isolation (NFVe23 × NFVe24, FRT5LL7-Albino × NFVe47, and FRT5LL7-Albino × NFVe78). Individual pseudothecia were squashed, and the contents were plated on 2% water agar. Single germinating ascospores were arbitrarily isolated using a microdissection needle and transferred to PDA and grown at 24 C with a 12-h photoperiod. Ascospore progeny were stored on silica at −20 C (Sinclair and Dhingra Citation1985).
DNA extraction and multilocus genotyping of ascospore progeny
Isolates were grown on PDA for 2–3 wk. DNA was extracted from freeze-dried fungal mycelium using the Qiagen MagAttract 96 DNA Plant Core Kit according to the manufacturer’s protocol. Parental and single-ascospore progeny were screened for polymorphisms using 32 microsatellite markers as described previously (Bock et al. Citation2016a, Citation2017b). Polymerase chain reaction (PCR) was performed in a 10 μL volume containing template DNA (approximately 2 ng), 0.5 U GoTaq DNA polymerase (Promega, Madison, Wisconsin), 1× Colorless GoTaq PCR buffer (Promega), 150 nM of each dNTP (Promega), 100 nM of the reverse primer, 25 nM of the M13-tagged forward primer, and 100 nM of the dye-labeled M13 forward primer. The cycling parameters were an initial denaturation step for 1 min at 94 C, 33 cycles of denaturation at 94 C for 40 s, annealing at 58 C for 40 s, and extension at 72 C for 20 s, followed by a final synthesis step at 72 C for 30 min. PCR products were pooled based on the fluorescent phosphoramidite dyes used (6-carboxyfluorescein [6-FAM], 2′-chloro-7′phenyl-1,4-dichloro-6-carboxyfluorescein [VIC], 2′-chloro-5′-fluoro-7′,8′-benzo-1,4-dichloro-6-carboxyfluorescein [NED], PET [unpublished proprietary dye of Applied Biosystems, Foster City, California]) to a 1:10 dilution. The pooled PCR products were added to 9.9 μL of Hi-Di formamide and 0.1 μL of GeneScan 500 LIZ size standard (Life Technologies, Carlsbad, California). Samples were denatured at 94 C for 5 min. Microsatellite fragments were analyzed on an ABI 3730 DNA analyzer. Analysis was performed using Peak Scanner Software 1.0 (Applied Biosystems). The 32 markers were successfully scored in the crosses except for two markers, Fe176_AGG12-F and Fe179_CTT6-F, due to mismatches in the parental strains NFVe47 and FRT5LL7-Albino. Each progeny colony was also tested for mating type as described above using a multiplex reaction including the housekeeping gene TUB2 as previously described (Young et al. Citation2018).
The proportion of MAT1-1-1 and MAT1-2-1 isolates was tested for equilibrium (the null hypothesis being 1:1 ratio) using a χ2 test to determine whether the proportions departed significantly from a 1:1 ratio (Everitt Citation1992). Due to the limitations of the χ2 test when analyzing small sample sizes (generally populations with expected values <5), an exact binomial test (two-tailed) for goodness-of-fit was used to determine whether observed mating type frequencies within populations deviated from the 1:1 ratio. Analyses of mating type data were performed using SAS 9.4 (SAS Institute, Cary, North Carolina).
Identification of the mutation associated with the albino phenotype
Genes involved in melanin biosynthesis were identified from the Venturia effusa genome sequence of the melanin-producing isolate 3Des10b (Bock et al. Citation2016b) using tblastn with orthologs from the Alternaria alternata melanin gene cluster (Kimura and Tsuge Citation1993; Fetzner et al. Citation2014). Three genes, PKS1 (encoding a polyketide synthase, with 79% similarity to ALM1 [PKSA]), CRM1 (encoding a C2H2 Zn2+ finger transcription factor, with 71% similarity to CRMA), and BRM2 (encoding a T3HN reductase, with 85% similarity to BRM2), were located in a gene cluster in a similar orientation to A. alternata. Comparison of the melanin biosynthesis gene sequences of 3Des10b with those of FRT5LL7-Albino revealed a single nucleotide insertion in the PKS1 melanin biosynthesis gene encoding a polyketide synthase (), which is the likely causative mutation of the albino phenotype (Winter et al. Citation2020). Progeny from the crosses with the FRT5LL7-Albino parent were evaluated to confirm that the PKS1 mutation co-segregated with the albino phenotype. DNA from each of the parents and the progeny were amplified with the primers alm-F (5′-CAGTGTGATGGCCTTGACTATCAG-3′) and alm-R (5′-TTCTCCAACAAGTCCCAG-3′), which results in a 583-bp wild-type fragment spanning the proposed causative mutation. The PCR products were cleaned up using QIAquick PCR Purification Kit (Qiagen) according to the manufacturer’s instructions and then direct sequenced using Sanger sequencing technology in the Genomics Core Facility, Noble Research Institute.
RESULTS
Development of the mating assay
Mating type for six isolates was previously identified (Young et al. Citation2018), and the remaining 10 isolates were characterized as MAT1-1 or MAT1-2 based on multiplex PCR (). Different isolates representing MAT1-1 and MAT1-2 types were paired () and observed for production of pseudothecia. Fruiting bodies were never observed on PDA. Immature fruiting bodies were observed after 6 mo of incubation (2 mo at 24 C and 4 mo at 4 C) during the preliminary experiments on oatmeal agar (). Once transferred to 24 C with a 12-h photoperiod, maturation of the pseudothecia occurred, and mature asci and ascospores were observed (). The squash mounts of the pseudothecia confirmed maturation by the presence of fully developed asci and ascospores. Pseudothecia were observed between all paired isolates of opposite mating types. Pseudothecia never formed between self or non-self-same mating type pairings. Pseudothecia formed on the bottom, top, and embedded throughout the agar between isolates of opposite mating types, regardless of the orientation of the Petri dish during incubation. Depending on the isolate, some pseudothecia would form closer to one parent over the other, which may indicate differences in fertility between isolates.
Figure 2. Zone observed between pairings of Venturia effusa after 4 mo of cold treatment (4 C). A. Same mating types (ALB1L5 × ALB1L5) with no ascocarps present. B. opposite mating types (ALB1L5 × ALB2L1). Pseudothecia indicated by arrows.
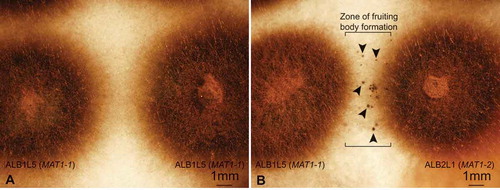
Figure 3. Sexual reproductive structures in Venturia effusa. A. DIC image of a section of a pseudothecium from a cross between NFVe23 (MAT1-1) × NFVe24 (MAT1-2), 3 wk at 24 C, 12-h photoperiod, post cold incubation, with ascospores in asci. B. DIC image of a section of a pseudothecium from NFVe23 (MAT1-1) × NFVe49 (MAT1-2), 5 wk at 24 C, 12-h photoperiod, post cold incubation. C, D. Squash mount of pseudothecium from NFVe23 (MAT1-1) × NFVe49 (MAT1-2), 6 wk at 24 C following 4 C incubation. Numerous asci with mature asci and ascospores. Bars: A = 10 µm; B = 20 µm; C, D = 20 µm.
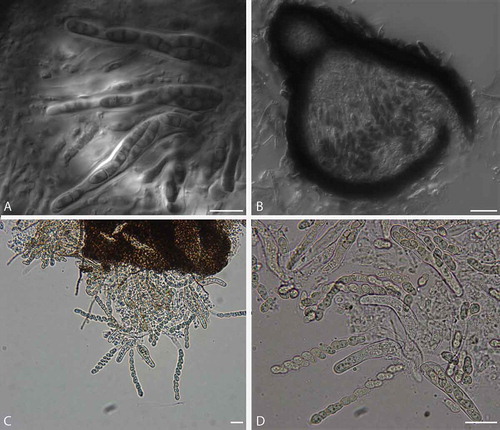
The morphology of the pseudothecia, asci, and ascospores observed were consistent with descriptions of the genus Venturia (Von Arx Citation1952; Zhang et al. Citation2011) (). Orientation of the ascospores in each ascus is similar to that in V. pyrina rather than V. inaequalis, with the larger end of the spore facing toward the ostiole. Ascospores of V. effusa, like those of V. pyrina, are septate in the lower third, in contrast to V. inaequalis ascospores, which are septate in the upper third (Barr Citation1968). Pseudothecia from V. inaequalis have been described as being with or without setae (MacHardy Citation1996) and may have setae surrounding the ostiole according to Gadoury and MacHardy (Citation1985). We observed pseudothecia of V. effusa with and without setae ().
Figure 4. Pseudothecia of Venturia effusa with and without setae from crosses between opposite mating types. Pseudothecia developed in the oatmeal agar; therefore, hyphae were typically present on all pseudothecia, whereas setae can be distinguished from hyphae as highly pigmented bristle like hairs. A, B. Examples of setae observed on pseudothecia. C, D. Examples of pseudothecia without setae. Bars: A, B = 50 µm; C, D = 100 µm.
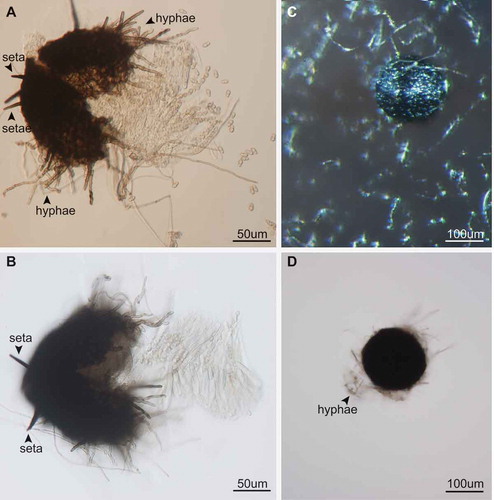
Pseudothecial development is affected by time
Observations were made to assess the development of pseudothecia and the maturation of asci after varying the length of the cold treatment. The pairings observed after 1 mo of cold treatment showed no signs of fruiting bodies or fruiting body initials. The pairings observed after 2 mo of cold treatment had both pseudothecia and smaller “hyphal knots,” which may be protopseudothecia. The pseudothecia appeared to remain immature or partially mature, with few ascospores observed. All pairings observed after 4 mo had some asci with ascospores. The pseudothecia became more mature (increase in number of asci with ascospores) after a minimum 2 wk of incubation and up to 6 wk at 24 C.
Multilocus genotyping of ascospore progeny confirmed sexual recombination
Progeny from independent sexual crosses were evaluated to determine whether sexual reproduction produced recombinant progeny (). A total of 32, 13, and 30 single-ascospore progeny were isolated from three crosses between isolates NFVe23 (cv. Pawnee) × NFVe24 (native), FRT5LL7-Albino (cv. Desirable) × NFVe47 (cv. Choctaw), and FRT5LL7-Albino (cv. Desirable) × NFVe78 (cv. Pawnee), respectively. The percentages of unique multilocus genotypes for NFVe23 × NFVe24, FRT5LL7-Albino × NFVe47, and FRT5LL7-Albino × NFVe78 were 88% (4 possible clones), 100% (no possible clones), and 93% (2 possible clones), respectively. The mating type ratios (MAT1-1:MAT1-2) were 17:15, 9:4, and 14:16. Mating types of all three crosses were in gametic equilibrium based on both the χ2 test and the exact binomial test (). The phenotype ratios (albino:wild type) were 5:8 and 10:20 and considered to be in statistical equilibrium (data not shown). The numerical imbalance in phenotype frequency could be due to wild-type spores being preferentially selected over albino spores, perhaps due to difficulty observing hyaline, albino spores on the medium.
Table 3. Genotypes of ascospore progeny produced from in vitro sexual crosses using 32 microsatellite markers, and mating type and phenotype if applicable.
Table 4. The mating type frequencies of the different crosses of isolates of Venturia effusa.
Confirmation of the causative mutation for the albino phenotype
The proposed causative mutation for the albino phenotype was identified as a single-base insertion in the PKS1 gene of the melanin biosynthesis gene cluster () located on chromosome 6 of the FRT5LL7-Albino genome sequence (Winter et al. Citation2020). All albino progeny (n = 15) have the identical single-base insertion found in PKS1, which was not observed in melanized isolates, confirming that this mutation is the likely cause of the albino phenotype.
DISCUSSION
This is the first report of successful in vitro mating of V. effusa through direct contact of isolates of opposite mating types on OMA. We demonstrated reproducible production of pseudothecia and mature, viable ascospores between isolates of opposite mating types from different locations and pecan cultivars (5 crosses between isolates from the same cultivars, 5 crosses between isolates from different cultivars). Venturia effusa was able to complete the sexual cycle after a minimum 2 mo of cold treatment, but a greater number of asci matured after a 4-mo cold treatment. Maturation of asci and ascospores did require an increase in temperature for a minimum of 2 wk after the cold period, with an increase in the number of mature asci as the incubation time at 24 C increased (observed up to 6 wk at 24 C).
Ascospore viability was confirmed, and genotyping the progeny demonstrated genetic recombination through sexual reproduction. Evidence that V. effusa can reproduce sexually supports the population studies that have identified moderate to high genetic diversity along with mating type ratios in equilibrium (Bock et al. Citation2017b, Citation2018; Young et al. Citation2018). The progeny from the crosses revealed unique multilocus genotypes based on the microsatellite analysis where the locations of the microsatellite markers are known to be distributed across multiple chromosomes (Winter et al. Citation2020). These observations support (and provides a basis for) the results from studies of field populations of V. effusa showing characteristics of population genetic diversity and structure suggestive of sexual reproduction. The recombinant genotypes resulting from sexual reproduction could lead to a more adaptable fungal population (McDonald and Linde Citation2002; McDonald and Mundt Citation2016).
In addition to increasing our understanding of the pathogen life cycle, we have now developed a valuable tool to establish mapping populations for genetic mapping of genes of interest associated with specific traits. The use of an albino isolate provided the first proof of concept through the visualization of a phenotypic segregating trait. As expected, the observed mutation in the FRT5LL7-Albino parent was found in each of the albino progeny. However, the expected phenotype equilibrium was lower than expected, likely due to the efficiency of picking albino isolates from a pale medium. Identifying genes associated with traits such as virulence and fungicide resistance would be valuable to map. Previous studies have shown that V. effusa isolates exhibit variation in virulence, which may suggest that a possible pathogen race structure exists (Conner Citation2002; Conner and Stevenson Citation2004). Quantitative trait locus mapping of virulence traits in the wheat–Zymospetoria tritici system revealed that virulence could be broken down into traits involved with host damage and pathogen reproduction (Stewart et al. Citation2018). Combining genetic mapping with the availability of a complete V. effusa reference genome (Winter et al. Citation2020) will facilitate identification of genes associated with cultivar specificity.
Researchers have focused their efforts on studying pseudothecia formation and ascospore discharge to better understand the role of ascospores. Ascospores of Venturia species can play an important role not just as a vehicle of novel diversity but also as a component of the epidemiology of the disease (González-Domínguez et al. Citation2017). Studies from other Venturia species may aid in identifying the role of ascospores in the epidemiology of pecan scab. For example, conidia of Venturia nashicola have been considered the primary inoculum for pear scab, but recent studies suggest that ascospores may play a more important role in the epidemiology of this disease. Results showed that pseudothecia formation in pear scab in northern China was influenced by rain events in either winter or early spring, with ascospore release occurring by late May (Lian et al. Citation2006). Further studies by (Lian et al. Citation2007) found that free water or 100% relative humidity was required for ascospore release, with approximately 80% of ascospores being released within the first hour after being wetted. Similarly, ascopores of V. inaequalis play a significant role in epidemics of apple scab (MacHardy Citation1996), and some control measures can effectively target the sexual stage (Holb Citation2006).
In vitro sexual reproduction in V. inaequalis may have similar requirements to that identified for V. effusa, but these findings have not been effectively reproduced. Early studies showed that pseudothecia of V. inaequalis would form in culture on OMA when stored at 10 C for 5 mo (Jones Citation1914a, Citation1914b). However, others have been unable to produce ascospores in vitro without using apple leaves and have only seen pseudothecia initials on oatmeal agar (Rudloff Citation1934; Schmidt Citation1935; Herbst et al. Citation1937). Infected leaves have been used under controlled growth conditions to determine environmental requirements. Moisture and temperature were factors in pseudothecial development in V. inaequalis on apple in North Carolina (James and Sutton Citation1982). In addition, moisture and temperature from February to April influenced the maturation of pseudothecia, with the optimum temperature ranges of 8–12 C for ascogonial development and 16–18 C for ascospore maturation (James and Sutton Citation1982). Earlier studies found that production of pseudothecia was greatest at 4 C and that of asci without ascospores was greatest at 15 C (Ross and Hamlin Citation1962). With the identification of a sexual stage in V. effusa, disease management may now align more closely to management practices for apple scab.
If the V. effusa life cycle is similar to those of V. inaequalis and V. nashicola, the source of overwintering pseudothecia would be on dead pecan leaves. An ascomycetous fungus has been observed on dead pecan leaves, but the fungus was not identified (Demaree Citation1924). In Oklahoma, we have observed initial infections on the abaxial side of leaves lowest in the canopy (approximately 2 m) in early May to early June, which leads us to hypothesize that the inoculum source may in fact be on the orchard floor. Demaree (Citation1924) did note an evident reduction in scab after a fire moved through a heavily infected orchard, as well as control of the disease in plowed orchards where the leaf, twig, and shuck debris were buried under the soil compared with unplowed orchards. We have identified an ascomycetous fungus, Mycosphaerella, in the pecan leaf litter (Charlton and Young, unpublished), which has introduced an additional challenge to identifying the role of V. effusa ascospores in the disease cycle of pecan scab. Mycosphaerella carygena, cause of downy spot, and V. effusa appear to have morphologically similar pseudothecia and ascospores. The timing of M. carygena ascospore release has been established as early spring, early April, prior to budbreak (Goff et al. Citation1987), around the same time we would expect V. effusa ascospores to be released. The morphological characteristics of the culture in vitro can be used to distinguish V. effusa and M. carygena but can take up to 1 wk to appear. Due to the similarity of the ascospores, it will likely be challenging to identify the role of the sexual spores in the epidemiology of pecan scab.
The discovery of the sexual stage of V. effusa could have profound implications on management strategies to control pecan scab. Additional studies are required to determine the optimal temperature ranges for pseudothecial development and ascus maturation in V. effusa in vitro. Future studies that focus on the time and environmental factors required to initiate and develop mature pseudothecia could provide more definitive requirements for the sexual cycle and ascospore release under field conditions. Identification of where V. effusa overwinters and the location of pseudothecial development is critical to developing management strategies to reduce the primary inoculum in the disease cycle, as has been described for V. inaequalis. With further knowledge of the sexual stage of V. effusa in the field, we have an opportunity to develop additional science-based management tools to control scab in pecan.
Supplemental Material
Download PDF (470.3 KB)ACKNOWLEDGMENTS
We thank Jin Nakashima and the Cellular Imaging Core Facility, Noble Research Institute, for technical support and Rachael Davis, Noble Research Institute, for graphic design. The authors also wish to thank Basil Savage for allowing access to his orchard to make observations and collect samples throughout the year, as well as Dr. Charles Rohla, Noble Research Institute, for helping collect samples.
Supplemental Material
Supplemental data for this article can be accessed on the publisher’s website.
Additional information
Funding
LITERATURE CITED
- Barr ME. 1968. The Venturiaceae in North America. Canadian Journal of Botany 46:799–864.
- Bock C, Brenneman T, Wood B, Stevenson K. 2017a. Challenges of managing disease in tall orchard trees-pecan scab, a case study. CAB Reviews 12:1–18.
- Bock C, Chen C, Yu F, Stevenson K, Arias R, Wood B. 2016a. Characterization of microsatellites in Fusicladium effusum, cause of pecan scab. Forest Pathology 46: 600–609.
- Bock CH, Chen C, Yu F, Stevenson KL, Wood BW. 2016b. Draft genome sequence of Fusicladium effusum, cause of pecan scab. Standards in Genomic Sciences 11:36.
- Bock CH, Hotchkiss MW, Young CA, Charlton ND, Chakradhar M, Stevenson KL, Wood BW. 2017b. Population genetic structure of Venturia effusa, cause of pecan scab, in the southeastern United States. Phytopathology 107:607–619.
- Bock CH, Young CA, Stevenson KL, Charlton ND. 2018. Fine-scale population genetic structure and within-tree distribution of mating types of Venturia effusa, cause of pecan scab in the United States. Phytopathology 108:1326–1336.
- Brock J, Brenneman TB. 2014. Pecan. In: Little EL, ed. Georgia plant disease loss estimates 2014. University of Georgia Extensions Annual Publication 102-7. Athens, Georgia: University of Georgia. p. 14.
- Conner PJ. 2002. A detached leaf technique for studying race-specific resistance to Cladosporium caryigenum in pecan. Journal of the American Society for Horticultural Science. 127:781–785.
- Conner PJ, Stevenson KL. 2004. Pathogenic variation of Cladosporium caryigenum isolates and corresponding differential resistance in pecan. HortScience 39:553–557.
- Demaree JB. 1924. Pecan scab with special reference to sources of the early spring infections. Journal of Agricultural Research 28:321–333.
- Everitt BS. 1992. The analysis of contingency tables. London: Chapman and Hall/CRC. 168 p.
- Fetzner R, Seither K, Wenderoth M, Herr A, Fischer R. 2014. Alternaria alternata transcription factor CmrA controls melanization and spore development. Microbiology 160:1845–1854.
- Fisher EE. 1961. Venturia carpophila sp. nov., the ascigerous state of the apricot freckle fungus. Transactions of the British Mycological Society 44:337–342.
- Gadoury DM, MacHardy WE. 1985. Negative geotropism in Venturia inaequalis. Phytopathology 75:856–859.
- Gladieux P, Zhang X-G, Afoufa-Bastien D, Sanhueza R-MV, Sbaghi M, Le Cam B. 2008. On the origin and spread of the scab disease of apple: out of Central Asia. PLoS ONE 3:e1455.
- Gladieux P, Zhang X-G, Róldan‐Ruiz I, Caffier V, Leroy T, Devaux M, Van Glabeke S, Coart E, Le Cam B. 2010. Evolution of the population structure of Venturia inaequalis, the apple scab fungus, associated with the domestication of its host. Molecular Ecology 19:658–674.
- Goff W, Drye C, Miller R. 1987. Ecology and epidemiology of pecan downy spot. Phytopathology 77:491–496.
- González-Domínguez E, Armengol J, Rossi V. 2017. Biology and epidemiology of Venturia species affecting fruit crops: a review. Frontiers in Plant Science, doi:10.3389/fpls.2017.01496
- Gottwald T. 1982. Spore discharge by the pecan scab pathogen, Cladosporium caryigenum. Phytopathology 72:1193–1197.
- Gottwald T. 1985. Influence of temperature, leaf wetness period, leaf age, and spore concentration on infection of pecan leaves by conidia of Cladosporium caryigenum. Phytopathology 75:190–194.
- Gottwald T, Bertrand P. 1982. Patterns of diurnal and seasonal airborne spore concentrations of Fusicladium effusum and its impact on a pecan scab epidemic. Phytopathology 72:330–335.
- Gottwald T, Bertrand P. 1983. Effect of time of inoculation with Cladosporium caryigenum on pecan scab development and nut quality. Phytopathology 73:714–718.
- Herbst 55W, Rudloff C, Schmidt M. 1937. Vergleichend-morphologische Studien an verschiedenen Venturiaarten. Die Gartenbauwissenschaft 11:183–207.
- Holb IJ. 2006. Effect of six sanitation treatments on leaf litter density, ascospore production of Venturia inaequalis and scab incidence in integrated and organic apple orchards. European Journal of Plant Pathology 115:293–307.
- James J, Sutton T. 1982. Environmental factors influencing pseudothecial development and ascospore maturation of Venturia inaequalis. Phytopathology 72:1073–1080.
- Jones FR. 1914a. Perithecia in cultures of Venturia inaequalis. Phytopathology 4:52–53.
- Jones FR. 1914b. Is the Venturia on the Wisconsin wild crab, Pyrus coronaria, identical with the Venturia inequalis of the cultivated apple [Master's Thesis]. Wisconsin, Madison: University of Wisconsin, Madison. 154 p.
- Kimura N, Tsuge T. 1993. Gene cluster involved in melanin biosynthesis of the filamentous fungus Alternaria alternata. Journal of Bacteriology 175: 4427–4435.
- Lian S, Li BH, Dong XL, Li BD, Xu XM. 2007. Effects of environmental factors on discharge and germination of ascospores of Venturia nashicola. Plant Pathology 56:402–411.
- Lian S, Li BH, Xu XM. 2006. Formation and development of pseudothecia of Venturia nashicola. Journal of Phytopathology 154:119–124.
- Linde CC, Zhan J, McDonald BA. 2002. Population structure of Mycosphaerella graminicola: from lesions to continents. Phytopathology 92:946–955.
- Littrell R. 1976. Resistant pecan scab strains to benlate and pecan fungicide management. Pecan South 3:335–337.
- MacHardy WE. 1996. Apple scab: biology, epidemiology, and management. St. Paul, Minnesota: American Phytopathological Society (APS Press). 545 p.
- McDonald BA, Linde C. 2002. Pathogen population genetics, evolutionary potential, and durable resistance. Annual Review of Phytopathology 40:349–379.
- McDonald BA, McDermott JM. 1993. Population genetics of plant pathogenic fungi. Bioscience 43:311–319.
- McDonald BA, Mundt CC. 2016. How knowledge of pathogen population biology informs management of Septoria tritici blotch. Phytopathology 106:948–955.
- McDonald BA, Pettway RE, Chen R, Boeger J, Martinez J. 1995. The population genetics of Septoria tritici (teleomorph Mycosphaerella graminicola). Canadian Journal of Botany 73:292–301.
- Milgroom MG. 1996. Recombination and the multilocus structure of fungal populations. Annual Review of Phytopathology 34:457–477.
- Passey T, Robinson J, Shaw M, Xu XM. 2017. The relative importance of conidia and ascospores as primary inoculum of Venturia inaequalis in a southeast England orchard. Plant Pathology 66:1445–1451.
- Reynolds KL, Brenneman TB, Bertrand PF. 1997. Sensitivity of Cladosporium caryigenum to propiconazole and fenbuconazole. Plant Disease 81:163–166.
- Ross R, Hamlin S. 1962. Production of perithecia of Venturia inaequalis (Cke.) Wint. on sterile apple leaf discs. Canadian Journal of Botany 40:629–635.
- Rossman AY, Allen WC, Castlebury LA. 2016. New combinations of plant-associated fungi resulting from the change to one name for fungi. IMA Fungus 7:1–7.
- Rudloff C. 1934. Venturia inaequalis (Cooke) Aderhold. III. Zur Formenmannigfaltigkeit des Pilzes. Die Gartenbauwissenschaft 9:105–119.
- Schmidt M. 1935. Venturia inaequalis (Cooke) Aderhold. IV. Weitere Beiträge zur Rassenfrage beim Erreger des Apfelschorfes. Die Gartenbauwissenschaft 9:364–389.
- Schubert K, Ritschel A, Braun U. 2003. A monograph of Fusicladium s. lat. (Hyphomycetes). Schlechtendalia 9:1–132.
- Sinclair JB, Dhingra OD. 1985. Basic plant pathology methods. Boca Raton, Florida: CRC Press. 355 p.
- Standish J, Avenot H, Brenneman T, Stevenson K. 2016. Location of an intron in the cytochrome b gene indicates reduced risk of QoI fungicide resistance in Fusicladium effusum. Plant Disease 100:2294–2298.
- Standish J, Brenneman T, Stevenson K. 2018. Dynamics of fungicide sensitivity in Venturia effusa and fungicide efficacy under field conditions. Plant Disease 102:1606–1611.
- Stevenson K, Bertrand P. 2001. Within-season dynamics of yield loss due to pecan scab fruit infections. Phytopathology 91:S85.
- Stewart EL, Croll D, Lendenmann MH, Sanchez‐Vallet A, Hartmann FE, Palma‐Guerrero J, Ma X, McDonald BA. 2018. Quantitative trait locus mapping reveals complex genetic architecture of quantitative virulence in the wheat pathogen Zymoseptoria tritici. Molecular Plant Pathology 19:201–216.
- Umemoto S. 1990. Infection sources in Japanese pear scab (Venturia nashicola) and their significance in the primary infection. Japanese Journal of Phytopathology 56:658–664.
- Von Arx J. 1952. Studies on Venturia and related genera. Tijdschrift over Plantenziekten 58:260–266.
- Wells L. 2017. Pecan: America’s native nut tree. Tuscaloosa, Alabama: University of Alabama Press. 264 p.
- Winter DJ, Charlton ND, Krom N, Shiller J, Bock CH, Cox MP, Young CA. 2020. Chromosome-level reference genome of Venturia effusa, causative agent of pecan scab. Molecular Plant-Microbe Interactions 33:149–152.
- Winter G. 1885. New North American fungi. The Journal of Mycology 1:101–102.
- Wood BW, Payne JA, Grauke LJ. 1990. The rise of the US pecan industry. HortScience 25:594–723.
- Young CA, Bock CH, Charlton ND, Mattupalli C, Krom ND, Bowen JK, Templeton MD, Plummer K, Wood BW. 2018. Evidence for sexual reproduction: identification, frequency and spatial distribution of Venturia effusa (pecan scab) mating type idiomorphs. Phytopathology 108:837–946.
- Zhang Y, Crous PW, Schoch CL, Bahkali AH, Guo LD, Hyde KD. 2011. A molecular, morphological and ecological re-appraisal of Venturiales―a new order of Dothideomycetes. Fungal Diversity 51:249–277.