Abstract
As part of state-of-environment reporting, soil quality monitoring in Auckland has indicated that decreasing macroporosity is a concern for grazed pasture. This prompted a more intensive study undertaken in 2011 to resample the original 35 sites. Comparisons were made between Soil Orders and land uses, with soil samples taken from all repeat sites and under adjacent/ungrazed fence-lines to act as a control. Macroporosity was significantly less for grazed/tread (7%) than ungrazed/control (15%) treatments. However, macroporosity in the control treatment was only just within recommended guidelines for the Ultic Soil Order, representing >40% of Auckland's soils. The study found that what were originally described as two land use categories; dairy and drystock, increased to four categories; dairy, dairy-drystock conversion, drystock, and lifestyle conversion. Although 83% of dairy sites occupied what would be considered prime land, a large proportion of prime land was also occupied by lifestyle sites (64%), followed by dairy-drystock (38%) and drystock (30%). Such information raises two concerns: first, that a large proportion of prime land is not being used for commercial primary production; and second, the difficulty in developing soil quality trends as a result of land use changes.
Introduction
Over recent years, the detrimental impacts on soil physical quality resulting from livestock grazing have become of increasing concern in New Zealand (e.g. Drewry et al. Citation2008; Curran-Cournane Citation2010; Houlbrooke et al. Citation2011) and worldwide (e.g. Bilotta et al. Citation2007; Savadogo et al. Citation2007; Cattle & Southorn Citation2010; Kumbasli et al. Citation2010). The implications of declining soil physical quality are well documented and have both environmental and agronomic repercussions. Hoof action can deplete pasture density, particularly when soil is close to or above field capacity, and a depleted sward is less able to trap soil particles (Nash & Halliwell Citation1999). This promotes the generation of soil erosion and associated contaminants in surface runoff and subsequently deteriorates surface water quality (McDowell et al. Citation2008). Livestock grazing can also compact soil, reducing subsequent regrowth and yield potential (Edmond Citation1966; Drewry et al. Citation2008).
Macroporosity, a measurement of large pore space in soil and therefore soil compaction, has proven to be the most sensitive indicator for both environmental and agronomic responses. Relationships between macroporosity and concentrations of phosphorus and suspended sediment in surface runoff have been reported (McDowell et al. Citation2003; Curran Cournane et al. Citation2011a). Furthermore, Drewry et al. (Citation2004) associated a 1.6% increase in spring relative pasture yield with a unit increase in macroporosity (−10 kPa) at a soil depth of 0–5cm for four New Zealand Soil Orders, namely Brown, Gley, Pallic and Recent.
Macroporosity is also a key soil quality indicator as part of the ‘500 Soils Project’ instigated in 1995 for regional councils nationwide to undertake as part of the state-of-environment (SoE) reporting (Hill et al. Citation2003). Other key soil quality indicators include total carbon (TC), total nitrogen (TN), Olsen P, bulk density, pH and anaerobic mineralizable nitrogen. For pastoral-based systems within the Auckland region, a recurring concern is soil compaction indicated by macroporosity (determined at both −5 and −10 kPa). Macroporosity averaged 4% at −5 kPa and 8% at −10 kPa for combined dairy and drystock sites sampled during 2009–10 (Stevenson Citation2010; Fraser & Stevenson Citation2011). Macroporosity at the same sites sampled between 1995 and 2000 averaged 10% at −5 kPa (−10 kPa had not been measured during these periods) (Sparling Citation2009). The decreasing trend in soil physical quality in Auckland is of concern, particularly given that the mean values for macroporosity −10 kPa fall below the 10% v/v recommended guideline for both environmental and pasture health. The recommended guideline for macroporosity −5 kPa is 8% v/v (Sparling et al. Citation2003).
Many reports have been published on soil physical quality across several regions in New Zealand including Northland (Singleton et al. Citation2000), Waikato (Singleton & Addison Citation1999; Singleton et al. Citation2000; Zegwaard et al. Citation2000; Drewry Citation2003, et al. 2004; Taylor et al. Citation2010), Hawkes Bay (Betteridge et al. Citation1999), Manawatu (Climo & Richardson Citation1984), Canterbury (Drewry et al. Citation2001), North Otago (Houlbrooke et al. Citation2009, Citation2011; Curran Cournane et al. Citation2011b) and Otago and Southland (Drewry et al. Citation2000, Citation2004; Curran Cournane et al. Citation2010a,Citationb). In contrast, very little has been published on soil physical quality within the Auckland region, a region where about 90% of area is rural land.
Although there has been a pronounced change in land use over the past 20 years with the conversion of sheep farms to dairy farms across many parts of New Zealand (Monaghan et al. Citation2005), the picture is quite different within the Auckland region. The State of the Auckland Region (Auckland Regional Council Citation2010) reported that dairy cattle numbers declined by 23%, dairy farms by 33%, and dairy farmed land area (ha) declined by 25% between 2002 and 2008. Changes were also apparent for beef cattle and sheep numbers which have declined by 16% and 28%, respectively, from 2002 to 2008. Andrew & Dymond (Citation2012) reported that lifestyle blocks have expanded and cover 187,000 ha of land including 10% of New Zealand's high-class land, something that was also highlighted in the State of the Auckland Region report (Auckland Regional Council Citation2010).
The degree of soil physical damage is dependent on a range of factors such as antecedent soil moisture at time of grazing, livestock loading and inherent soil characteristics. Therefore, Soil Order has proven to be an indicator of the likely soil susceptibility to treading damage (Drewry et al. Citation2004; Curran Cournane et al. Citation2010b). In this study, sampling sites were located across seven contrasting Soil Orders, representative of the Auckland Region. The objective of this paper is to compare soil quality for grazed pasture over time (particularly soil physical quality) and to determine soil quality for both grazed and ungrazed conditions.
Material and methods
Study sites
This study took place across 35 sites within the Auckland region, located on 28 pastoral grazed, privately owned properties. The sites were visited from 03–19 October 2011. Twenty-one sites were located within North Auckland and 14 within South Auckland. The combination of sites represented seven contrasting Soil Orders, namely (with number of sites sampled in parentheses) Allophanic (3), Brown (6), Gley (4), Granular (7), Organic (4), Recent (2) and Ultic (9) and consisted of 18 genetic soil series () (Hewitt Citation1998). Sites were chosen on these particular soils because they are representative of grazing land within the Auckland region (Hicks Citation1995).
Table 1 Soil Series (with number of sites in parentheses), New Zealand Soil Classification (NZSC), S-map (family/sibling code), parent material and origin, soil drainage class, and site category (with number of sites in parentheses) (Hewitt Citation1998; Lilburne et al. Citation2011, Taylor Citation1954).
At the time of sampling in October 2011, 20 sites were ungrazed for >2 weeks, six sites ungrazed for >1 week and nine sites had been grazed within a week of sampling. Site land use and grazing management history were obtained for each site from landowners. Stocking rates varied between 9–18 stocking units/ha (Fleming Citation2003) across the 35 sites. Fence-lines adjacent to sampling sites were also sampled provided they had been established for more than 5 years (with one exception established approximately 3 years previously) and were no further than 40 m from the paired grazed site. Vegetation was predominantly a mix of ryegrass (Lolium sp.)/clover (Trifolium sp.) with kikuyu (Pennisetum sp.) pasture at some sites and moss species were apparent under some fence-lines. Grazed and fence-line sampled sites are hereafter referred to as tread and control treatments, respectively.
Tread pastoral sites are those previously monitored for soil quality as part of the nationwide ‘500 Soils Project’ (Hill & Sparling Citation2009) and had originally been sampled between 1995 and 2000 (hereafter referred to as 1995/00) and resampled during 2009–10 (hereafter referred to as 2009/10). These two sampling events were conducted in late winter/early spring in accordance with the Land and Soil Monitoring Guidelines (Hill & Sparling Citation2009). A pedologist involved in the original site selection helped to relocate sites in some instances while a GPS was used in 2009/10 to reference the sites for later sampling events. The pedologist also ascribed a land use capability (LUC) class to each of the sampled sites (Lynn et al. Citation2009). Nineteen of the 35 sites were reported as dairy land use and 16 as drystock during the original 1995/00 site selection process. When sites were resampled in the current study only six of the original 19 dairy sites were still dairy and only 10 of the original 16 drystock sites were still drystock. Eight of the 19 former dairy sites had been converted to drystock operations and five to lifestyle blocks. Similarly, six of the original 16 drystock sites had been converted to lifestyle blocks. The 11 lifestyle blocks were grazed predominantly by beef cattle alternating with horses and mixed livestock. One lifestyle site had been ungrazed by stock for more than 2 years. As a result of land use change, sites were divided into four categories for the current study: (i) dairy (n=6), (ii) dairy-drystock conversion (n=8), (iii) drystock (n=10), and (iv) lifestyle (n=11), the latter reflecting dairy and drystock conversions to lifestyle blocks. In the current study, dairy and drystock sites are defined as sites being grazed for commercial income, whereas lifestyle sites are defined as land being grazed for other purposes than commercial gain.
Sample collection and analysis
The soil sampling methodology was similar to that reported in the Land and Soil Monitoring Guidelines whereby composite soil chemistry samples of 2.5 cm diameter were collected every 2 m along a 50 m transect at a 0–10 cm soil depth. Soil physical analyses were sampled from the insertion of stainless steel rings (10 cm diameter and 7.5 cm depth) for determination of macroporosity at −5 and −10 kPa (% v/v) (volumetric percentage of pores >60 µm and >30 µm, respectively) and bulk density (g/cm3). Data presented from 1995/00 and 2009/10 sampling events were collected in accordance with Hill & Sparling (Citation2009) whereby a stainless steel ring was taken at 15, 30 and 45 m intervals along a 50 m transect for soil physical analysis. However, in the current study, five stainless steel rings were collected at 10 m intervals along the 50 m transect in order to increase the number of replicates.
All analyses were carried out at Landcare Research IANZ-accredited laboratories (Hamilton for physical analysis and Palmerston North for chemical analyses) according to the Land and Soil Monitoring Guidelines (Hill & Sparling Citation2009). There were a total of 70 composite samples for chemical analysis, 35 for both tread and control treatments. The composite samples were well mixed, air-dried and sieved (<2 mm) for Olsen P (Olsen et al. Citation1954). High temperature combustion methods were used for total carbon (TC) and total nitrogen (TN) analyses (Blakemore et al. Citation1987). There were a total of 350 intact soil cores collected for soil physical analysis, 175 for both tread and control treatments. In the laboratory, smaller stainless steel rings (5.5 cm width and 3 cm depth) were used to subsample the larger rings by pressing into the larger core using a bench-mounted drill press. The subsampling of the larger rings is to correct for any sampling error or bias between field staff and to ensure the measurement of a fully intact soil core. The smaller cores were saturated and equilibrated at both −5 and −10 kPa on ceramic tension plates to determine volumetric pore sizes >60 µm and >30 µm, respectively (macroporosity). Dry bulk densities were calculated from oven dry weights (105 °C). Chemical analyses are presented on a gravimetric basis to align with target guidelines for soil quality (Sparling et al. Citation2003).
Statistical analysis
Concentrations of Olsen P, macroporosity at −10 kPa, bulk density, TC and TN were tested for normality and log transformed if necessary before being subjected to ANOVA fitting terms for treatment, Soil Order, land use and the factorial interaction of treatment and Soil Order, and treatment and land use, all based on the 2011 sampling event data. Macroporosity −10 kPa is only described in the 2011 sampling event data when comparing against Soil Order and land use since macroporosity −5 kPa and −10 kPa trends are very similar. Blocking was used when comparing between the three sampling periods (1995/00, 2009/10 and 2011) and site number used as the blocking factor. Mean replicate data were used when comparing soil physical quality (macroprosity −5 kPa and bulk density) between the three sampling periods to correct for replicate variation, and macroporosity −5 kPa was log transformed when comparing between the sampling periods. For the 2011 sampling event only tread treatment data were used when comparing between the three sampling periods. The correlation coefficients between mean bulk density and TC or TN based on the 2011 sampling event data were determined by fitting linear regressions and the level of significance was determined using the F-statistic. Where used, the mean standard error of difference (SED) and F-statistic are presented in tabular form while specific comparisons in graphs are made with the mean least significant difference (LSD) at P=0.05. All analyses were carried out using the statistical package Genstat 14 (Citation2011) and graphical package Sigmaplot 11 (Citation2008).
Results and discussion
Changes in soil quality over time
There were significant differences (P<0.001) in soil quality for mean macroporosity (−5 kPa), bulk density and Olsen P between the three sampling events that took place in 1995/00, 2009/10 and 2011 (). Both Olsen P and bulk density increased significantly in the second sampling event and decreased in 2011. Based on log transformed data, mean macroporosity (−5 kPa) decreased significantly in the second sampling event and neither increased nor decreased in 2011 sampling. The observed changes in bulk density are likely to have implications on measured TC and TN stocks in the 0–10 cm soil depth, but since a large focus of the study was to explore the low macroporosity, TC and TN contents are presented on a gravimetric basis. There were no significant changes in TC and TN over time. Mean TC contents were 8%, 7.8% and 7.8% for sampling events 1995/00, 2009/10 and 2011, respectively, and mean TN contents were 0.66%, 0.69% and 0.68%, respectively (data not shown). Mean TC and TN were within the recommended guideline range for Soil Order and land use across all three sampling events (Sparling et al. Citation2003).
Figure 1 Changes in mean Olsen P concentrations, bulk density and macroporosity (−5 kPa), for three soil sampling periods. The boxes represent the inter-quartile range (25th to 75th percentile) and the whiskers show the range of values that fall within the inner fences. •=outliers. The median is shown as a line in each box.
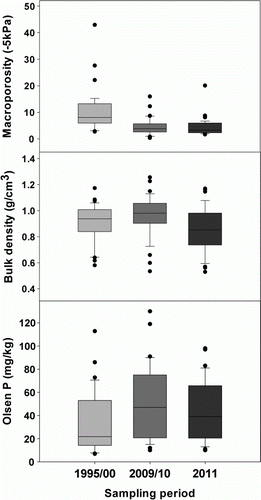
Although there were several outliers for median bulk density and Olsen P concentrations (), the majority of sites were within the recommended guideline range (Sparling et al. Citation2003; Taylor Citation2011). There was a significant decrease in macroporosity (−5 kPa). The guideline for macroporosity is 8% v/v for −5 kPa (Sparling et al. Citation2003) and 10% v/v for −10 kPa (Mackay et al. Citation2006) for pastoral land. Macroporosity (−5 kPa) in the Auckland region is a cause for concern considering that median values were just within the guideline range for soil quality of 8% v/v in the 1995/00 sampling event but had dropped by half in 2009/10 and remained low in the current study (). Soil compaction is also an issue for the Waikato region for dairy and drystock sites with macroporosities below target guidelines (Taylor et al. Citation2010). Changes in soil physical quality could be attributed to some extent to sampling time given that there is a strong annual cycle of compaction and soil recovery, with soil physical quality generally better in summer than in late winter (Curran Cournane et al. Citation2011a). Across the three soil sampling events, sampling varied at most by 2–3 months (August–October), but as climatic conditions may have significant seasonal effects on these measurements, it is not possible to rule out climatic variability. The sampling timeframe is also likely to capture a worst case scenario for soil physical quality when soil moisture is more likely to be near or close to field capacity.
Influence of treatment on soil quality
There were significant differences between tread and control treatments for macroporosity (−10 kPa) and Olsen P concentrations (). Macroporosity (−10 kPa) was significantly greater (P<0.001) and Olsen P significantly lower (P<0.001) for control treatments (). There were no significant differences for bulk density, TC and TN between treatments. Singleton et al. (Citation2000) reported mean macroporosities (−10 kPa) 14%, 10% and 10% for never trodden (NT; taken underneath fence-lines), usual (U; average pasture and paddock condition) and previously pugged (PP) treatments, respectively, at the 5–10 cm soil depth. Mean macroporosities (−5 kPa) were recorded as 11%, 7% and 8% for NT, U and PP treatments, respectively, sampled at the same soil depth and representing Allophanic, Gley, Podzol and Ultic soils (Singleton et al. Citation2000). Bearing in mind the variation in sampling depth, the findings reported by these authors (5–10 cm soil depth) are similar in range for tread and control treatments in the current trial (0–7.5 cm soil depth) although values are at the lower end of the scale for the tread treatment in the current study.
Table 2 Concentrations of Olsen P (mg/kg), total carbon (%), total nitrogen (%), bulk density (g/cm3) and macroporosity −5 and −10 kPa (% v/v) for control and tread treatments. Standard error of difference (SED) is given for comparison between treatments. ns and *** denote not significant and significant at the P<0.001 level, respectively.
Cattle & Southorn (Citation2010) also reported that macroporosities, defined as pores between 65–150 µm and >150 µm using image analysis, were greatest under pasture cages and least under set-stocked Merino sheep from a pasture trial in New South Wales, Australia. These authors found no significant differences in bulk density and carbon content between treatments over a 3-year trial, reiterating the sensitivity of macroporosity as a key soil quality indicator. In south-east Ireland, Kurz et al. (Citation2006) reported both lower macroporosity and higher bulk density in areas where cattle had free access to graze, than in areas where they could graze but could neither walk nor defecate on the plot area. In the current study, it was both physically and visually apparent that the majority of fence-lines were well established; physically given the cores were a lot easier to push into the soil, and visually given that soil surface height was higher in level under the fence-line than the surrounding grazed area at the majority of sites. Zegwaard (Citation2000) also reported that soil under a well-established fence-line was 40–80 mm higher than the surrounding grazed area as a result of treading.
Sources of phosphorus (P) in grazed pasture are soil, plant, fertilizer and faecal in origin (Nash & Halliwell Citation1999). Soil quality is likely to be at its least compacted state under and near fence-lines with cattle and sheep preferring to walk on camps and easy contoured areas (Sheath & Carlson Citation1998; Niu et al. Citation2009). This is in contrast to deer grazing where they have a tendency to pace fence-lines causing soil compaction and increased P loss (McDowell et al. Citation2004). Such camp site activity may have contributed to increased concentrations of Olsen P for the tread treatment for some sites in the current trial, particularly lifestyle and drystock, although it is less likely on dairy sites. Olsen P concentrations in grazed pasture could equally be a result of daily grazing and continuous defecation considering that cattle and sheep have been reported to defecate 11–16 and 7–26 times a day, respectively (Haynes & Williams Citation1993). Concentrations of Olsen P in grazed pasture may also be an artefact of P top-dressing, as bulk spreaders operate in paddocks and away from fence-lines.
Influence of Soil Order on soil quality
There were significant differences for combined treatments between Soil Order for all soil measurements () and significant differences for the interaction of Soil Order and treatment for macroporosity (−10 kPa), Olsen P and TC (). The Recent Soil Order had greatest macroporosity (−10 kPa) and bulk density towards the upper limit. The Recent Soil Order was represented by the Pinaki sand soil series, a young, weakly weathered sand of aeolian origin (Claridge Citation1961). Typically, high macroporosities are linked with low bulk densities and vice versa (Curran Cournane et al. Citation2010a,Citationb), albeit being subject to soil texture. However, Pinaki sands on the western coasts of Auckland contain heavy minerals, such as magnetite (Claridge Citation1961) so high bulk density does not necessarily indicate soil compaction but is a distinctive characteristic of this soil series. Given that Recent soils are young soils they have not had enough time to develop significant amounts of organic material (Claridge Citation1961) and this is reflected in their TC and TN contents ( and ).
Figure 2 Concentrations of Olsen P (mg/kg), total carbon (%) and macroporosity −10 kPa (% v/v) for the interaction of control and tread treatments with Soil Order. The least significant difference (LSD05) is given for the interaction of control and tread treatments and Soil Orders.
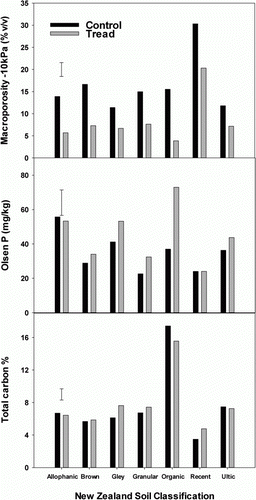
Table 3 Concentrations of Olsen P (mg/kg), total carbon (%) and total nitrogen (%), bulk density (g/cm3), macroporosity −5 and −10 kPa (% v/v) for Soil Order. Standard error of difference (SED) is given for comparison between Soil Orders for combined control and tread treatments. *** denotes significant at the P<0.001 level.
Unsurprisingly, Organic soils had greatest TC and TN contents being dominated by organic matter with characteristically low bulk densities (Molloy Citation1993; Hewitt Citation1998). Total C and TN have been reported to be positively correlated (Schipper et al. Citation2007), and similarly, correlated well in the current trial (r 2 =0.96). Furthermore, there was a significant negative correlation for bulk density and TC (a) and for bulk density and TN (b). Organic soils typically have >15% total C (Sparling et al. Citation2003) and up to 70% organic matter (Hewitt Citation1998). As a result, C content has been reported to be an inappropriate soil quality indicator for this Soil Order (Sparling et al. Citation2003). When Organic soil was excluded from the dataset, significant differences continued to be observed for TC content and Soil Order and, also for the factorial interaction of Soil Order and treatment (), so Organic soil remained in the analysis ().
Figure 3 Correlations between bulk density (g/cm3) and (A) total carbon (%) and (B) total nitrogen (%). There was no significant difference between regression lines for control and tread treatments for the correlation between bulk density and total carbon, therefore only a single line was fitted (A). There was a significant difference between regression lines for control and tread treatments for the correlation between bulk density and TN and therefore two lines were fitted (B).
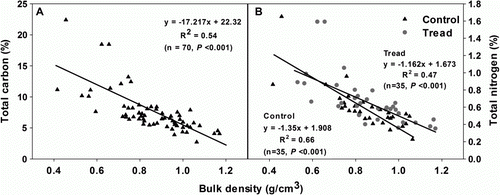
Ultic soils had second-highest TC and TN contents ( and ). This was largely associated with two Ultic soil sites being located where kikuyu pasture was dominant, a pasture species that originates in South Africa, with characteristically deep rooting systems. Milne & Haynes (Citation2004) also reported that soil organic carbon (OC) was significantly greater under kikuyu pasture than that under ryegrass and that OC was negatively correlated with bulk density.
Although the Organic soils had high TC content and low bulk density (), these soils had the greatest difference between tread and control treatments for macroporosity (). However, Ultic and Gley soils were most compact overall measured by macroporosity (−10 kPa) ( and ). These soils are typically regarded as having drainage impediments and being susceptible to treading damage (Hewitt Citation1998; Singleton et al. Citation2000; Curran Cournane et al. Citation2010a,Citationb). For the tread treatment, all Soil Orders failed to meet the macroporosity (−10 kPa) guideline except for Recent soils. For the control treatment, macroporosity (−10 kPa) varied between 11% and 30% across the seven Soil Orders (). Macroporosity was just within the target range for the Gley and Ultic soils for the control treatment (11% and 12%, respectively) and therefore unlikely to meet the recommended guidelines under livestock treading. Given that about 40% of the area in Auckland is currently mapped as Ultic soil (NZLRI Citation2010), which is also reflected in the number of soil quality sites located on these soils (), the overall issues with soil compaction in rural Auckland are largely influenced by the extent of this Soil Order. Extra care should be taken when grazing these soils by implementing practices such as restricted grazing, reduced stocking densities and/or off-site grazing facilities for periods of high soil moisture. More research is required to establish the impact of such issues both agronomically and environmentally.
There were significant differences for concentrations of Olsen P and Soil Order with concentrations being greatest for Allophanic and Organic soils (). The majority of Allophanic and Organic soils in the current study were occupied by dairy sites () and the high Olsen P concentrations observed for these soils largely reflect the intensification associated with dairying. There was also a significant difference for the factorial interaction of Soil Order and treatment (), but when Organic soils was excluded from the dataset, significance was only apparent for Soil Order and not the factorial interaction of Soil Order and treatment.
Influence of land use on soil quality
There were significant land use differences for all soil measurements for combined tread and control treatments () and significant differences for the interaction of land use and treatment for macroporosity (−10 kPa) and Olsen P (). For combined treatments, macroporosity (−10 kPa) was greater for drystock sites and lower for dairy, dairy-drystock and lifestyle sites. There was very little difference between dairy-drystock conversion and lifestyle sites with regard to bulk density, but soil under dairy pasture had clearly higher bulk density (). Macroporosity (−10 kPa) was also within the recommended guideline range (10%–30% v/v) for soil health for the combination of treatments (Mackay et al. Citation2006; Hill & Sparling Citation2009). However, for the tread treatment, compaction measured by macroporosity −10 kPa was below recommended guidelines for all but drystock sites, and was most apparent for dairy sites followed by lifestyle and dairy-drystock conversion sites (). In New Zealand there has been pronounced change in land use with the conversion of many sheep farms to dairy farms over the past 20 years (Monaghan et al. Citation2005). However, the picture is quite different within the Auckland region. The State of the Auckland Region (Auckland Regional Council Citation2010) reported that dairy cattle numbers declined by 23%, dairy farms by 33% and dairy farmed area (ha) by 25% between 2002 and 2008, while beef cattle and sheep numbers declined by 16% and 28%, respectively. Despite the average dairy herd size increasing by 21% between 2002 and 2008 in the Auckland region (Auckland Regional Council Citation2010), average herd sizes remain small by New Zealand standards (LIC Citation2011). In the current study, the degree of intensification associated with dairying was apparent in the low macroporosity and the high Olsen P concentrations for dairy sites ( and ). Although Olsen P concentrations were least for lifestyle sites, reflecting their lesser intensive land use, these sites were the second most compacted land use in terms of macroporosity. It will be important to determine if improved macroporosity is detected for these lesser intensive sites at later sampling events. Significant differences for the interaction of land use and treatment effect for Olsen P concentrations were only apparent for dairy and dairy-drystock sites ().
Figure 4 Concentrations of Olsen P (mg/kg) and macroporosity −10 kPa (% v/v) for control and tread treatments for land use types. The least significant difference (LSD05) is given for the interaction of control and tread treatments and land use types.
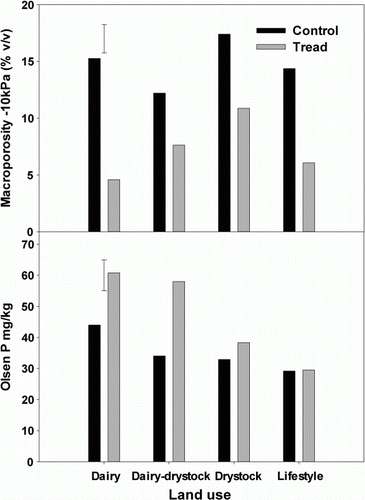
Table 4 Concentrations of Olsen P (mg/kg), total carbon (%) and total nitrogen (%), bulk density (g/cm3) and macroporosity −5 and −10 kPa (% v/v) for land use for combined control and tread treatments. Standard error of difference (SED) is given for comparison between treatments. * and *** denote significance at the P<0.05 and P<0.001 levels, respectively.
Total C and TN contents were greatest for lifestyle>dairy>dairy-drystock>drystock sites (). Lifestyle blocks were the largest land use category in the current study representing 11 sites (31%). Andrew & Dymond (Citation2012) reported that in Auckland 21% of lifestyle blocks are on high-class land which amounts to 35% of all high-class land in the region. Currently, lifestyle blocks cover about 10% of the Auckland region and represent 64% of land parcels (AssureQuality Citation2012). In comparison, drystock and dairy farms cover 49% and 14% of the Auckland region, respectively, and account for 17% and 2%, respectively, of land parcels (AssureQuality Citation2012). Although 83% of dairy sites in the current study occupied elite or prime land (defined as LUC classes 1–3 in the Auckland Regional Policy Statement [Auckland Regional Council Citation2008]), a large proportion of lifestyle sites (64%) were also on high-class land followed by dairy-drystock (38%) and drystock (30%). Such information raises two concerns: first, that a large proportion of elite or prime land in the current study is not solely being used for commercial primary production; and second, the difficulty in developing soil quality trends for dairy and drystock sites over time as a result of the extent of land use change that is being experienced within the Auckland region. The latter should not deter continuing soil quality monitoring across the Auckland region because landowners were advised of their soil quality results, either in person or by letter, and best management practices were recommended, with particular attention to macroporosity. It will be important to determine if a response is detected in future reporting.
Conclusions
There were significant differences in mean changes for macroporosity (−5 kPa), bulk density and Olsen P across sampling events 1995/00, 2009/10 and 2011 for grazed pasture. Bulk density and Olsen P increased in the second sampling event, then reverted close to original values in 2011, whereas macroporosity decreased in the second sampling event and remained low in 2011. Olsen P and bulk density across grazed sites were within the recommended guidelines for soil health but macroporosity was below the recommended guideline, indicating soil compaction. When tread and control treatments were compared, significant differences were observed for macroporosity (−10 kPa) and Olsen P with the former being less and the latter being greater for the tread treatment. Soil Order had a significant effect on all soil measurements. Significant differences for the interaction of Soil Order and treatment were observed for macroporosity (−10 kPa), Olsen P and TC. For the tread treatment, all Soil Orders failed to meet the macroporosity (−10 kPa) guideline except for Recent soils. For combined treatments, macroporosity was least for Ultic and Gley soils. For the control treatment macroporosity was just within the target range for Ultic and Gley soils. Ultic soils represent about 40% of the soils within rural Auckland and land management issues with low macroporosity are largely influenced by this Soil Order.
The degree of land use change was also very apparent in the study. What were originally described as two land use categories across the 35 sites (dairy, n=19; and drystock, n=16) increased to four land use categories: dairy (n=6), dairy-drystock (n=8), drystock (n=10) and lifestyle sites (n=11), of which five were dairy-lifestyle and six drystock-lifestyle conversions for the latter. Consequently, land use had a significant impact on all soil measurements. Macroporosity was least and Olsen P greatest for grazed dairy sites reflecting the intensification associated with this land use. Lifestyle sites had greatest TC and TN contents followed by dairy>dairy-drystock>drystock sites. Dairy, lifestyle, dairy-drystock and drystock sites occupied 83%, 64%, 38% and 30%, respectively, of what is considered to be elite or prime land which raises two concerns: first, that a large proportion of elite or prime land in the current study is not solely being used for commercial primary production; and second, the difficulty in developing soil quality trends for dairy and drystock sites over time given the rapid land use change within the Auckland region.
Acknowledgements
The authors would like to thank all the landowners for giving permission to undertake soil sampling on their properties and for providing site information. The Environmental Monitoring and Land & Management teams at Auckland Council are thanked for assisting with field work. John Claydon from Landcare Research is thanked for providing expert laboratory advice. The authors would like to thank the anonymous reviewers of this paper which has improved its quality.
References
- Andrew R , Dymond J 2012 . Expansion of lifestyle blocks and urban areas onto high-class land . Journal of the Royal Society of New Zealand . doi: 10.1080/03036758.2012.736392
- AssureQuality 2012 . Agribase Database Version 2012 . Wellington , Assure Quality .
- Auckland Regional Council 2008 . Auckland regional policy statement: Soil conservation . Auckland , Auckland Regional Council . Chapter 12 . 240
- Auckland Regional Council 2010 . State of the Auckland region . Auckland , Auckland Regional Council . 300
- Betteridge , K , Mackay , AD , Shepherd , TG , Barker , DJ , Budding , PJ , Devantier , BP and Costall , DA . 1999 . Effect of cattle and sheep treading on surface configuration of a sedimentary hill soil . Australian Journal of Soil Research , 39 : 743 – 760 .
- Bilotta , GS , Brazier , RE and Haygarth , PM . 2007 . The impacts of grazing animals on the quality of soils, vegetation, and surface waters in intensively managed grasslands . Advances in Agronomy , 94 : 237 – 280 .
- Blakemore , LC , Searle , PL and Daly , BK . 1987 . Methods for chemical analysis of soils. NZ Soil Bureau Scientific Report 80 , Lower Hutt , Wellington : Department of Scientific and Industrial Research Soil Bureau .
- Cattle , SR and Southorn , NJ . 2010 . Macroporosity of pasture topsoils after three years of set-stocked and rotational grazing . Australian Journal of Soil Research , 48 : 43 – 57 . doi: 10.1071/SR09004
- Claridge , GGC . 1961 . Mineralogy and origin of the yellow-brown sands and related soils . New Zealand Journal of Geology and Geophysics , 4 : 48 – 72 . doi: 10.1080/00288306.1961.10420138
- Climo , WJ and Richardson , MA . 1984 . Factors affecting the susceptibility of 3 soils in the Manawatu to stock treading . New Zealand Journal of Agricultural Research , 27 : 247 – 253 . doi: 10.1080/00288233.1984.10430426
- Curran-Cournane F 2010 . Impacts of livestock grazing on soil physical quality and phosphorus and suspended sediment losses in surface runoff. PhD thesis. Canterbury , Faculty of Agriculture and Life Science, Lincoln University .
- Curran Cournane , F , McDowell , RW and Condron , LM . 2010a . Do aggregation, treading and dung deposition affect phosphorus and suspended sediment losses in surface runoff? . Australian Journal of Soil Research , 48 : 705 – 712 . doi: 10.1071/SR10043
- Curran Cournane , F , McDowell , RW and Condron , LM . 2010b . Effects of cattle treading and soil moisture on phosphorus and sediment losses in surface runoff from pasture . New Zealand Journal of Agricultural Research , 53 : 365 – 376 . doi: 10.1080/00288233.2010.509903
- Curran Cournane , F , McDowell , RW , Littlejohn , RP and Condron , LM . 2011a . Effects of cattle, sheep and deer grazing on soil physical quality and phosphorus and suspended sediment losses in surface runoff. Agriculture . Ecosystems & Environment , 140 : 264 – 272 . doi: 10.1016/j.agee.2010.12.013
- Curran Cournane F , McDowell RW , Littlejohn RP , Houlbrooke DJ , Condron LM 2011b . Is mechanical soil aeration a strategy to alleviate soil compaction and decrease phosphorus and suspended sediment losses from irrigated and rain-fed cattle-grazed pastures? Soil Use and Management . doi: 10.1111/j.1475-2743.2011.00345.x
- Drewry , J , Littlejohn , R , Paton , R , Singleton , P , Monaghan , R and Smith , L . 2004 . Dairy pasture responses to soil physical properties . Australian Journal of Soil Research , 42 : 99 – 105 . doi: 10.1071/SR03055
- Drewry , JJ . 2003 . Dairy grazing strategies to minimise soil pugging and compaction in the Waikato . Proceedings of the New Zealand Grassland Association , 65 : 99 – 103 .
- Drewry , JJ , Cameron , KC and Buchan , GD . 2008 . Pasture yield and soil physical property responses to soil compaction from treading and grazing: A review . Australian Journal of Soil Research , 46 : 237 – 256 . doi: 10.1071/SR07125
- Drewry , JJ , Cameron , KC and Buchan , KC . 2001 . Effect of simulated dairy cow treading on soil physical properties and ryegrass pasture yield . New Zealand Journal of Agricultural Research , 44 : 181 – 190 . doi: 10.1080/00288233.2001.9513476
- Drewry , JJ , Littlejohn , RP and Paton , RJ . 2000 . A survey of soil physical properties on sheep and dairy farms in southern New Zealand . New Zealand Journal of Agricultural Research , 43 : 251 – 258 . doi: 10.1080/00288233.2000.9513425
- Edmond DB . 1966 . The influence of animal treading on pasture growth . In : Proceedings of the X International Grassland Congress 7–16 July . Finland , University of Helsinki . 453 – 458 .
- Fleming P 2003 . Farm technical manual, Lincoln University . Christchurch , , New Zealand , The Caxton Press .
- Fraser S , Stevenson B 2011 . Soil quality of drystock sites in the Auckland Region 2010 . Prepared by Landcare Research for Auckland Regional Council. Auckland Regional Council Technical Report 2011/011 .
- GenStat 2011 . Genstat for Windows , 14th edition . Oxford , , UK , VSN International .
- Haynes , RJ and Williams , PH . 1993 . Nutrient cycling and soil fertility in the grazed pasture ecosystem . Advances in Agronomy , 49 : 119 – 199 .
- Hewitt AE 1998 . New Zealand soil classification . Lincoln , , New Zealand , Manaaki Whenua Press .
- Hicks DL 1995 . Ranking of Auckland soils' susceptibility to degradation . Prepared for Auckland Regional Council. Auckland Regional Council Technical Report TR 2009/044 .
- Hill RB , Sparling GP 2009 . Soil quality monitoring. In: Land monitoring forum. Land and soil monitoring: a guide for SoE and regional council reporting . Hamilton , Land Monitoring Forum . 27 – 88 .
- Hill , RB , Sparling , GP , Frampton , C and Cuff , J . 2003 . National soil quality review and program design. Ministry for the Environment Technical Report 74 , Wellington : Ministry for the Environment .
- Houlbrooke , DJ , Paton , RJ , Morton , JD and Littlejohn , RP . 2009 . Soil quality and plant yield response under dryland and irrigated winter forage crops by sheep or cattle . Australian Journal of Soil Research , 47 : 470 – 477 . doi: 10.1071/SR08228
- Houlbrooke , DJ , Paton , RJ , Littlejohn , RP and Morton , JD . 2011 . Land-use intensification in New Zealand: effects on soil properties and pasture production . Journal of Agricultural Science , 149 : 337 – 349 . doi: 10.1017/S0021859610000821
- Kumbasli , M , Makineci , E and Cakir , M . 2010 . Long term effects of red deer (Cervus elaphus) grazing on soil in a breeding area . Journal of Environmental Biology , 31 : 185 – 188 .
- Kurz , I , O'Reilly , CD and Tunney , H . 2006 . Impact of cattle on soil physical properties and nutrient concentrations in overland flow from pasture in Ireland . Agriculture, Ecosystems & Environment , 113 : 378 – 390 . doi: 10.1016/j.agee.2005.10.004
- LIC 2011 . Livestock Improvement Corporation http://www.lic.co.nz/lic_News_Archive.cfm?nid=371 (accessed 15 May 2012) .
- Lilburne LR , Webb TH , Hewitt AE , Lynn IH , de Pauw B 2011 . S-map database manual v.1.2 , October 2011. Landcare Research Contract Report LC 478. Christchurch , , New Zealand , Landcare Research .
- Lynn , I , Manderson , A , Page , M , Harmsworth , G , Eyles , G , Douglas , G , Mackay , A and Newsome , P . 2009 . Land use capability survey handbook: A New Zealand handbook for the classification of land , 3rd edition , Hamilton : AgResearch . Lincoln, Landcare Research New Zealand; Lower Hutt, Institute of Geological and Nuclear Sciences Ltd
- Mackay A , Simcock R , Sparling G , Vogler I , Francis G 2006 . Macroporosity. Internal SLURI report . Hamilton , AgResearch .
- McDowell , RW , Drewry , JJ and Paton , RJ . 2004 . Effects of deer grazing and fence-line pacing on water and soil quality . Soil Use and Management , 20 : 302 – 307 . doi: 10.1079/SUM2004261
- McDowell , RW , Drewry , JJ , Paton , RJ , Carey , PL , Monaghan , RM and Condron , LM . 2003 . Influence of soil treading on sediment and phosphorus losses in overland flow . Australian Journal of Soil Research , 41 : 949 – 961 . doi: 10.1071/SR02118
- McDowell RW , Houlbrooke DJ , Muirhead RW , Muller K , Sheperd M , Cuttle SP 2008 . Grazed pastures and surface water quality . New York , Nova Science Publishers .
- Milne , R and Haynes , R . 2004 . Comparative effects of annual and permanent dairy pastures on soil physical properties in the Tsitsikamma region of South Africa . Soil Use and Management , 20 : 81 – 88 . doi: 10.1079/SUM2003227
- Molloy L . 1993 . Soils in the New Zealand landscapes: The living mantle, New Zealand Society of Soil Science . Canterbury , Lincoln University, New Zealand Society of Soil Science .
- Monaghan , RM , Paton , RJ , Smith , LC , Drewry , JJ and Littlejohn , RP . 2005 . The impacts of nitrogen fertilizer and increased stocking rate on pasture yield, soil physical condition, and nutrient losses in drainage from a cattle-grazed pasture . New Zealand Journal of Agricultural Research , 48 : 227 – 240 . doi: 10.1080/00288233.2005.9513652
- Nash , DM and Halliwell , DJ . 1999 . Fertilizers and phosphorus loss from productive grazing systems . Australian Journal of Soil Research , 37 : 403 – 429 . doi: 10.1071/S98087
- Niu , Y , Guangdi , L , Lingling , L , Yin Chan , K and Oates , A . 2009 . Sheep camping influences soil properties and pasture production in an acidic soil of New South Wales, Australia . Canadian Journal of Soil Science , 89 : 235 – 244 . doi: 10.4141/CJSS08004
- NZLRI 2010 . New Zealand Land Resource Inventory . Landcare Research http://lris.scinfo.org.nz/#/layer/79-fsl-new-zealand-soil-classification/ (accessed 7 March 2012) .
- Olsen SR , Cole CV , Watanabe FS , Dean LA 1954 . Estimation of available phosphorus in soils by extraction with sodium bicarbonate . United States Department of Agriculture Circular No. 939 . Washington DC US .
- Savadogo , P , Sawadogo , L and Tiveau , D . 2007 . Effects of grazing intensity and prescribed fire on soil physical and hydrological properties and pasture yield in the savanna woodlands of Burkina Faso. Agriculture . Ecosystems & Environment , 118 : 80 – 92 . doi: 10.1016/j.agee.2006.05.002
- Schipper , LA , Baisden , TW , Parfitt , RL , Ross , C , Claydon , JJ and Arnold , G . 2007 . Large losses of soil C and N from soil profiles under pasture in New Zealand during the past 20 years . Global Change Biology , 13 : 1138 – 1144 . doi: 10.1111/j.1365-2486.2007.01366.x
- Sheath , GW and Carlson , WT . 1998 . Impact of cattle treading on hill land 1. Soil damage patterns and pasture status . New Zealand Journal of Agricultural Research , 41 : 271 – 278 . doi: 10.1080/00288233.1998.9513311
- SigmaPlot 2008 . Version 11.0 for Windows . Chicago , Systat Software .
- Singleton , PL and Addison , B . 1999 . Effects of cattle treading on physical properties of three soils used for dairy farming in the Waikato, North Island, New Zealand . Australian Journal of Soil Research , 37 : 891 – 902 . doi: 10.1071/SR98101
- Singleton , PL , Boyes , M and Addison , B . 2000 . Effect of treading by dairy cattle on topsoil physical conditions for six contrasting soil types in Waikato and Northland, New Zealand, with implications for monitoring . New Zealand Journal of Agricultural Research , 43 : 559 – 567 . doi: 10.1080/00288233.2000.9513453
- Sparling G 2009 . An overview of soil quality in the Auckland region using data from the 500 Soils Project 1995–2001 . Prepared by Landcare Research for Auckland Regional Council. Auckland Regional Council Technical Report 2009/007 .
- Sparling G , Lilburne L , Vojvodic-Vucovic M 2003 . Provisional targets for soil quality indicators in New Zealand . Landcare Research Science Series No. 34 . Lincoln, Canterbury , , New Zealand , Manaaki Whenue Press .
- Stevenson B 2010 . Soil quality of dairy sites in the Auckland Region 2010 . Prepared by Landcare Research for Auckland Regional Council. Auckland Regional Council Technical Report 2010/026 .
- Taylor , MD , Kim , ND , Hill , RB and Chapman , R . 2010 . A review of soil quality indicators and five key issues after 12 yr soil quality monitoring in the Waikato Region . Soil Use and Management , 26 : 212 – 224 . doi: 10.1111/j.1475-2743.2010.00276.x
- Taylor MD 2011 . Towards developing targets for soil quality indicators in New Zealand: Findings of a review of soil quality indicators workshop , 6 May 2011 . Unpublished report. Hamilton, New Zealand Land Monitoring Forum.
- Taylor NH 1954 . General survey of the soils of the North Island, New Zealand . Soil Bureau. Bulletin (n.s.) 5 . Wellington , New Zealand Department of Scientific and Industrial Research .
- Zegwaard KE 2000 . The effect of stock trampling and pugging on selected soil physical properties . MSc (technology) thesis. Hamilton , , New Zealand , University of Waikato .
- Zegwaard KE , Balks MR , Singleton PL , Thom ER , Chapman R 2000 . Effect of pugging and treading duration on selected soil physical properties and pasture production—preliminary results . Soil 2000: New horizons for a new century. In: Australian and New Zealand Second Joint Soils Conference, 3–8 December 2000 . Lincoln , , New Zealand , Lincoln University .