ABSTRACT
The objectives of this research were to investigate Lolium perenne L. responses to water deficit, compare drought tolerance of two cultivars derived from different germplasm and the role of endophyte in drought tolerance of the host cultivar. Plants of cultivars Ceres One50 and Grasslands Commando with and without AR37 endophyte were kept irrigated or unirrigated for two weeks and then rehydrated for one month in a glasshouse. A positive correlation between the extent of osmotic adjustment (OA) and leaf dry matter regrowth indicated that OA was correlated with the plant regrowth during recovery. For One50, the reduction in leaf elongation rate was less and the increase in proline was greater in response to drought, compared to Commando. From this observation, it was inferred One50 has enhanced capacity to continue growth, and had greater intra-cellular protection than Commando. Endophyte status did not affect plant responses to drought in this experiment.
Introduction
Perennial ryegrass is widely used in New Zealand, but can suffer from poor production during short-term drought in summer. Current New Zealand ryegrass populations were mainly developed from the ‘Hawkes Bay’ and ‘Mangere’ ecotypes and introduced Spanish germplasm. The ‘Mangere’ ecotype formed the basis of many cultivars well adapted to the North Island in over several decades from the 1960s. Grasslands Nui was the first perennial ryegrass cultivar derived from this ‘Mangere’ ecotype (Armstrong Citation1977). Grasslands Commando has a close genetic affinity to Grasslands Nui (Wang et al. Citation2014). In the 1980s, germplasm from mild oceanic regions of North West Spain was introduced to New Zealand and provided valuable improvements in a number of traits including winter activity, late flowering, low vernalisation response and excellent resistance to crown and stem rust (Stewart Citation2006). In the 1990s, Grasslands Impact was bred from the Spanish germplasm and Grasslands Nui. Later, more cultivars including Tolosa, Arrow and the tetraploid Banquet were derived from Grasslands Impact. The development of other cultivars such as Trojan and Ceres One50 also incorporated Spanish germplasm. National trials evaluating perennial ryegrass cultivars showed that cultivars released after 1991 yielded 6% average more herbage annually and 9% more herbage in summer than cultivars released before 1991, and this gain was attributed mainly to the introduction of Spanish germplasm (Easton et al. Citation2001).
Perennial ryegrass with desirable characteristics of easy establishment and management, high palatability and digestibility and good persistence has been the most commonly sown grass species in New Zealand farming systems, especially in the North Island where 74% of the New Zealand’s dairy herds are located (Livestock Improvement Corporation Ltd. and DairyNZ Ltd. Citation2015). However, summer drought has increasingly restricted perennial ryegrass growth and development, in recent years; severe summer drought occurred twice in Waikato and Manawatu regions (the two largest farming regions in the North Island) within 10 years from 2004 to 2013 (He Citation2016). Such events cause significant reduction in pasture productivity and economic loss of New Zealand. Moreover, it has been predicted that New Zealand is going to experience more frequent and severe drought by the mid of this century, especially in the North Island and east areas of South Island (NIWA Citation2013); therefore, it is increasingly important to understand how perennial ryegrass cope with drought and to improve drought tolerance of perennial ryegrass. Previously, research has been conducted to investigate drought tolerance mechanisms of ryegrass (for example, Thomas & James Citation1999; Jiang & Huang Citation2001; Chai et al. Citation2010; Hatier et al. Citation2014; Jonaviciene et al. Citation2014). Some historical experiments were conducted in New Zealand using a rainout shelter to investigate grazing management effects on ryegrass drought tolerance (Barker et al. Citation1985a), and water extraction profiles for contrasting wet and dry soil moisture regimes (Barker et al. Citation1985b). However, comparative information on drought tolerance of New Zealand perennial ryegrass cultivars has been very limited.
A series of plant physiological traits have often been determined to investigate drought tolerance. For examples, malondialdehyde (MDA), one of the end products of lipid peroxidation, is commonly measured as an indicator of oxidative damage to cells and tissues (Fu & Huang Citation2001). The accumulation of free proline, an amino acid, is also often determined in stress experiments. However, the exact role of proline accumulation remains a point on which opinions expressed in the literature vary. It has been suggested that proline accumulation is merely a passive reaction to severity of stress experienced by the plant (Bokhari & Trent Citation1985). However, others see proline as a protectant: proline accumulation is associated with prevention of protein denaturation, preservation of enzyme structure and activity (Samuel et al. Citation2000) and protection of membranes from damage by reactive oxygen species (ROS) produced under stress (Hamilton & Heckathorn Citation2001). Meanwhile, the role of proline accumulation in osmotic adjustment (OA) is now commonly considered less important than other roles (Meloni et al. Citation2001; Gomes et al. Citation2010).
Another factor to be considered in drought tolerance research is the presence or absence of fungal endophyte in the material to be tested. Epichloë endophytes were present in perennial ryegrass seeds from Europe in the early nineteenth century, and are now almost universally incorporated in commercial ryegrass cultivars to assist control of insect predation (Johnson et al. Citation2013). It has also been reported that Epichloë endophyte presence improved drought tolerance of some forage grass species such as tall fescue (Elmi & West Citation1995; Nagabhyru et al. Citation2013), perennial ryegrass (Amalric et al. Citation1999; Kane Citation2011) and meadow fescue (Malinowski et al. Citation1997). To overcome the animal disorders caused by alkaloid secondary metabolites of fungal endophyte in forage grasses, endophyte strains, particularly in perennial ryegrass, that produce no toxic alkaloids or low levels of the alkaloids, have been identified and selected in New Zealand (Johnson et al. Citation2013). Those selected endophyte strains have been licensed with ryegrass cultivars in the seed market, including AR1, Endo5, NEA2 and AR37 (Johnson et al. Citation2013). However, a majority of the published research has been conducted with the so-called ‘wild type’ endophyte which can cause animal disorders, and much less scientific information is available to define whether the commercial endophyte strains play a role in enhancing drought tolerance of perennial ryegrass host cultivars.
Based on these considerations, the objectives of this research were: (1) to investigate perennial ryegrass responses to water deficit; (2) to compare drought tolerance of two perennial ryegrass cultivars with and without Spanish germplasm in their breeding pedigree; (3) to investigate whether the commercial Epichloë endophytes have an effect on drought tolerance of the host cultivar.
Materials and methods
Plant material
Seeds of cultivar Ceres One50 (One50) and Grasslands Commando (Commando) infected with AR37 endophyte (E+) or free of endophyte infection (E–) were obtained from PGG Wrightson Seeds Ltd. Seeds were placed in an incubator in a temperature regime of 30°C/20°C light/dark and 40% humidity from 18 November 2013. One week later, seedlings of similar size were transplanted to plastic pots containing potting mix and slow release fertiliser. Seedlings were kept in a glasshouse under natural light and well irrigated for one and half months. Endophyte status was checked using immunoblotting (Simpson et al. Citation2012).
Experiment design
On 17 January 2014, 10 individual plants with the required endophyte status were selected to represent each cultivar-endophyte association, and each plant was split into six clones. Each clone, consisting of four adult tillers, was transplanted into a PVC pipe (height 50 cm, diameter 10 cm). From the bottom to top, the pipe was filled with 15 cm of sand (dry bulk density 1.42 g/ml), 10 cm of a mixture (50%, 50%) of air-dried Manawatu silt loam (dry bulk density 1.25 g/ml) and sand, and 20 cm of air-dried Egmont soil A horizon (dry bulk density 0.74 g/ml). Two layers of fine wind netting nylon mesh were taped on the end to hold soil in the pipe. After plants were transplanted into pipes, a PVC ring (height 1.5 cm, diameter 3.5 cm) cut from a pipe was fully inserted into the soil surrounding each plant to avoid plant horizontal expansion and to achieve similar plant size before drought treatment was initiated. Every pot was weighed after the air-dried soil was filled in and weighed again after being fully watered (24 hours later) to estimate the soil water holding capacity of the pot (931 ml on average).
Plants in their pipes were arranged in groups in a split-plot experimental design, with irrigation treatment (I+, irrigation and I–, non-irrigation) as the main-plot factor. Ten individual plants (ten genotypes) of each cultivar-endophyte association were randomly arranged in each group. Groups of pipes were considered equivalent to plots in a field experiment, to allow for possible temperature or light intensity gradients within the glasshouse. Plants were fully irrigated on the first day after being transplanted into the pipes, and 150 ml water was supplied daily to each plant over the following two weeks. Thereafter, 300 ml water was supplied to each plant daily. On 17 March 2014, plants that had not fully colonised the PVC ring were discarded, leaving eight genotypes of each cultivar-endophyte association in the experiment. The total number of plants finally used in the experiment was therefore 192 (2 cultivars × 8 genotypes × 2 endophyte conditions × 2 irrigation treatments × 3 replicates). The six clonal replicates of each genotype allowed for I+ and I– treatments to be replicated three times for each genotype in the split-plot stratum of the experiment. Leaf water potential (LWP) and gas exchange parameters of a certain number of plants were determined one week before drought treatment began, in order to confirm there was no difference between plants in I+ and I– plots at this stage. From 3 April 2014, irrigation was withheld for two weeks for plants in the I– plots and followed with one month’s rehydration, while plants in I+ plots were consistently well irrigated. All plants were in vegetative growth in this experiment as they were not vernalised.
During the months of the experimental period from January to May inclusive, the monthly means for daily maximum temperature in the glasshouse were 28.6°C ± 0.5°C, 29.6°C ± 0.6°C, 26.4°C ± 0.5°C, 23.8°C ± 0.6°C, 21.1°C ± 0.2°C, respectively, and the average minimum daily temperature were 16.6°C ± 0.5°C, 17.6°C ± 0.3°C, 15.0°C ± 0.4°C, 14.6°C ± 0.4°C, 11.2°C ± 0.6°C, respectively.
Measurements
Measurements including leaf elongation rate (LER), leaf senescence rate (LSR), plant water relations, gas exchange parameters, electrolyte leakage (EL), and plant sample collection for proline and MDA concentration of foliar material were conducted during the drought period. On 18 April 2014, half of the plants (four genotypes of each cultivar-endophyte association from each plot, in total 96 plants) were clipped to 7.5 cm above soil surface to determine leaf dry matter, and then plants were transferred to a 4°C walk-in refrigerator for storage while awaiting further sampling for stubble dry matter, root organic matter and gravimetric soil water content. The remaining half of the plants was rehydrated for one month, after which the regrowth leaf dry matter was determined at the end of the rehydration period.
Plant water relations, gas exchange parameters, EL, proline concentration and MDA concentration were all determined in the first fully expanded leaves of a/several randomly selected adult tiller/s of each plant.
Soil water content
Approximately 300 g soil samples from each soil layer were recorded before and after oven-drying at 105°C for 24 hours. The SWC (%, w/w) was calculated as: SWC (%, w/w) = (FW – DW)/DW × 100%, where FW and DW refer to fresh weight and dry weight of the soil samples, respectively.
Plant growth parameters
Three adult tillers of each plant were randomly selected and labelled with plastic tapes with different colours. Initial leaf length (from leaf ligule to tip, or to the boundary of the chlorotic portion if senescence had begun) of each leaf on each labelled tiller was recorded on 7–8 April (L1) and again 15–16 April (L2). The LER and LSR of each plant were calculated as:
Leaf DM and the stubble DM (mainly pseudostem) were harvested and oven-dried at 80°C for 48 hours. The sum of the leaf DM and stubble DM was considered to be the total shoot DM.
For the 96 plants in the storage room as mentioned above, all visible roots were picked out of the soil, washed and oven-dried. The root organic matter (root OM) was determined by combusting samples in a porcelain mortar in a muffle furnace at 650°C for two hours (Schuurman & Goedewaagen Citation1965). Root OM was calculated as the difference between weight (mortar, oven-dried root and residual soil) before and after combustion. The root OM was recorded instead of the root DM because soil particles could not be washed off completely from the roots, which would contribute errors to the determination of root dry matter. The root:shoot ratio was calculated as: root:shoot ratio = root OM/shoot DM.
Plant water relations
The relative water content (RWC), LWP and osmotic potential (OP) were measured from 12 April to 14 April, one replication per day. The RWC was determined as: RWC (%) = [(FW−DW)/(TW−DW)] × 100%, where FW is the leaf fresh weight, DW is the dry weight of leaves after drying 80°C for 48 hours, and TW is the turgid weight of leaves after soaking in water overnight in darkness at room temperature. The predawn LWP was measured between 05:00 h and 08:00 h using a Scholander pressure chamber (Soil Moisture Equipment Crop., Santa Barbara. CA) (Scholander et al. Citation1965). After taking leaf samples to determine LWP, the lower 2 cm of the same leaves were cut immediately as samples for the OP determination. The OP was measured using a number of Wescor C-52 sample chambers (Wescor, Logan, UT, USA) by the dew-point method (Turner Citation1981), in conjunction with the Wescor HR-33 T microvolt metre. In order to convert the µV values to MPa, a standard curve was prepared for each of the chambers by measuring a series of NaCl solutions with different molalities (0. 0.2, 0.4, 0.6, 0.8, 1.0 m) according to the Wescor C-52 manual. Turgor pressure (TP) was calculated as: TP = LWP – OP.
Osmotic adjustment
On 18 April, the 96 un-harvested plants were fully irrigated in the evening and the OP of these plants was determined the next morning (predawn). OA was calculated as the difference in OP between rehydrated I– plants (OP1) and the consistently I+ counterparts (OP0): OA = OP1 – OP0 (Begg Citation1980; Blum Citation1989).
Gas exchange parameters
It was possible to measure the gas exchange parameters of a maximum of 12 plants in a day between 09:00 h and 11:00 h, after which time gas exchange might have been curtailed by falling LWP and stomatal closure. Therefore, only three genotypes of cultivar One50 with and without endophyte in each plot were measured from 12 to 14 April, one replication per day.
The rate of photosynthesis (Pn, µmol CO2 m−2 leaf s−1), transpiration rate (Tr, mmol H2O m−2 leaf s−1) and stomatal conductance (Gs, mol H2O m−2 leaf s−1) were measured for each entry, using a portable photosynthesis system (Li6400, LiCor Inc., USA) fitted with a standard 20 mm × 30 mm leaf chamber, leaf thermocouple and a blue-red LED light source. The photosynthetically active radiation was 1500 µmol m−2 s−1, ambient CO2 concentration was 390 µmol CO2 mol−1 air, temperature of the leaf chamber was 20°C, and the relative humidity in the chamber was controlled within a range of 50–75%.
Proline and MDA concentration
On 17 April 2014, leaf samples for free proline and MDA analysis were cut and placed in a plastic-sealed bag and immersed in liquid nitrogen immediately. Samples were then stored in a –80°C freezer. Leaf samples were freeze-dried then ground to powder using a Retsch MM200 ball mill.
To determine leaf proline concentrations, about 40 mg of leaf powder was weighed into a centrifuge tube and a free proline concentration analysis conducted using the proline assay kit for plant tissue (Product No. A107, Nanjing Jiancheng Bioengineering Institute Ltd., China). Absorbance was read at 518 nm using a spectrophotometer (Bausch & Lomb Spectronic 20).
A further sample of about 40 mg leaf powder was analysed for MDA using an MDA assay kit (Product No. A003-3, Nanjing Jiancheng Bioengineering Institute Ltd., China). Absorbance was read at 532 nm and 600 nm (Biochrom Libra S60PC Double Beam Spectrophotometer, Cambridge, England). The absorbance for the nonspecific turbidity at 600 nm was subtracted from the reading at 532 nm.
Electrolyte leakage
Cell membrane EL was determined from 12 to 14 April 2014. Leaf samples were collected and placed in a self-seal plastic bag on ice, then immediately transferred to the lab after sample collection was complete. Leaf samples were washed with running reverse osmosis water followed with deionised water to remove any electrolytes adhering to the leaf surface, and then cut into 1 cm long segments and put in a test tube containing 15 ml of deionised water. Tubes were shaken for 30 minutes at room temperature to allow electrolyte diffusion from the leaf tissue. The initial conductance (EC1) was determined with an electric conductivity meter. Tubes were put in the autoclave at 120°C for 30 minutes to lyse cell membranes and completely release electrolytes, then brought to room temperature, shaken for 24 hours and conductance was measured again (EC2). The percentage of EL were calculated as: EL (%) = EC1/EC2 × 100% (Blum & Ebercon Citation1981; Bayat et al. Citation2009).
Statistical analysis
A general linear model procedure (Proc GLM) using SAS software (version 9.4, SAS institute, Cary, NC, USA) was employed for the majority of variables. The SAS code used to define the statistical model was: variable = block block*irrigation irrigation|cultivar|endophyte. Then A TEST option was included in the model to determine the irrigation effect using the ‘irrigation × block’ interaction as the error term. Irrigation treatment or endophyte effects on each cultivar were evaluated using a SLICE function, that is, Cultivar × irrigation (or endophyte)/slice = cultivar pdiff, which requests a P value to detect the effect of irrigation or endophyte on each cultivar. The residuals from the GLM procedure for RWC, TP and proline concentration were not normally distributed even after data transformation, so a non-parametric factorial analysis was applied. The data were aligned rank transformed (ART) using the ARTool package in R, to generate normally distributed ranks, then subjected to the ANOVA test (Wobbrock et al. Citation2011).
Results
Before the drought treatment commenced, the average LWPs of plants in I+ pots and I– pots were –0.20 MPa and –0.16 MPa, respectively, indicating that all plants were well hydrated. Gas exchange parameters were also similar between I+ and I– plants at this stage.
At the end of drought treatment, the SWC of I– pots was significantly lower than that of I+ pots in all three soil layers (). The LER of I– plants was reduced about 50% compared to I+ plants, meanwhile, the LSR of I– plants was approximately three times higher than that of I+ plants (). The leaf DM, stubble DM, shoot DM, root OM and root:shoot ratio were not significantly influenced by water deficit (). The LWP, OP and RWC were reduced from values of −0.2 MPa, −1.0 MPa and 90% for I+ plants to about −0.7 MPa, −1.5 MPa and 70% for I– plants, respectively (). The TP of I− plants remained similar to that of I+ plants (). The LER was positively correlated with the RWC of plants under water deficit and the LSR was negatively correlated with the RWC of plants under water deficit (). As noted above, gas exchange parameters were only measured on cultivar One50. The Gs, Pn and Tr were markedly reduced by water deficit (). Proline concentration increased dramatically in response to water deficit, and was on average about 10 times higher than that of I+ plants (), and the EL was increased by about 50%. However, MDA levels were not significantly increased compared to those of the I+ plants (). After rehydration for one month, the I+ and I− plants did not show a difference in the regrowth leaf DM (). The regrowth leaf DM was negatively correlated with the LER and positively correlated with the level of OA of non-irrigated plants ().
Table 1. Soil water content (w/w, %) (± standard error) of each soil layer in irrigated (I+) and non-irrigated (I–) pots at the end of drought treatment.
Table 2. Means (± standard error) of leaf elongation rate (LER), leaf senescence rate (LSR), leaf dry matter (leaf DM), stubble dry matter (stubble DM), shoot dry matter (shoot DM), root organic matter (root OM), root: shoot ratio, relative water content (RWC), leaf water potential (LWP), osmotic potential (OP), turgor pressure (TP), photosynthesis rate (Pn), stomatal conductance (Gs), transpiration rate (Tr), electrolyte leakage (EL), proline concentration (proline), malondialdehye concentration (MDA), regrowth leaf dry matter (regrowth leaf DM) and osmotic adjustment (OA) of One50 and Commando under irrigated (I+) and non-irrigated (I–) conditions.
Table 3. Correlations between leaf elongation rate (LER), leaf senescence rate (LSR), root organic matter (root OM), shoot dry matter (shoot DM), root: shoot ratio, relative water content (RWC), regrowth leaf dry matter (regrowth leaf DM) and osmotic adjustment (OA) (converted to positive value) of non-irrigated plants.
An interaction between cultivar and irrigation was detected for the LER and proline concentration (). Both One50 and Commando responded to drought with decreased LER and increased proline concentration (). However, Commando had 20% higher LER than One50 under I+ conditions but similar LER to One50 under I− conditions (). The proline concentration was similar for One50 and Commando under I+ conditions, while One50 had 24% higher proline concentration than Commando under I− conditions (). However, this effect on proline concentration was also affected by endophyte status, as indicated by a significant three-factor interaction between cultivar, irrigation and endophyte (three-factor interactions are not shown in ). E+ and E− plants of One50 and Commando had similar proline concentrations under I+ conditions, while under I− conditions, E+ and E− plants of One50 showed a 15% difference in proline concentration but this was not so for Commando ().
Figure 1. Proline concentration of One50 and Commando with endophyte (E+) and without endophyte (E−) under irrigated (I+) and non-irrigated (I−) conditions.
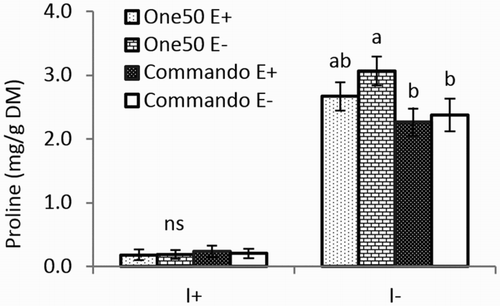
Among all the measured parameters, endophyte status only had a significant effect on the root:shoot ratio, where E+ plants had higher root:shoot ratio than E− plants (Table S1).
Discussion
Plant responses to drought
Based on the SWC of I− pots and the soil water holding capacity of the pot, it was estimated that plants in I− pots consumed 768 ml water on average from each pot during the drought period (soil evaporation from the pot would have been negligible as pots were fully covered by plants). Since plant size was large when drought treatment was initiated, the daily water consumption was 200–250 ml (estimated on a sunny day prior to imposing the drought treatment); thus plants in the I− pots potentially could have dried out in three days, though it is likely the rate of water loss slowed progressively as soil moisture declined. Even so the comparatively sudden drought shock arising from limited soil moisture storage might have produced physiological responses in the experiment different from those typical of field plants.
Plant growth was restricted by water deficit as indicated by decreased LER and increased LSR (). The LSR was even higher than the LER of I− plants (), which would indicate herbage yield reduction. However, despite this, the herbage accumulation of I+ plants and I− plants was not significantly different (), indicating that perhaps the 14-day drought treatment period was too short for significant differences in harvested mass to evolve.
Stomatal closure is usually the earliest plant response to water deficit (Schroeder et al. Citation2001), which enables plants to reduce the transpiration water loss, but with a concomitant reduction of photosynthesis due to restricted CO2 uptake, as indicated by decreased Gs, Pn and Tr of I− plants compared to I+ plants (). When water supply cannot meet the water demand of plants, plants will become dehydrated. Here, the RWC of I− plants was in the range 64−73%, compared with approximately 90% for I+ plants (). The more severe plant dehydration, the more the LER was limited and the greater the LSR, as indicated by negative correlation between LER and RWC and positive correlation between LSR and RWC (). ROS are usually over-produced and cause oxidative damage of organic molecules when plants are under drought stress, resulting in damage to cell membranes, as indicated by increased EL in this experiment (). The non-significant increase of MDA in I– plants compared to I+ plants suggests that lipid peroxidation of perennial ryegrass is less sensitive to drought stress than EL. Farooq et al. (Citation2009) stated that the appearance of lipid peroxidation depends on drought severity. The non-significant increase in MDA observed in the present study could be because the drought stress experienced by plants in this experiment would have been sudden and short.
OA is generally considered a drought tolerance strategy. The active accumulation of compatible solutes under drought decreases OP and thus stimulates water uptake of plants (Turner Citation1986; Blum Citation1996; Zlatev & Lidon Citation2012). Certain types of these solutes help to protect cellular proteins, enzymes and cellular membrane (Chaves et al. Citation2003; Farooq et al. Citation2009). Here, the TP was maintained even though plants were dehydrated (). However, if LWP and OP had been measured at midday, the TP values of I+ and I− plants would probably have been different, as TP decrease induced by water deficit is strongly diurnal, and is more pronounced at midday than in the early morning (Jones et al. Citation1980). Leaf wilting was observed around midday supporting this deduction. The positive correlation between OA and regrowth leaf DM () indicates that the accumulated solutes benefited plant regrowth during recovery from drought. The regrowth leaf DM was also negatively correlated with LER (), which indicates that the more plant growth was restricted during drought, the more solutes accumulated in plant cells. It has been stated that the accumulation of solutes during water deficit is because the cell expansion and elongation rates fall more rapidly than the photosynthesis rate, thus resulting in the supply of photosynthate exceeding its utilisation (Wardlaw Citation1969; Munns & Weir Citation1981; Van Volkenburgh & Boyer Citation1985). The relationship between OA and crop yield was reviewed by Serraj and Sinclair (Citation2002), who pointed out that most published papers indicated no effect or a negative influence of OA on crop yield during drought stress. We hypothesise that in drought-prone environments, the evolutionary benefit of OA is associated with summer survival in temperate perennial grass plants rather than summer growth rate, as higher OA was associated with better plant growth in rehydration but at the cost of more herbage yield reduction during drought stress in this experiment.
Plant species that inhabit dry sites generally have higher root:shoot ratio than those of wet habitats, and the root:shoot ratio is usually increased under drought stress as reviewed by Wu and Cosgrove (Citation2000). Blum (Citation2005) stated that the increased root:shoot ratio mainly arises from reduced shoot growth rather than increased root dry matter; therefore, the root:shoot ratio should not be considered a good indicator of drought tolerance nor a selection criterion for drought tolerant plants. However, in this experiment, it was shown that the increased root:shoot ratio was mainly due to increased root OM rather than decreased shoot DM, as indicated by significant correlation between root:shoot ratio and root OM and non-significant correlation between root:shoot ratio and shoot DM under drought conditions (). This experiment also demonstrated that the increased root OM and root:shoot ratio under drought had no effect on delaying dehydration, as indicated by the non-significant correlations between root:shoot ratio or root OM and RWC (). Sinclair and Muchow (Citation2001) assessed traits for crop yield under drought conditions and found that an increase in rooting depth consistently increased crop yield. Therefore, instead of root total biomass and root:shoot ratio, rooting depth could be one of the selection criteria for enhancing drought tolerance of perennial ryegrass. It is also worth noting that root response to drought is evidently rapid as there was almost 50% greater root mass after just two weeks.
Drought tolerance of One50 and Commando
One50 and Commando were bred from different germplasm sources. One50 incorporates Spanish germplasm while Commando has a close affinity to one of the cultivars derived from the ‘Mangere’ ecotype, Grasslands Nui (Wang et al. Citation2014). In this experiment, an interaction between cultivar and irrigation was detected for LER and proline concentration. One50 had a smaller reduction in LER and a larger increase in proline concentration in response to drought than Commando (), raising a possibility for future investigation that the larger accumulation of proline may partially contribute to the lesser growth restriction of One50. In addition to the improved traits that have been summarised by Stewart (Citation2006), Spanish germplasm probably has also provided enhanced drought protection with continued growth of New Zealand perennial ryegrass. This fact underpins the importance of introducing new germplasm into New Zealand perennial ryegrass populations. These findings support the conclusions of Easton et al. (Citation2011), who also suggested that New Zealand might need to import more genetic resources from other regions in order to cope with climate change. If the trait differences displayed by cultivar One50 are indeed a reflection of traits found in the Spanish ryegrass germplasm previously imported to New Zealand and used in the breeding of this cultivar, then the drought tolerance strategy of this material can be characterised as including a capacity to better maintain LER under drought stress, through physiological adjustments such as greater proline elevation in drought.
These trait combinations differ from those displayed by ryegrass germplasm of North African origin which generally displays a collection of traits referred to as ‘summer dormancy’ (Volaire et al. Citation2009). Use of Mediterranean germplasm to develop ryegrass cultivars for use in Australia has been investigated by Silsbury (Citation1961), but the summer dormancy is not attractive to farmers and the resulting cultivars were not commercially successful. However, both the drought tolerance strategy demonstrated by One50 and possibly linked to Spanish germplasm used in its breeding and the summer dormancy drought tolerance strategy are potentially useful in New Zealand in future as the New Zealand climate is trending towards warmer, drier summers, often referred to as a Mediterranean climate. Further research to assess the comparative benefits of these two different sets of trait combinations found in geographically separated summer-warm regions could be productive. It may be that the strategy demonstrated by One50 is optimal in moderate drought and the so-called summer dormancy strategy of material of Mediterranean origin is more advantageous in more severe summer drought where plant survival rather than plant growth is the critical criterion for drought tolerance.
Role of endophyte
Among all the measured morphological and physiological parameters, the main effect of endophyte status was only detected for root:shoot ratio. The root:shoot ratio was significantly increased by endophyte infection, which was mainly attributable to increased root OM (). Since this endophyte main effect was not associated with any interaction with irrigation treatments, it appears that endophyte-related increase in the root:shoot ratio was independent of water availability. Hesse et al. (Citation2003) also reported that endophyte presence increased root dry matter and root:shoot ratio of three perennial ryegrass genotypes that had been collected from three different sites in Germany with different edaphic characteristics (wet, dry and neither wet or dry). An expectation in industry circles has been that endophyte may enhance drought tolerance through drought specific responses, but in this experiment and in a previous field experiment (He Citation2016), the primary pattern has been that any endophyte-induced trait normally associated with enhanced drought tolerance also occurred in irrigated plants. This means the putative endophyte benefit was constitutive rather than induced, that is, the endophyte already positioned the plant at a higher level of protection ex ante facto. It should be noted that many field studies have found improved persistence during dry conditions with endophyte infection. It might be that endophyte confers an indirect benefit by protecting plants from other stressors, such as insect predation, and that these plants are more tolerant to drought.
The only specifically drought-related response in this experiment was the three-factor interaction where proline accumulation in response to drought was similar between One50 and Commando in E+ plants, while it differed significantly between the two cultivars in E− plants ().
Conclusions
OA has often been considered as a drought adaptation trait and selection criterion; however, here it was demonstrated that in perennial ryegrass, OA was associated with greater plant regrowth during plant rehydration but with a sacrifice of plant growth during drought stress, as indicated by the negative correlation with LER. One50 displayed more continued growth and better protection under drought than Commando, which can probably be attributed to the introduction of Spanish germplasm to One50. Among all the measured morphological and physiological traits, AR37 endophyte infection only significantly increased the root:shoot ratio and this effect was independent of irrigation. Thus it is concluded that AR37 endophyte had no effect on drought tolerance of the host One50 and Commando plants in this experiment.
Table S1
Download MS Word (15.3 KB)Acknowledgements
We thank Stephen Ray, Lesley Taylor and Lindsay Sylva (Plant Growth Unit, Massey University) for support with plant cultivation in the glasshouse. We thank Mark Osborne, Simon Orsborn, Wei Zhang and Yang Liu for help with sample collection. We also thank the anonymous reviewers for their careful reading of our manuscript and their many insightful comments and suggestions.
Disclosure statement
No potential conflict of interest was reported by the authors.
ORCID
C. Matthew http://orcid.org/0000-0002-6151-4828
References
- Amalric C, Sallanon H, Monnet F, Hitmi A, Coudret A. 1999. Gas exchange and chlorophyll fluorescence in symbiotic and non-symbiotic ryegrass under water stress. Photosynthetica. 37:107–112. doi: 10.1023/A:1007027131613
- Armstrong CS. 1977. ‘Grasslands Nui’perennial ryegrass (Lolium perenne L.). New Zealand Journal of Experimental Agriculture. 5:381–384. doi: 10.1080/03015521.1977.10426000
- Barker D, Chu A, Korte C. 1985a. Some effects of spring defoliation and drought on perennial ryegrass swards. Proceedings of the New Zealand Grassland Association. p. 57–63.
- Barker DJ, Chu A, Korte C. 1985b. Depletion of soil water from a Tokomaru silt loam by a perennial ryegrass sward. New Zealand Journal of Experimental Agriculture. 28:525–530. doi: 10.1080/00288233.1985.10417998
- Bayat F, Mirlohi A, Khodambashi M. 2009. Effects of endophytic fungi on some drought tolerance mechanisms of tall fescue in a hydroponics culture. Russian Journal of Plant Physiology. 56:510–516. doi: 10.1134/S1021443709040104
- Begg JE. 1980. Morphological adaptations of leaves to water stress. In: NC Turner, PJ Kramer, editor. Adaptation of plants to water and high temperature stress. New York: John Wiley & Sons; p. 33–42.
- Blum A. 1989. Osmotic adjustment and growth of barley genotypes under drought stress. Crop Science. 29:230–233. doi: 10.2135/cropsci1989.0011183X002900010052x
- Blum A. 1996. Crop responses to drought and the interpretation of adaptation. Plant Growth Regulation. 20:135–148. doi: 10.1007/BF00024010
- Blum A. 2005. Drought resistance, water-use efficiency, and yield potential—are they compatible, dissonant, or mutually exclusive? Australian Journal of Agricultural Research. 56:1159–1168. doi: 10.1071/AR05069
- Blum A, Ebercon A. 1981. Cell-membrane stability as a measure of drought and heat tolerance in wheat. Crop Science. 21:43–47. doi: 10.2135/cropsci1981.0011183X002100010013x
- Bokhari UG, Trent JD. 1985. Proline concentrations in water stressed grasses. Journal of Range Management. 38:37–38. doi: 10.2307/3899329
- Chai Q, Jin F, Merewitz E, Huang B. 2010. Growth and physiological traits associated with drought survival and post-drought recovery in perennial Turfgrass species. Journal of the American Society for Horticultural Science. 135:125–133.
- Chaves MM, Maroco JP, Pereira JS. 2003. Understanding plant responses to drought—from genes to the whole plant. Functional Plant Biology. 30:239–264. doi: 10.1071/FP02076
- Easton HS, Stewart AV, Kerr GA. 2011. Ryegrass in pastures—breeding for resilience. New Zealand Grassland Associaiton Grassland Research and Practice. 15:139–148.
- Easton HS, Baird DB, Cameron NE, Kerr GA, Norriss M, Stewart AV. 2001. Perennial ryegrass cultivars: herbage yield in multi-site plot trials. Proceedings of the New Zealand Grassland Association. 63:183–188.
- Elmi AA, West CP. 1995. Endophyte infection effects on stomatal conductance, osmotic adjustment and drought recovery of tall fescue. New Phytologist. 131:61–67. doi: 10.1111/j.1469-8137.1995.tb03055.x
- Farooq M, Wahid A, Kobayashi N, Fujita D, Basra SMA. 2009. Plant drought stress: effects, mechanisms and management. Agronomy for Sustainable Development. 29:185–212. doi: 10.1051/agro:2008021
- Fu JM, Huang BR. 2001. Involvement of antioxidants and lipid peroxidation in the adaptation of two cool-season grasses to localized drought stress. Environmental and Experimental Botany. 45:105–114. doi: 10.1016/S0098-8472(00)00084-8
- Gomes FP, Oliva MA, Mielke MS, Almeida A-AF, Aquino LA. 2010. Osmotic adjustment, proline accumulation and cell membrane stability in leaves of Cocos nucifera submitted to drought stress. Scientia Horticulturae. 126:379–384. doi: 10.1016/j.scienta.2010.07.036
- Hamilton EW, Heckathorn SA. 2001. Mitochondrial adaptations to NaCl. Complex I is protected by anti-oxidants and small heat shock proteins, whereas complex II is protected by proline and betaine. Plant Physiology. 126:1266–1274. doi: 10.1104/pp.126.3.1266
- Hatier J-HB, Faville MJ, Hickey MJ, Koolaard JP, Schmidt J, Carey B-L, Jones CS. 2014. Plant vigour at establishment and following defoliation are both associated with responses to drought in perennial ryegrass (Lolium perenne L.). Journal of Experimental Botany. 65:5823–5834. doi: 10.1093/jxb/eru318
- He L. 2016. Drought tolerance of perennial ryegrass (Lolium perenne L.) and the role of Epichloë endophyte. Unpublished A thesis presented in partial fulfilment of the requirements for the degree of Doctor of Philosophy in Plant Science thesis, Massey University, Palmerston North.
- Hesse U, Schoberlein W, Wittenmayer L, Forster K, Warnstorff K, Diepenbrock W, Merbach W. 2003. Effects of Neotyphodium endophytes on growth, reproduction and drought-stress tolerance of three Lolium perenne L. genotypes. Grass and Forage Science. 58:407–415. doi: 10.1111/j.1365-2494.2003.00393.x
- Jiang Y, Huang B. 2001. Physiological responses to heat stress alone or in combination with drought: a comparison between tall fescue and perennial ryegrass. Hortscience. 36:682–686.
- Johnson LJ, de Bonth AC, Briggs LR, Caradus JR, Finch SC, Fleetwood DJ, Fletcher LR, Hume DE, Johnson RD, Popay AJ, et al. 2013. The exploitation of epichloae endophytes for agricultural benefit. Fungal Diversity. 60:171–188. doi: 10.1007/s13225-013-0239-4
- Jonaviciene K, Statkeviciute G, Kemesyte V, Brazauskas G. 2014. Genetic and phenotypic diversity for drought tolerance in perennial ryegrass (Lolium perenne L.). Zemdirbyste-Agriculture. 101:411–418. doi: 10.13080/z-a.2014.101.052
- Jones MB, Leafe EL, Stiles W. 1980. Water-stress in field-grown perennial ryegrass.I. Its effect on growth, canopy photosynthesis and transpiration. Annals of Applied Biology. 96:87–101. doi: 10.1111/j.1744-7348.1980.tb04772.x
- Kane KH. 2011. Effects of endophyte infection on drought stress tolerance of Lolium perenne accessions from the Mediterranean region. Environmental and Experimental Botany. 71:337–344.
- Livestock Improvement Corporation Ltd. and DairyNZ Ltd. 2015. New Zealand Dairy Statistics.
- Malinowski D, Leuchtmann A, Schmidt D, Nosberger J. 1997. Symbiosis with Neotyphodium uncinatum endophyte may increase the competitive ability of meadow fescue. Agronomy Journal. 89:833–839. doi: 10.2134/agronj1997.00021962008900050019x
- Meloni DA, Oliva MA, Ruiz HA, Martinez CA. 2001. Contribution of proline and inorganic solutes to osmotic adjustment in cotton under salt stress. Journal of Plant Nutrition. 24:599–612. doi: 10.1081/PLN-100104983
- Munns R, Weir R. 1981. Contribution of sugars to osmotic adjustment in elongating and expanded zones of wheat leaves during moderate water deficits at two light levels. Australian Journal of Plant Physiology. 8:93–105. doi: 10.1071/PP9810093
- Nagabhyru P, Dinkins RD, Wood CL, Bacon CW, Schardl CL. 2013. Tall fescue endophyte effects on tolerance to water-deficit stress. BMC Plant Biology. 13:127. doi: 10.1186/1471-2229-13-127
- NIWA. 2013. Droughts. Retrieved February 25 2016, from https://www.niwa.co.nz/natural-hazards/hazards/droughts.
- Samuel D, Kumar TKS, Ganesh G, Jayaraman G, Yang PW, Chang MM, Trivedi VD, Wang SL, Hwang KC, Yu C, et al. 2000. Proline inhibits aggregation during protein refolding. Protein Science. 9:344–352. doi: 10.1110/ps.9.2.344
- Scholander PF, Hammel HT, Bradstreet ED, Hemmingsen EA. 1965. Sap Pressure in Vascular Plants: Negative hydrostatic pressure can be measured in plants. Science. 148:339–346. doi: 10.1126/science.148.3668.339
- Schroeder JI, Allen GJ, Hugouvieux V, Kwak JM, Waner D. 2001. Guard cell signal transduction. Annual Review of Plant Physiology and Plant Molecular Biology. 52:627–658. doi: 10.1146/annurev.arplant.52.1.627
- Schuurman JJ, Goedewaagen MAJ. 1965. Methods for the examination of root systems and roots: methods in use at the Institute for Soil Fertility for ecomorphological root investigations. Wageningen: Centre for Agricultural Publications and Documents.
- Serraj R, Sinclair TR. 2002. Osmolyte accumulation: can it really help increase crop yield under drought conditions? Plant, Cell and Environment. 25:333–341. doi: 10.1046/j.1365-3040.2002.00754.x
- Silsbury JH. 1961. A study of dormancy, survival and other characteristics in Lolium perenne L. at Adelaide, SA. Australian Journal of Agricultural Research. 12:1–9. doi: 10.1071/AR9610001
- Simpson WR, Schmid J, Singh J, Faville MJ, Johnson RD. 2012. A morphological change in the fungal symbiont Neotyphodium lolii induces dwarfing in its host plant Lolium perenne. Fungal Biology. 116:234–240. doi: 10.1016/j.funbio.2011.11.006
- Sinclair TR, Muchow RC. 2001. System analysis of plant traits to increase grain yield on limited water supplies. Agronomy Journal. 93:263–270. doi: 10.2134/agronj2001.932263x
- Stewart A. 2006. Genetic origins of perennial ryegrass (Lolium perenne) for New Zealand pastures. Proceedings of the 13th Australasian Plant Breeding Conference. Christchurch, New Zealand. p. 11–20.
- Thomas H, James AR. 1999. Partitioning of sugars in Lolium perenne (perennial ryegrass) during drought and on rewatering. New Phytologist. 142:295–305. doi: 10.1046/j.1469-8137.1999.00388.x
- Turner NC. 1981. Techniques and experimental approaches for the measurement of plant water status. Plant and Soil. 58:339–366. doi: 10.1007/BF02180062
- Turner NC. 1986. Adaptation to water deficits – a changing perspective. Australian Journal of Plant Physiology. 13:175–190. doi: 10.1071/PP9860175
- Van Volkenburgh E, Boyer J. 1985. Inhibitory effects of water deficit on maize leaf elongation. Plant Physiology. 77:190–194. doi: 10.1104/pp.77.1.190
- Volaire F, Norton MR, Lelièvre F. 2009. Summer drought survival strategies and sustainability of perennial temperate forage grasses in Mediterranean areas. Crop Science. 49:2386–2392. doi: 10.2135/cropsci2009.06.0317
- Wang J, Pembleton LW, Baillie RC, Drayton MC, Hand ML, Bain M, Sawbridge TI, Spangenberg GC, Forster JW, Cogan NOI. 2014. Development and implementation of a multiplexed single nucleotide polymorphism genotyping tool for differentiation of ryegrass species and cultivars. Molecular Breeding. 33:435–451. doi: 10.1007/s11032-013-9961-6
- Wardlaw IF. 1969. Effect of water stress on translocation in relation to photosynthesis and growth. II. Effect during leaf development in Lolium temulentum. Australian Journal of Biological Sciences . 22:1–16.
- Wobbrock JO, Findlater L, Gergle D, Higgins JJ. 2011. The aligned rank transform for nonparametric factorial analyses using only ANOVA procedures. Proceedings of the SIGCHI Conference on Human Factors in Computing Systems: 143–146.
- Wu YJ, Cosgrove DJ. 2000. Adaptation of roots to low water potentials by changes in cell wall extensibility and cell wall proteins. Journal of Experimental Botany. 51:1543–1553. doi: 10.1093/jexbot/51.350.1543
- Zlatev Z, Lidon FC. 2012. An overview on drought induced changes in plant growth, water relations and photosynthesis. Emirates Journal of Food and Agriculture. 24:57–72. doi: 10.9755/ejfa.v24i6.14669