ABSTRACT
The objective was to determine the effect of grazing fodder beet versus ryegrass-dominant pasture from mid-to-late gestation on dam and offspring performance under practical farming conditions. Twin-bearing ewes were randomly allocated to grazing regimes of either fodder beet plus ad libitum ryegrass/clover hay (FB; n = 100) or ryegrass-dominant pasture plus ad libitum ryegrass/clover hay (RG; n = 100) with three replicates per group, from pregnancy day (P) 100–110 to birth. At P135, 10 ewes per treatment were randomly selected from each replicate, slaughtered and back fat and eye muscle depth and fetal body weight and composition were measured. Samples were evaluated for selected blood metabolites. Ewe BW and BCS change and lamb growth were measured, and lamb survival from birth to weaning calculated. Compared with RG, FB ewes had lower ADG and overall lower BCS resulting from increased mobilisation of both fat and muscle. Lambs born to FB-ewes had lower BW and postnatal growth rates and increased mortality from birth to weaning (29% versus 12%). Body composition and blood parameters indicated that FB compared to RG ewes were subject to undernutrition. This study provides new insights into the consequences of FB grazing regimes, as an alternative to ryegrass pasture, on animal performance.
Introduction
Ruminant livestock production in New Zealand and other temperate regions is largely pasture-based and aligned to seasonal pasture supply. In such systems, pasture growth rate varies over the annual cycle and there are periods when pasture quantity and quality is insufficient to meet the requirements of livestock. One approach to fill feed deficits is using forage crops with reliable, fast-growing characteristics within a wide range of climatic conditions (Dalley and Geddes Citation2012). Fodder beet (Beta vulgaris L.) is a crop (leaf and bulb combined) that has a high dry matter (DM) yield and low cost per unit of feed, compared with other crops and supplementary feeds (Milne et al. Citation2014), and is characterised by high sugar and low crude protein (CP) and neutral detergent fibre (NDF) contents (Clark et al. Citation1987). Although average daily gain (ADG) responses in growing beef cattle fed fodder beet have been evaluated (Gibbs et al. Citation2015), less is known how suitable a fodder beet winter grazing regime is to support nutritional requirements of the pregnant ewe and her progeny.
Anecdotally, fodder beet is increasingly being used as an alternative forage option for pregnant ewes to provide winter feed in temperate areas of New Zealand. However, there is no published literature available that describes the potential benefits or trade-offs of grazing pregnant ewes on fodder beet on ewe and lamb performance. Therefore, there is a need to understand how this feed impacts dam and offspring performance, especially during mid-to-late gestation when foetal nutritional demands are the highest (Kenyon and Webby Citation2007). Feeds that contain a low DM content, such as fodder beet, should be avoided in late gestation because it is difficult for ewes to consume enough DM to meet specific nutrient requirements such as CP and NDF. Pregnant ewes in mid-late gestation have a high demand for protein and specific amino acids to meet the demands of the developing conceptus (McCoard et al. Citation2016). Therefore, it is reasonable to speculate that a diet low in both DM and CP contents may have negative effects on the production performance of pregnant ewes and their progeny. The impact of a FB winter grazing regime for meeting the nutritional requirements of pregnant ewes, and on their production performance is poorly understood. The objective of this study was to determine the effects of fodder beet winter grazing regime (as an alternative forage source) compared with ryegrass-dominant pasture (Lolium perenne L.) grazing regime from mid-to-late gestation on dam and offspring performance under practical farming conditions.
Materials and methods
Experimental design
The animal trial was conducted in the Manawatu region of New Zealand (latitude 40°19′S, longitude 175°29″, 13 m above sea level) in spring (late August to early September lambing) in 2016. All animal manipulations in this study were conducted in compliance with the institutional Code of Ethical Conduct for the Use of Animals in Research, Testing, and Teaching, as prescribed in the Animal Welfare Act of 1999 and its amendments (New Zealand). The AgResearch Grasslands Animal Ethics Committee (Approval number 13911) approved the manipulations.
Two hundred twin-bearing, mixed-age ewes were sourced from two farms (Farm 1, n = 38, or Farm 2, n = 162), stratified according to farm source, estimated parturition date, ewe body weight (BW) and body condition score (BCS) and randomly allocated to two winter grazing groups: (1) FB: fodder beet plus ad libitum ryegrass/clover hay (n = 100 split evenly across three replicated paddocks) or (2) RG: ryegrass-dominant pasture plus ad libitum ryegrass/clover hay (n = 100 split evenly across three replicated paddocks). Paddocks within each treatment were a similar size and stocking density. Ewes within each mob remained in their assigned paddock for the duration of the treatment period and until the end of the transition back to a 100% ryegrass-dominant pasture diet. Thereafter, the ewes in all mobs were managed on a ryegrass-dominant pasture as a single group until weaning. According to recommended practice (Gibbs and Saldias Citation2014), FB ewes in each mob were adapted to the winter fodder beet crop over 21 days from an average of 85 days pregnancy (P85), which entailed on/off grazing between ryegrass-dominant pasture and fodder beet crop paddocks. The duration ewes spent on the fodder beet crop increased daily, so that by day 16 of the adaptation period ewes remained on the fodder beet crop with hay and no pasture available. From an average of P105 until onset parturition, the ewes in each mob were grazed on either the fodder beet crop (average of 43 days) or ryegrass-dominant pasture. Around the start of parturition (average of 9 days prior to parturition), which was spread over a 5-week period, FB ewes in each mob were transitioned over 7 days back to ryegrass-dominant pasture. Fodder beet plants were harvested daily by hand and offered whole to the FB ewes (both pregnant and lambed) in the pasture paddocks to support the transition of FB ewes back to pasture, and to enable all ewes to have access to the fodder beet crop up to parturition. This occurred until the last ewe lambed. Ryegrass/clover hay was provided in feeding racks to ewes during adaptation and throughout the experimental period which was from P105 to parturition. The RG ewes in each mob were managed on a ryegrass-dominant pasture with ad libitum access to ryegrass/clover hay throughout pregnancy (i.e. no change in diet or requirement for dietary transition). Once all ewes in each mob had lambed (i.e. end of the treatment period), the ewes were managed as a single mob on a ryegrass-dominant pasture until weaning.
Animals
Foetal age (and therefore days of pregnancy (P)) was estimated on the same day for all animals via transabdominal ultrasonography of the ewes at approximately 75–78 days after the start of the mating period. Ewe BCS (Jefferies Citation1961; scale 0–5 including half units) was recorded by a single trained operator at the same time as pregnancy scanning.
All ewes were vaccinated against Clostridia (Coopers Nilvax Selenised, MSD Animal Health, Wellington, New Zealand), Salmonella (Salvexin + B, MSD Animal Health, Wellington, New Zealand) and Campylobacter (CampyVax 4, MSD Animal Health, Wellington, New Zealand) at P100, and injected with a slow-release source of iodine at P120 to avoid deficiency of this mineral (Depodine, Alleva Animal Health, Auckland, New Zealand) due to the low iodine content in the FB diet.
Winter grazing forage treatments
The fodder beet used in the FB treatment was cultivar ‘Rivage’ which had an estimated yield of 30 t DM/ha at the beginning of the adaptation period. Crop yield was estimated independently by an agronomist using industry-standard methods (Agricom, Christchurch, New Zealand) where selected fodder beet from 3 sampling sites within each paddock immediately prior to the start of the adaptation period, were cleaned and fresh weight (FW) measured of both leafy top and bulb. The average wet weight for an area of 2 m2 was determined to give kg FW/ha which was then multiplied by the DM concentration to derive kg DM/ha. For DM determination, fodder beet bulbs were chopped into 5 cm portions and the DM content (for both leaf and bulb) was determined by oven drying (48 h at 105°C) a representative subsample from 3 to 5 of the fodder beet plants from each sampling site.
Due to a lack of feeding recommendations for pregnant ewes grazing fodder beet crops, ewes were offered ad libitum fodder beet and ryegrass/clover hay. However, to ensure both leaf and bulb were consumed, ewes were provided with a fresh allocation of crop every second day and were allowed to back graze bulbs left behind (i.e. no back fencing). Ewes in the RG group were offered pasture of an average pre-grazing yield of 2800 kg DM/ha and ab libitum hay. Pasture was managed to maintain a minimum cover of 1200 kg DM/ha at all times, according to the recommendations of Morris and Kenyon (Citation2004), who suggested ewes rearing twins should be fed sward height allowances of 4 cm (i.e. 1180 kg DM/ha) or better during gestation. A fresh allocation of pasture was provided every second day and, consistent with the FB group, no back fencing was used.
Ewes in both grazing treatments were provided fresh drinking water in troughs and supplemented with ryegrass/clover hay ad libitum.
Diet DM and chemical composition
Samples of fodder beet (leaf and bulb) and ryegrass-dominant pasture (taken at P80 and P147), and hay (taken at P80) were collected from each paddock grazed. A composite sample made up of over 50 individual samples taken at various sites within each paddock/bale for each feed was analysed. The FW ratio of fodder beet bulb to leaf was also determined. Triplicate sub-samples from each feed composite were dried at 105⁰C for 48 h for DM determination (method 930.15; AOAC Citation2005). Further duplicate sub-samples were submitted for chemical composition analyses (Massey University Nutrition Laboratory, Palmerston North, New Zealand) after being freeze-dried and ground using a Wiley mill (Thomas Scientific, Swedesboro, NJ, USA) fitted with a 1 mm screen. The analyses were performed following the procedures of the AOAC (Citation1990) for ash (method 942.05), crude fat (method 991.36), CP (method 968.06), soluble sugars and starch (method 996.11). Analysis of structural carbohydrates included NDF assayed with heat-stable α-amylase, ADF and ADL in sulfuric acid (Robertson and Van Soest Citation1981), while in vitro enzymatic organic matter digestibility of DM (DOMD) was determined according to Roughan and Holland (Citation1977). Metabolisable energy was calculated from DOMD, according to SCA (Citation1990) which assumes that 16% of the energy in DOMD is lost as methane and urine during digestion.
Estimated feed allowance
Estimated pasture allowance for the RG group was based on measured DM and pre- and post-grazing pasture mass determined using the default equation of a rising plate meter (FarmWorks F400, Ag Hub, Tauranga, New Zealand) every 2 d for each paddock grazed. For FB ewes, feed allowance was calculated using measured DM, estimated initial FB yield, and the area of fodder beet grazed every 2 d for each mob. Animals were not back fenced for either treatment to allow ad libitum intake of FB and therefore backgrazing of FB bulbs left behind after every allocation. This meant that feed utilisation and therefore feed intake was not determined.
For estimate of hay consumed within each paddock, in addition to measurement of hay DM, individual hay bales were weighed before going into hay racks and then reweighed every week thereafter. The disappearance of hay over this week was used to estimate the daily amount consumed over this timeframe.
Ewe performance
Ewe BW and BCS were determined by the same operator at P80, P100, P120, P135, and approximately 40 (lamb tail removal) and 100 (lamb weaning) days after birth and used for calculating ADG between each time point. Rumen fluid samples were collected by stomach tube from a subset of ewes in each treatment group (n = 12/treatment randomly selected from each mob from farm 2; the same animals used for post-mortem evaluations) either in the morning (AM) or afternoon (PM) approximately 2 or 6 h after receiving their new allocation of fresh feed respectively, on P135. The samples were stored at −20°C until analysis of short-chain fatty acids (SCFA) using previously described methods (Hammond et al. Citation2013; Tavendale et al. Citation2005). Ewes were randomly selected for slaughter from a group that had been foetal aged and predicted to have similar due dates (± 6 d), across each mob per treatment from farm 2. Blood samples were collected from the ewes selected for slaughter by jugular venipuncture on P133 using 10 ml potassium-EDTA containing vacutainers (Becton Dickinson Ltd., Auckland, NZ). Plasma was harvested and analysed for total protein, glucose, triglycerides, NEFA, urea and beta-hydroxybutyrate using the Roche modular platform P800 module using Randox kits (Randox Laboratories Ltd, UK) and previously described methods (McCoard et al. Citation2019). On the day of slaughter (P135), ewes were removed from their grazing paddocks 1 h before transport to the abattoir, weighed and BCS determined.
Ewes were euthanised using a captive bolt gun for stunning, followed by exsanguination. The maternal liver, kidney and perirenal fat (left and right), spleen and mammary gland were dissected free from skin and connective tissue and weighed. Ewe carcass composition was also measured on chilled carcasses from a subset of ewes at P135 (n = 10/dietary treatment) pre-parturition. Carcass composition measurements included carcass fat C, which was the measured depth of fat over the depth of the eye muscle at the 12th rib; carcass fat GR, which was the total tissue depth to the 12th rib but 10 cm from the midline; and eye muscle depth and width, which were measured at the max depth and width of the eye muscle at the 12/13th rib.
Foetal growth and body composition and lamb growth and survival
Following euthanasia of the subset of ewes at P135, the foetuses were exposed, a blood sample collected using cardiac puncture followed by euthanasia of the foetus via an overdose of barbiturate. Foetal weight, sex, crown-rump length (CRL), hind leg length (HLL), and heart girth were recorded. The liver, kidneys and perirenal fat (left and right), lungs, heart, spleen, pancreas, adrenals, thymus, thyroids, large intestine (empty), small intestine (empty) and semitendinosus skeletal muscle were dissected free of connective tissue and weights recorded along with the length of the small and large intestine.
At birth, lamb birth weight, body size (CRL, HLL), sex, and rectal temperature were obtained within 12 h of birth from live lambs. Lamb performance was determined by measuring the growth rate up to weaning, where lambs were weighed at an average of 40 (tail removal: ‘docking’) and 100 (weaning) days postpartum. Lamb survival was measured as the number of dead or orphaned lambs, collected daily over the periods of scanning to birth, birth to 3 days, 3–40 days, and 40–100 days postpartum.
Statistical analyses
No statistical analyses on diet chemical composition were undertaken due to diet being the treatment rather than a response. Results provided are therefore descriptive only.
All analyses were conducted using GenStat 19th edition (VSN International Ltd.), except where otherwise stated. For all variables, the transformation of data (where indicated) was performed to meet the assumptions of normality and homogeneity. Fisher’s Least Significant Differences were used as the post-hoc test at α = 0.05. The experimental unit for the live animal analyses was paddock (3 replicates for each grazing treatment), and for the post-mortem analyses was ewe or lamb, indicated by the random effect. For post-mortem analyses, all ewes were randomly selected from one source farm.
Ewe performance: Ewe performance variables included BW, ADG, BCS and carcass composition. Quadratic regression models (using R statistical software; R Core Team Citation2016) were fitted to ewe BW and BCS between scanning and weaning, and linear regression models (VSN International Citation2015) were fitted to ADG prior to parturition. The random coefficient models utilised enabled separate slopes and intercepts to be fitted for the random effects of paddock (i.e. replicate) and ewe. The fixed effects were treatment (FB and RG) and farm origin (Farms 1 and 2) and the interactions. Ewe ADG between parturition and docking, and between parturition and weaning, were analysed using a linear mixed-effects model, with the fixed effect of treatment (FB and RG) and the covariate day since parturition.
Ewe weight and body condition score were analysed using Repeated Measurements Linear Mixed Model (REML) for the factors Period (P100, P120, P135, docking and weaning) and forage grazing treatment (FB and RG), with the random effect of ewe nested within paddock. Average daily gain was analysed using a Linear Mixed Model (REML) for the factors Period (P100, P120, P135, docking and weaning) and forage grazing treatment (FB and RG), with the random effect of ewe nested within paddock.
Ewe post-mortem: Ewe carcass composition data (carcass fat C and GR, eye muscle depth and width, liver weight, kidney, weight, perirenal fat weight, spleen weight, mammary gland weight) were analysed using a linear mixed-effects model, with the fixed effect of treatment (FB and RG), and the random effect of ewe. The data for mammary gland weight were log-transformed.
Ewe plasma: One-way analysis of variance (ANOVA) was carried out on ewe plasma total protein, non-esterified fatty acids (NEFA), triglycerides, and urea for the fixed effect of treatment (FB and RG), and the random effect of ewe. The data for NEFA were log-transformed, and urea square-root transformed. Non-parametric Kruskal–Wallis tests were carried out on glucose and β-hydroxybutyrate for the fixed effect of treatment (FB or RG) due to the data failing to meet the ANOVA assumptions of normality and homogeneity.
Foetal plasma: Foetal plasma metabolite data were analysed for the fixed effect of treatment (FB or RG) and with the random effect of foetus nested within ewe. The response variables were total protein, glucose (which was square-root transformed), NEFA and triglycerides (which were log-transformed) and urea.
Rumen SCFA: Linear mixed models were used to analyse rumen SCFA data from the ewes for the fixed effects of treatment (FB or RG) and time of sampling (AM or PM) and the random effect of ewe. The response variables were total SCFA concentration, acetic:propionic ratio, and the concentrations and molar proportions (mol of each SCFA per 100 moles of total SCFA) of acetate, propionate, butyrate, caproate, isobutyrate, isovalerate and valerate. The data for the concentration of butyrate and caproate and molar proportion of caproate were square-root transformed, and data for molar proportions of butyrate, isobutyrate and isovalerate were log-transformed.
Foetal growth: Linear mixed models were used to analyse foetus growth data were analysed for the fixed effects of winter forage grazing system (FB or RG), gender of the foetus (male or female) and presence of colostrum in the ewe (present or absent; indicator of proximity to parturition) and the random effects were foetus nested within ewe. The response variables were foetal weight, crown-rump length (CRL), hind leg (HHL), CRL:girth ratio, heart girth, weight of liver, kidneys, perirenal fat, lung, heart, spleen (which was log-transformed), pancreas, adrenal, thymus, thyroid, large intestine, small intestine and semitendinosus skeletal muscle, and length of small and large intestines. Ewe weight at pregnancy scanning was used as the covariate for the analysis of foetal weight data, and foetal weight was used as the covariate for the other analyses.
Lamb growth and development data: Lamb growth and development data were analysed using linear mixed models. The response variables crown rump length (CRL), hind leg (HHL), CRL:girth ratio, The fixed effects were the winter forage grazing system (FB and RG), farm origin (1 or 2) and lamb sex (male or female), and the random effects were lamb nested within ewe within paddock. A Chi-square (Χ2) test of independence was carried out using software package Minitab 18 (Minitab Inc.) to test for a significant association between winter forage grazing system (FB and RG) and whether lamb birth rectal temperature was above or below 38.5°C, due to the lack of normality of the data.
Lamb survival: Binary logistic regression analysis was used to test for lamb survival rates between two measurement periods, with survival as the binary response variable (lamb survived or died) and the predictor of grazing system (FB and RG), using the 3 paddocks per grazing system.
Results and discussion
Fodder beet is marketed in New Zealand for its high yields of DM and being cost-competitive. These attributes make FB a feed option worth pursing in New Zealand pastoral farming systems. Therefore, fodder beet is an attractive feed source for all classes of grazing livestock (Dalley and Geddes Citation2012), particularly in winter when it can make up a large proportion of the diet (i.e. >60%) during periods of pasture deficits. However, these benefits can be jeopardised if any nutritional shortcomings of FB, when grazed by different stock classes with varying nutrient requirements, are not identified and mitigated.
To the authors’ knowledge, this is the first study to investigate the impact of grazing multiple-bearing ewes on a FB-based diet in mid-to-late gestation when foetal nutritional demands are the greatest. It is well established that nutritional restriction of the dam, through the restricted intake, dietary imbalances or deficiencies, leads to restricted foetal growth, and poor survival outcome (McCoard et al. Citation2017). With this in mind, we hypothesised that the FB diet would be energy-dense and of sufficient quality to maintain ewe BW and BCS, but the low CP content of the diet would lead to adverse effects for the offspring. The findings from this study illustrate that grazing twin-bearing ewes in mid-late gestation on a fodder beet crop with supplementary hay compared with a ryegrass-dominant pasture has detrimental effects on dam and offspring performance particularly ewe body condition and lamb survival and growth to weaning. While this study does not identify the underlying causes of these effects (e.g. DMI limitation, energy:protein imbalances, protein deficiency etc.), some insights into potential mechanisms that warrant further investigation are discussed.
Diet chemical composition and estimated feed allowance
The estimated chemical composition of the diets offered (fodder beet, ryegrass-dominant pasture and ryegrass/clover hay), as well as the composition of actual individual dietary components, are given in . The estimated CP concentration of the FB treatment offered was almost half that of the RG treatment (118 vs. 224 g CP/kg DM) and almost one third lower in NDF concentration (293 vs. 429 g NDF/kg DM).
Table 1. Chemical composition (g/kg DMa) of individual diet components (fodder beet (FB), ryegrass-dominant pasture and ryegrass/clover hay) and the FB and RG treatment diets grazed by twin-bearing ewes in mid-to-late gestation.
As this was a grazing study, the main limitation was the inability to accurately estimate animal feed intakes, both on a treatment group basis and individually. Only the hay supplement could be measured with accuracy, however, it is uncertain what proportions of FB vs. hay made up the individual animal’s diet and therefore whether individual animal nutrient requirements were fully met. Estimated feed offered (kg DM/ewe/d) for ewes on the FB treatment was 1.8 of fodder beet, with 0.5 of hay consumed daily (estimated from daily hay disappearance from the rack based on visual assessment of residuals). The hay was estimated to contribute to about 28% of total daily feed offered. The hay was offered as a fibre source, given the expected high non-structural carbohydrate content of the FB, with the FB plus hay combination offered containing 293 g NDF/kg DM. Feed offered for ewes on the RG treatment was estimated to be about 1.2 kg DM/ewe/d. Although hay was offered to ewes on the RG treatment, it was not consumed (i.e. there was no hay disappearance from the rack). Based on estimated feed offered (kg DM/animal/d) of 2.3 for the fodder beet/ hay treatment and 1.2 for the RG treatment, ME offered was doubled for FB compared with RG ewes (26 vs 13 MJ ME/d).
There is limited data on minimum fibre requirements for sheep. However, the amount of NDF offered in the FB diet was less than the 400 g/kg DM suggested as optimum to maintain rumen fermentation and milk composition in dairy cows (SCA Citation1990) and less than the recommended minimum NDF content of 350 g/kg DM for cows grazing pasture (Waghorn et al. Citation2007). Thus, for the FB treatment, NDF appeared to be limiting. This has repercussions on the ability of the pregnant ewe to effectively utilise the supply of energy from FB, which was estimated to be almost double that of RG-fed ewes regarding ME intake.
Although FB had negative consequences on both ewe and offspring performance and lamb survival when fed to ewes in mid-to-late gestation, it provides a cost-effective feed option in times of pasture shortages. However, the results of this study provide for the first time in the literature, the data to support the recommendation that fodder beet should be avoided as the main feed source for multiple-bearing ewes in mid-late pregnancy. Alternative feeding management which mitigates the nutritional short comings of FB when fed to multiple-bearing sheep requires further investigation. For example, the stage of gestation during which FB is fed and animal physiological state, the proportion of FB in the diet, and the addition of alternative supplements which can complement the nutritional deficiencies of FB, are all factors that will impact the animal response to a winter FB diet. Further research on the mechanisms underpinning the effect of a FB versus a traditional RG grazing regime, on offspring phenotypes and lifetime performance is needed to inform the development of feeding recommendations which meet the requirements of pregnant ewes to support both dam and offspring performance.
Ewe performance
The ADG (P < .001) and ewe BW (P < .001) between pregnancy, scanning, and parturition (P76 to birth), declined in the FB treatment when compared with the RG treatment (B). Ewes on the FB compared with the RG treatment also had lower BCS after 120 days gestation (C). There was a diet by day interaction ewe BW (P < .001) and ADG (P < .001) between scanning and parturition, whereby FB ewes gained less weight than RG ewes over that period. At ∼P135, compared with the RG ewes, FB ewes had lower longissimus dorsi muscle depth and width, with a trend towards less carcass fat GR and fat C (). These observations indicate greater muscle and fat mobilisation to support foetal growth, consistent with the reduction in ewe BW. These observations indicate that the ewes grazing FB were nutritionally restricted compared with RG ewes during pregnancy, despite the greater feed allowance. As intake of the ewes on either could not be measured directly in this study, it is not possible to determine whether feed intake and/or quality (e.g. CP content, energy:protein ratio) contributed to the greater mobilisation of body reserves in the ewes on the FB treatment.
Figure 1. A, Mean BW (kg) in relation to day of pregnancy, B, mean average daily gain (ADG. kg/d) and C, mean body condition score (BCS) for twin-bearing ewes grazing dietary treatments of either fodder beet or ryegrass-dominant pasture from mid-to-late gestation, and pasture only from parturition onwards. Shading represents the SEM. Vertical lines at 147, 185 and 245 d represent parturition, docking (i.e. tail removal at ∼40 days post-partum) and weaning (∼100 days post-partum), respectively.
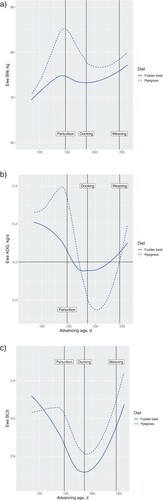
Table 2. Carcass composition and organ weights at gestation d 135 (P135; n=10/group) and selected plasma metabolites (n = 6/group) from twin-bearing ewes grazing dietary treatments of either fodder beet (FB) or ryegrass-dominant pasture (RG) from mid-to-late gestation.
Despite the CP content of the diet offered in this study (assumed average composition based on the feed offered) being within NRC (Citation2007) recommendations for non-lactating ewes in late gestation (i.e. no less than 11% CP), this recommendation is for single-bearing, while twin-bearing ewes’ requirements are more likely in the range of 14–18% (Stevens Citation1999). However, it is important to highlight that feed intake could not be measured in this study, therefore no measure of protein intake is available. Meeting the protein requirements of pregnant ewes is important. The implications of a lower supply of dietary protein during gestation is particularly important as 75% of foetal growth occurs in the last 6 weeks of gestation (McCoard et al. Citation2016), thus mid-to-late gestation is a crucial window for meeting both dam and foetal nutrient demands. The substantial level of muscle degradation indicated by the reduction in eye muscle area at P135 in the FB compared with RG ewes, coupled with lower liver and kidney weight () is indicative of protein deprivation. This is further substantiated by the lower plasma urea and glucose concentrations in FB compared with RG ewes (). The lower BCS, tendency for reduced carcass fatness and perirenal fat, coupled with the elevated NEFAs at P135 () are indicative of mobilisation of fat to meet the demands of rapidly growing conceptus. Collectively, these results indicate that the FB ewes were subject to undernutrition, particularly protein deprivation, compared with RG ewes.
Total rumen SCFA concentrations were lower in FB than RG ewes in late pregnancy, as were the concentrations of acetate, caproate, isobutyrate, isovalerate and the acetate:propionate ratio, but no other treatment or treatment by time interactions were observed (). The molar proportion of acetate, isobutyrate and isovalerate were higher, and the molar proportion of propionate was lower in FB than RG ewes while the molar proportions of butyrate, caproate and valerate did not differ and no time or diet by time interactions were observed (). The concentrations of SCFA and the acetate to propionate ratios observed in this study are similar to those reported for sheep fed temperate forages and crops (e.g. Pacheco et al. Citation2016; Sun et al. Citation2019). The increase in the molar proportion and propionate and the lack of effect on butyrate is in contrast with studies with dairy cattle, in which increasing the proportion of fodder beet in a diet with pasture resulted in a decrease in propionate and an increase in butyrate proportions (Pacheco et al. Citation2020). The molar proportion of butyrate was also found to increase in the rumen fluid of dairy cows in the work of Vérité et al. (Citation1973). In these studies with dairy cattle, the increase in butyrate has been explained as a result of the increased amount of sugar in fodder beet. The lack of effect of fodder beet on butyrate in the current study is unknown and could be the result of differences in the production or removal of rumen butyrate between ruminant species. In the case of the decrease in the molar concentration of branched-chain SCFA, our results are consistent with observations in dairy cattle (Pacheco et al. Citation2020) attributable to the lower concentration of CP in the diet resulting in a reduced formation of iso-acids, which are products of the degradation of amino acids (AA) by rumen microbes (Andries et al. Citation1987).
Table 3. Rumen short-chain fatty acids (SCFA; concentrations and molar proportions: mols per 100 mols of total SCFA) at gestation d 135 (P135; n = 12/group) in twin-bearing ewes grazing dietary treatments of either fodder beet (FB) or ryegrass-dominant pasture (RG) from mid-late gestation.
From just prior to parturition to weaning, all ewes and lambs were grazed on a ryegrass-dominant pasture diet. From birth to 40 days post-partum, there was an interaction between treatment and day (P < .001), where FB ewes had a lower BW compared with RG ewes (A). Between 40 days post-partum and weaning, differences in ewe BW between treatments became smaller but were still significant (P < .001). There was a treatment by day interaction for ADG (P < .001) whereby a less marked negative ADG was followed by a positive ADG post-partum in FB compared with RG ewes (B). There continued to be a treatment by time interaction (P < .001) in ADG from 40 to 100 days post-partum. Ewes from the RG treatment had a greater increase in ADG over this period, with less of a response in the ewes from the FB treatment (B). Although BCS from 40 to 100 days post-partum was lower (P < .001) for the FB compared with RG ewes, changes in BCS were similar for both treatment groups (C). The decline in BW and BCS in the FB ewes in late gestation is consistent with the notion that their nutritional requirements (via intake or diet composition) were not able to be met by the FB regime. The recovery of LW and BCS post-partum in the FB group likely reflects the transition back to a ryegrass-based diet coupled with the higher lamb losses, the latter leading to a reduction in the nutritional demands on the dam.
In addition to total dietary CP content, AA supply is important for overall foetal growth given its role as a building block for proteins, and there are also functional AAs which play key roles in foetal growth and multiple physiological processes (McCoard et al. Citation2016). It has been identified that low-CP diets may alter the profile of circulating AA which is important as the developing uterus in sheep is specific in taking up mainly glucose and AA as sources of energy (Hopkins Citation1975). Pacheco et al. (Citation2016) reported that proportions of arginine, citrulline and ornithine in plasma of lactating cows fed fodder beet were lower than those from cows fed ryegrass, suggesting the possibility of insufficient AA supply/synthesis. Arginine, in particular, has been found to have a key role in foetal growth and development (McCoard et al. Citation2016), where parenteral supplementation to twin-bearing pregnant sheep in the last trimester increased foetal brown-fat reserves and core body temperature at birth (McCoard et al. Citation2014), increased female lamb birth weight (McCoard et al. Citation2013), placental development and function (van der Linden et al. Citation2015) and improved offspring postnatal growth rate and muscle mass (Sales et al. Citation2016). The potential for changes in specific AA to mediate, at least in part, the differences in ewe and lamb performance observed in this study warrant further investigation. It is important to note that from this grazing study, it is not possible to determine whether intake, or an imbalance/deficiency in specific diet components (e.g. CP, CP:ME ratio, specific AA etc.) drive these phenotypic responses. Such investigations need to be the subject of future studies.
Lamb performance and survival
Progeny of FB ewes had reduced survival from pregnancy scanning to weaning compared with lambs born to RG ewes, with an overall mortality of 29% vs 12% (). Compared with lambs from RG ewes, the majority of lamb losses from FB ewes was in the first 3 d after birth (). Mortality rates of lambs between parturition and weaning of twin-born lambs under New Zealand’s pastoral conditions have been reported to be within ranges of 15–32% (Kenyon et al. Citation2006), and it is well accepted that twins have a reduced birth weight leading to greater mortality rates at parturition compared with singletons (McCoard et al. Citation2017). The mortality rates of lambs that were born to ewes which grazed fodder beet in this study were similar to those recorded by Scales et al. (Citation1986) of 14.7%, and lower than 19.7% reported by Kenyon et al. (Citation2002). Mortality rates for lambs born to FB grazing ewes were almost double that of lambs born to RG grazing ewes. This finding was associated with the dams fed FB having a greater mobilisation of body reserves (fat and protein), as indicated by the reduced muscularity and subcutaneous fat in the carcasses of FB ewes. In addition, greater mortality in the FB lambs was associated with lower birth weights and frame size at birth as a consequence of in utero growth restriction (), both of which are well established to be contributing factors to lamb mortality (Scales et al. Citation1986). Furthermore, lamb rectal temperature tended to be lower in lambs from FB than RG ewes (). The Pearson Chi-square statistic for the percentage of lambs with rectal temperatures < 38.5°C was 4.30, with 1 degree of freedom, with almost twice as many lambs (15.6 vs 8.4%) having low rectal temperatures (p = .038; ). Therefore, while there was no evidence for a difference in brown fat stores at P135 (i.e. perirenal fat; ), these results indicate that FB lambs had a greater incidence of failure to thermoregulate compared with RG lambs. The biological mechanism(s) responsible remain to be established, however, failure to thermoregulate is a key contributing factor to lamb mortality (McCoard et al. Citation2017).
Table 4. Lamb mortality from scanning to birth, birth to 3 d of age, 3 d of age to docking, and from docking (i.e. tail removal at ∼40 days post-partum) to weaning (100 days post-partum), for lambs born to twin-bearing ewes grazing dietary treatments of either fodder beet or ryegrass-dominant pasture in mid-to-late gestation, and pasture only from parturition onwards with three replicates per treatment.
Table 5. Body size, rectal temperature and body weight within 12 h of birth, and body weight at docking (tail removal at ∼40 days post-partum) and weaning (100 days post-partum) for lambs born to twin-bearing ewes fed dietary treatments of either fodder beet (FB) or ryegrass-dominant pasture (RG) from mid-to-late gestation, and then pasture only from parturition onwards.
Table 6. Selected plasma metabolite concentrations from foetuses carried by twin-bearing ewes grazing dietary treatments of either fodder beet (FB) or ryegrass-dominant pasture (RG) at gestation d 135 (P135; n = 12/group).
There was no evidence of disproportionate growth of the foetal organs (i.e. organ weight as a percentage of BW; data not shown). However, the lower birth weight of the FB lambs was associated with reduced skeletal muscle and kidney weight () which is consistent with in-utero undernutrition. During foetal development, nutrient partitioning for skeletal muscle growth has a lower priority compared with other tissues such as brain, heart and liver, and is thus more vulnerable to nutrient deficiency (Zhu et al. Citation2006). As no other organ weights beyond the kidney and skeletal muscle were affected by treatment, it is likely that the nutrient deprivation was sufficient to restrict muscle growth and overall foetal growth, but not severe enough to cause restricted growth of other organs. The lower plasma urea concentrations in the foetuses from FB compared with RG ewes, but no differences in total protein, glucose, NEFA or triglycerides (), also suggests that protein deprivation may be a key limiting factor which warrants further study.
Lambs born to FB compared with RG ewes had reduced postnatal growth rates to weaning, even after accounting for differences in birth weight (). Possible explanations for this include reduced dam lactation performance because of undernutrition during gestation and/or early lactation (Tygesen et al. Citation2008), and/or reduced capacity for muscle growth as a result of growth restriction in utero (Greenwood et al. Citation2000; McCoard et al. Citation2010; Sales et al. Citation2014). Reduced skeletal muscle mass in foetuses who have experienced growth restricted in utero, can contribute to increased metabolic disease risk (Rozance et al. Citation2018). Further investigations into the long-term impacts of a FB grazing regime in ewes during pregnancy on their progeny, particularly those destined to become breeding animals, is warranted.
Conclusions and applications
In this study, FB was a high yielding forage crop option in winter with an estimated DM yield of about 30 t DM/ha, thus able to fill pasture deficits. However, outcomes of this study have indicated that a grazing regime based on FB compared with traditional grass-based pasture for twin-bearing ewes in late gestation has detrimental consequences on dam and offspring performance phenotypes, notably ewe body condition and foetal muscle and overall body growth, birth weight, lamb survival and postnatal growth. Although this study identifies potential risks of FB as a winter feed option grazed in situ for pregnant animals and their offspring, key drivers of the phenotypic responses (i.e. DMI, protein and/or AA deficiencies) and options around mitigating these risks are needed to address potential FB nutritional shortcomings in grazing situations are required. The new knowledge generated in this study will contribute to further research into the influence of maternal nutrition on foetal growth, survival and postnatal performance, and future development of feeding recommendations and better winter grazing management decisions on-farm to minimise adverse effects on dam and offspring performance.
Acknowledgements
This research was funded by AgResearch’s Strategic Science Investment Fund and Beef + Lamb New Zealand. The authors would also like to thank AgResearch’s Aorangi Farm Staff and the Animal Nutrition and Physiology Team for their assistance with the animal trial and sample collection and analysis, and Dr Siva Ganesh for assistance with statistical analysis.
Disclosure statement
No potential conflict of interest was reported by the author(s).
Additional information
Funding
References
- Andries JI, Buysse FX, De Brabander DL, Cottyn BG. 1987. Isoacids in ruminant nutrition: their role in ruminal and intermediary metabolism and possible influences on performances – a review. Animal Feed Science and Technology. 18:169–180.
- AOAC. 1990. Official methods of analysis. 15th ed. Arlington (VA): AOAC.
- AOAC. 2005. Official method of analysis. 18th ed. Gaithersburg (MD): AOAC.
- Clark P, Givens DE, Brunnen JM. 1987. The chemical composition, digestibility and energy value of fodder-beet roots. Animal Feed Science and Technology. 18:225–231.
- Dalley DE, Geddes T. 2012. Pasture growth and quality on Southland and Otago dairy farms. Procedures of NZ Grassland Association. 74:237–241.
- Gibbs SJ, Saldias B. 2014. Fodder beet in the New Zealand dairy industry. Conference Proceedings of the South Island Dairy Event, Invercargill, Lincoln University.
- Gibbs SJ, Saldias B, White J, Walsh D, Stocker N, Trotter C, Fisher B, Fisher A, Banks B, Hodge S. 2015. A comparison of liveweight gain of two groups of weaners of different entry liveweight in an ad libitum fodder beet feeding system for finishing beef cattle. Journal of New Zealand Grasslands. 77:23–28.
- Greenwood PL, Slepetis RM, Bell AW, Hermanson JW. 2000. Intrauterine growth retardation is associated with reduced cell cycle activity, but not myofibre number, in ovine fetal muscle. Reproduction Fertility and Development. 11:281–291.
- Hammond KJ, Burke JL, Koolaard JP, Muetzel S, Pinares-Patino CS, Waghorn GC. 2013. Effects of feed intake on enteric methane emission from sheep fed fresh white clover (Trifolium repens) and perennial ryegrass (Lolium perenne) forages. Animal Feed Science and Technology. 179:121–132.
- Hopkins PS. 1975. The development of the foetal ruminant. In: McDonald IW, Warner ACI, editor. Digestion and Metabolism in the ruminant. Armidale: University of New England Publishing Unit; p. 1–14.
- Jefferies B. 1961. Body condition scoring and its use in management. Tasmanian Journal of Agriculture. 32:19–21.
- Kenyon P, Morris S, McCutcheon S. 2002. Does an increase in lamb birth weight through mid-pregnancy shearing necessarily mean an increase in lamb survival rates to weaning? Proceedings of NZ Society of Animal Production. 62:53–56.
- Kenyon PR, Revell DK, Morris ST. 2006. Mid-pregnancy shearing can increase birthweight and survival to weaning of multiple-born lambs under commercial conditions. Australian Journal of Experimental Agriculture. 46:821–825.
- Kenyon PR, Webby RW. 2007. Pastures and supplements in sheep production systems. In: Rattray P, Brookes I, Nicol A, editors. Pasture and supplements for grazing animals. Hamilton: New Zealand Society of Animal Production; p. 255–274.
- McCoard SA, Cristobal-Carballo O, Knol FW, Heiser A, Khan A, Hennes N, Johnstone P, Lewis S, Stevens DR. 2019. Impact of early weaning on small intestine, metabolic, immune and endocrine system development, growth and body composition in artificially reared lambs. Journal of Animal Science. 98(1):skz356. doi:https://doi.org/10.1093/jas/skz356.
- McCoard SA, Koolaard J, Charteris A, Luo D. 2010. Effect of twinning and sex on carcass weight and composition in lambs. Proceeds of NZ Society of Animal Production. 70:133–136.
- McCoard SA, Sales FA, Sciascia QL. 2016. Amino acids in sheep production. Frontiers in Bioscience. 1:264–288.
- McCoard SA, Sales FA, Sciascia QL. 2017. Invited review: impact of specific nutrient interventions during mid-to-late gestation on physiological traits important for survival of multiple-born lambs. Animal. 22:1–10. doi:https://doi.org/10.1017/S1751731117000313.
- McCoard SA, Sales F, Wards N, Sciascia Q, Oliver MH, Koolaard J, van der Linden DS. 2013. Parenteral administration of twin-bearing ewes with L-arginine enhances the birth weight and brown fat stores in sheep. SpringerPlus. 2:1–12.
- McCoard S, Wards N, Koolaard J, Salerno MS. 2014. The effect of maternal arginine supplementation on the development of the thermogenic program in the ovine fetus. Animal Production Science. 54:1843–1847.
- Milne GD, Direen C, Kitson E, Evans P, Cleland RG, Treder D. 2014. Performance of fodder beet cultivars in the South Island. Proceeds of NZ Grasslands Association. 76:141–144.
- Morris ST, Kenyon PR. 2004. The effect of litter size and sward height on ewe and lamb performance. NZ Journal Agricultural Research. 47:275–286.
- National Research Council. 2007. Nutrient requirements of small Ruminants: sheep, Goats, Cervids, and new World Camelids. Washington (DC): The National Academies Press.
- Pacheco D, Muetzel S, Lewis S, Dalley D, Bryant M, Waghorn GC. 2020. Rumen digesta and products of fermentation in cows fed varying proportions of fodder beet (Beta vulgaris) with fresh pasture or silage or straw. Animal Production Science. 60:):524–534. doi:AN18002.
- Pacheco D, Waghorn GC, Dalley D. 2016. Brief Communication: plasma amino acid profiles of lactating dairy cows fed fodder beet and ryegrass diets. Proceeds of NZ Society of Animal Production. 76:62–64.
- R Core Team. 2016. R: A language and environment for statistical computing. Vienna, Austria: R Foundation for Statistical Computing.
- Robertson JB, Van Soest PJ. 1981. The detergent system of analysis and its application to human foods. In: James W, Theander O, editor. The analysis of dietary fiber in Food No. 3. New York: Marcel Dekker Inc; p. 123–158.
- Roughan PG, Holland R. 1977. Predicting in vivo digestibilities of herbages by exhaustive enzyme hydrolysis of cell walls. Journal of Science Food and Agriculture. 28:1057–1064.
- Rozance PJ, Zastoupil L, Wesolowski SR, Goldstrohm DA, Strahan B, Cree-Green M, Sheffield-Moore M, Meschia G, Jr HW, Wilkening RG, Brown LD. 2018. Skeletal muscle protein accretion rates and hindlimb growth are reduced in late gestation intrauterine growth-restricted sheep. Journal of Physiology. 596(1):67–82. doi:https://doi.org/10.1113/JPx275230.
- Sales FA, Pacheco D, Blair HT, Kenyon PR, Nicholas G, Senna Salerno M, McCoard SA. 2014. Identification of amino acids associated with skeletal muscle growth in late gestation and at weaning in lambs of well-nourished sheep. Journal Animal Science. 92:5041–5052.
- Sales FA, Sciascia Q, Van der Linden DS, Wards N, Oliver MH, McCoard SA. 2016. Intravenous maternal l-arginine administration to twin-bearing ewes, during late pregnancy, is associated with increased fetal muscle mTOR abundance and postnatal growth in twin female lambs. Journal of Animal Science. 94:2519–2531.
- SCA. 1990. Australian agricultural Council Ruminants Subcommittee – feeding standards for Australian livestock: Ruminants. Melbourne: CSIRO Publications, 266 p.
- Scales GH, Burton RN, Moss RA. 1986. Lamb mortality, birthweight, and nutrition in late pregnancy. NZ Journal Agricultural Research. 29:75–82.
- Stevens DR. 1999. Ewe nutrition: decisions to be made with scanning information. Proceeds of NZ Society of Animal Production. 59:93–94.
- Sun X, Chen A, Pacheco D, Hoskin SW, Luo D. 2019. Sheep rumen fermentation characteristics affected by feeding frequency and feeding level when fed fresh forage. Animals. 10:7. doi:https://doi.org/10.3390/ani10010007.
- Tavendale MH, Meagher LP, Pacheco D, Walker N, Attwood GT, Sivakumaran S. 2005. Methane production from in vitro rumen incubations with Lotus pedunculatus and Medicago sativa, and effects of extractable condensed tannin fractions on methanogenesis. Animal Feed Science and Technology. 123-124:403–419.
- Tygesen MP, Nielsen MO, Nørgaard P, Ranvig H, Harrison AP, Tauson AH. 2008. Late gestational nutrient restriction: effects on ewes’ metabolic and homeorhetic adaptation, consequences for lamb birth weight and lactation performance. Archives Animal Nutrition. 62:44–59.
- van der Linden DS, Sciascia Q, Sales F, Wards NJ, Oliver MH, McCoard SA. 2015. Intravenous maternal L-arginine administration to twin-bearing ewes during late pregnancy enhances placental growth and development. Journal of Animal Science. 93:4917–4925.
- Vérité R, Journet M, Fléchet J, Lefaivre R, Lefaivre J, Ollier A. 1973. Utilisation de quantites elevees de betteraves par les vaches laitieres: Etude de l'ingestion, de la digestion et des effets sur la production. Annales de Zootechnie. 22(2):219–235.
- VSN International. 2015. Genstat for Windows 18th edition. Hemel Hempstead: VSN International.
- Waghorn GC, Burke JL, Kolver ES. 2007. Principles of feeding value. In: P. Rattray, I. Brookes, A. Nicol, editor. Pasture and supplements for grazing animals. Hamilton: New Zealand Society of Animal Production; p. 35–60.
- Zhu MJ, Ford SP, Means WJ, Hess BW, Nathanielsz PW, Du M. 2006. Maternal nutrient restriction affects properties of skeletal muscle in offspring. Journal of Physiology. 575:241–250.