Abstract
Ricinus is an invasive species. We assessed its responses to intra- and interspecific competition, and different intensities and spectral qualities of light. To evaluate resource portioning, we obtained biomass measurements of juveniles in different densities of co-specifics and another species; we also compared the proportion of juveniles to determine if there is recruitment limitation. We obtained the same information for juveniles growing in different photosynthetic photon flux densities (PPFD) and ratios of red to far-red wavelengths (R/FR). Competition and light did not limit recruitment, resulting in differences in biomass allocation only. Biomass decreased more for plants subjected to interspecific competition than to intraspecific competition, suggesting that Ricinus is both a poor intra- and interspecific competitor. Juveniles growing under reduced PPFD and R/FR differed in biomass allocation and morphology as do shade-intolerant species subjected to shading. Our results show that Ricinus presents high plasticity, which may contribute to its invasion ability.
Introduction
Invasions by alien species are a global phenomenon that can cause irreversible changes in community structure and ecosystem function (Levine et al. Citation2003). Ricinus communis L. (Euphorbiaceae), commonly known as castor or castor bean, is a shrub or small tree distributed worldwide as a highly invasive species. In Brazil, it is commonly observed in disturbed environments, such as waste heaps, roadsides, forest edges and urban vacant lots. Ricinus colonizes these areas as soon as they are ploughed or exposed to other anthropogenic pressures. In urban scenarios, the species dominates many vacant lots, where it commonly occurs in monospecific stands surrounded by another exotic plant, Brachiaria decumbens Stapf. (Poaceae; Martins, Guimarães et al. 2009; Martins, Haddad & Semir 2009).
Martins et al. (2006) have shown that Ricinus seeds are dispersed by ants, which are attracted to their lipid-rich elaiosome. Ants carry the seeds into their nest, eat the elaiosome, and discard the seeds, usually in a viable condition, onto pile mounds. As a result, more Ricinus seeds without the elaiosome and seedlings can be found in pile mounds than in their vicinities. Therefore, seedlings may experience high competition (Martins, Guimarães et al. 2009), which is considered to be one of the main factors controlling plant growth processes and is crucial for seedling establishment (Lee et al. Citation1996; Whitmore Citation1996). The clumped spatial pattern that persists from the seedling to the adult stage of Ricinus (Martins, Guimarães et al. 2009; Martins, Haddad & Semir 2009) may be an outcome of either a poor or strong intraspecific competition ability of this species. If it is a poor intraspecific competitor, each individual should use relatively low quantities of resources, which are divided among the population; in this case, we hypothesize that such a low rate in the use of resources supports very high-density stands of individuals with similar biomass allocation to roots, stems and leaves when compared with individuals growing in low-density stands. By contrast, if Ricinus is a strong intraspecific competitor, each individual should use relatively high quantities of resources, which are divided among the population until they are depleted; in this case, we hypothesize that resource depletion results in individuals with less biomass in roots, stems and leaves when compared with individuals growing in low-density stands; ultimately, resource depletion may also lead to self-thinning, so that mortality is expected to be disproportionally higher in very dense stands (Sterner et al. Citation1986).
Ricinus seeds that do not germinate immediately after dispersal are probably incorporated into the soil seed bank (Martins, Haddad & Semir 2009). Seeds that make up soil seed banks usually respond to external environmental cues, such as alternating temperatures and/or presence of light, so that germination occurs at the appropriate time (Pons Citation1993; Probert Citation1993). Because seed banks are usually constituted of seeds of more than one species (Garwood Citation1989), the environmental cues that lead Ricinus to germinate probably result in the germination of other species as well. This means that the emerged Ricinus seedlings are subjected not only to intraspecific competition, but also to high competition with other plant species. Because Ricinus adults occur in monospecific stands (Martins, Guimarães et al. 2009; Martins, Haddad & Semir 2009), we hypothesize that this species acts as a strong interspecific competitor: its capacity to use disproportionately high quantities of resources when compared with plants of other species leads to the exclusion of the other species from the stand. The strong interspecific competition ability of Ricinus plants should be verified by small differences in both biomass allocation and number of established plants growing at different densities of a potential competitor (e.g. B. decumbens).
The occurrence of Ricinus adults in clumps surrounded by B. decumbens may result from a balance between the responses of this species as an intra- and interspecific competitor. It is possible to determine whether the intraspecific competition ability of Ricinus is weaker or stronger than its interspecific competition ability by directly comparing biomass allocation of Ricinus plants growing with co-specifics only, and with co-specifics as well as B. decumbens. If Ricinus plants growing with co-specifics only allocate more biomass to roots, stems and leaves, one can infer that this species is a stronger intraspecific competitor than it is an interspecific competitor. By contrast, if Ricinus plants growing with co-specifics as well as B. decumbens allocate more biomass to roots, stems and leaves, one can infer that it is a stronger interspecific competitor than it is an intraspecific competitor.
The alternating temperatures and/or presence of light that trigger germination of seeds in seed banks are commonly established after soil disturbance (Pons Citation1993; Probert Citation1993), which generally coincides with complete destruction of the established vegetation. This leads to a high exposure of emerged seedlings to sunlight, as observed by Martins, Haddad & Semir (2009) for Ricinus. The survival and rapid growth of Ricinus seedlings when exposed to sunlight, together with the wide distribution of the species in disturbed environments, indicate that it most likely is a shade-intolerant species. However, Ricinus responses to different light availability have never been explored and might be important for understanding its invasion success (Burns & Winn Citation2006). If this species presents phenotypic plasticity, i.e. differences in morphological or physiological traits when grown under different environmental conditions (Ryser & Eek Citation2000), it may optimize its capacity to acquire the most limiting resource (e.g. light) and hence maximize growth in a wider range of habitats when compared with less plastic species (Latham Citation1992; Valladares et al. Citation2000). Therefore, plastic responses may be one way for invasive species to succeed over a wide range of environmental conditions and thus gain an advantage over native species (e.g. Fogarty & Facelli Citation1999; Stratton & Goldstein Citation2001).
Phenotypic plasticity in the responses to light environment can occur when the intensity and quality of light to which plants are subjected are altered (Dale & Causton Citation1992; Lee et al. Citation1996). The intensity of radiation, and consequently of the photosynthetic photon flux density (PPFD), is decreased by passage through foliage, surface reflectance and penumbral effects. Leaf absorption also alters the quality of light, or spectral distribution (Lee et al. Citation1996), particularly the ratio of red to far-red wavelengths (R/FR; i.e. flux density at 655–665 nm/flux density at 725–735 nm; Holmes & Smith Citation1977).
Shade-intolerant species respond to shading by allocating more resources to leaves and stems. More and wider leaves probably enhance photosynthesis in the shade until the plant reaches increased light (van Hinsberg & van Tienderen Citation1997). By contrast, the mobilization of carbohydrate for stem elongation to a greater degree than do shade species (Smith Citation1982) makes it possible for shade-intolerants to overgrow smaller competitors in the short-term (Dale & Causton Citation1992). Therefore, they enhance light interception in tall vegetation by placing the photosynthetically active plant parts in the higher vegetation strata (Smith Citation1982; van Hinsberg & van Tienderen 1997). Moreover, transference of biomass from roots to stem in shade-intolerant species subjected to shading may also contribute to carbohydrate conservation because root respiration generally exceeds that of stem tissue (Corré Citation1983).
However, the exclusive effects of R/FR on plant total biomass vary among species, and positive, negative and no effect whatsoever on growth rate have been reported (e.g. Lee et al. Citation1996; van Hinsberg & van Tienderen 1997). The most remarkable feature of shade-intolerant species subjected to lower R/FR are morphological alterations such as reduced branching and increased stem elongation (Dale & Causton Citation1992; van Hinsberg & van Tienderen 1997), which illustrate their capacity to adapt to shade (Corré Citation1983)
Seed dispersal and germinatio n, and early seedling growth/survival are considered important processes for plant population regeneration (Herrera et al. Citation1994; Schupp Citation1995). Although seed dispersal and germination of the invasive Ricinus have been thoroughly studied (e.g. Lagôa & Pereira Citation1987; Bianchini & Pacini Citation1996; Lisci et al. Citation1996; Martins et al. Citation2006; Martins, Guimarães et al. 2009; Martins, Haddad & Semir 2009), there is no information, to our knowledge, about this species’ recruitment process. A single mechanism is unlikely to underlie invasiveness, but some common features may promote success as an invader, and knowledge of these features could help to identify potential invaders and limit their negative effects (Burns & Winn Citation2006). This study was designed to assess the responses of Ricinus seedlings to intra- and interspecific competition to a common co-occurring species (B. decumbens; Martins, Guimarães et al. 2009), and to different intensities and spectral qualities of light. Specifically, we obtained several biomass measurements of recruited seedlings (hereafter, called juveniles) to determine: 1. if Ricinus is either a poor or a strong intraspecific competitor, 2. if it is a strong interspecific competitor, 3. if it is a stronger or weaker intraspecific competitor than it is an interspecific competitor, and 4. if it presents high phenotypic plasticity in its shade-intolerant responses to different light environments.
Materials and methods
Species studied
Ricinus communis L. (Euphorbiaceae) originated in Africa and has been introduced to all continents as a crop for oil extraction. The species is monoecious and produces fruits that are an explosive capsule with three seeds, which bear a lipid-rich elaiosome (Singh Citation1976; Mabberley Citation1998). The seeds are primarily dispersed by autochory and secondarily dispersed by ants (despite the relative enormous seed weight of c. 0.123 g; Martins et al. Citation2006; Martins, Guimarães et al. 2009). In Brazil, this interaction is extremely conspicuous everywhere the plant occurs (Martins, Guimarães et al. 2009; Martins, Haddad & Semir 2009). Ricinus seeds germinate in a wide range of age, elaiosome, temperature and light conditions (Martins et al. Citation2006; Martins, Haddad & Semir 2009), and this generalist germination behaviour may be a key feature for the invasion ability of this species (Martins, Haddad & Semir 2009). Ricinus flowers all year long and each plant produces between 1500 and 150,000 seeds per reproductive period (Hogan Citation1992). It is also capable of early maturity, and rapid growth and recovery after disturbance (Martins, Guimarães et al. 2009; Martins, Haddad & Semir 2009).
Seed collection and experiment sites
We collected Ricinus seeds from 10 parent plants. We wrapped two or three large infructescences of each plant with tulle bags, which were removed when all fruits had ripened. This procedure prevents seeds from being thrown away from the parent plants due to autochory. The collections were performed in vacant lots, i.e. open areas with no considerable shady sites located in urban scenarios, in the municipality of Campinas, southeast Brazil (22°54′S, 47°03′W). The climate is Koeppen's Cwa, macrothermal temperate with mild dry winter (Camargo et al. Citation1966). The study sites were dominated by Ricinus, which occur in monospecific stands surrounded by the also invasive Brachiaria decumbens Stapf. (Poaceae; Martins, Guimarães et al. 2009; Martins, Haddad & Semir Citation2009).
The experiments to obtain juvenile biomass in intra- and interspecific competition, and in different intensities and spectral qualities of light were carried out in an open area at the State University of Campinas. This is an area of c. 10 × 21 m with many tables of 0.8 × 3 m where pots can be laid. These tables are under natural conditions, exposed to sunlight and mechanically watered four times a day. We believe that the temperature, humidity and light regime at the experiment site were very similar to those found by natural populations of Ricinus, because the distance from the seed-collection sites and the experiment site was no greater than 8 km. Moreover, the table tops are made of a loose mesh that does not increase the temperature of the pots.
Juvenile biomass in intra- and interspecific competition
To analyse Ricinus intraspecific competition response, we sowed freshly harvested seeds without elaiosome in pots at four different densities: 1, 4, 16 and 64 seeds/pot. The elaiosomes were removed in order to mimic as best as possible how these seeds are dispersed in nature and also to prevent pathogen infestation, which could lead to the death of the seeds. The elaiosomes were manually removed, which enabled them to be fully detached from the seeds without damaging the seed coat (Martins, Guimarães et al. 2009; Martins, Haddad & Semir 2009).
In order to analyse Ricinus interspecific competition response, we performed an experiment using B. decumbens as a model for interspecific competition, because this species is commonly observed co-occurring with Ricinus in vacant lots (Martins, Guimarães et al. 2009). It is true that Ricinus also competes with other plant species, but usually they occur as very few individuals in a dominant community of Ricinus and B. decumbens (V. Martins, pers. obs.). Therefore, we believe that B. decumbens is the commonest competitor of Ricinus in disturbed areas of southeast Brazil.
We bought B. decumbens seeds in a specialized store and had them germinated inside a germination cabinet after chemical and manual scarification. After some trials, the highest germination percentage of B. decumbens was obtained inside a cabinet with four 20 W fluorescent regular tube lights and alternating temperatures of 20 and 30 °C. We also adjusted the cabinet for uninterrupted 12 h light and 12 h darkness to simulate day and night periods; the dark period coincided with the 20 °C period. Brachiaria decumbens seeds were kept inside the cabinet for one week, after which most of them had germinated. Then we sowed B. decumbens young plants and freshly harvested Ricinus seeds without elaiosome (manually removed) in a 1:1 ratio in pots at three different densities: 4, 16 and 64 individuals in total/pot. The Ricinus seeds were sowed only after the B. decumbens plants were established. This was done in order to simulate the conditions observed in vacant lots, where B.cdecumbens is the first colonizing species after disturbance (V. Martins, pers. obs.).
For both intra- and interspecific competition experiments, Ricinus seeds and B. decumbens plants were equidistantly placed at 1 cm depth in pots of the same volume (5 L) and filled with a similar quantity of soil. Each pot had a girth of c. 25 cm, so seeds (and B. decumbens plants in the interspecific competition experiment) were at least 2 cm apart. There were ten pots for each density treatment in the experiments. Pots were consecutively laid on the tables following this order: density treatments of the intraspecific experiment and density treatments of the interspecific experiment. We checked the pots every three days for a three-month period, when we recorded the number of Ricinus juveniles. Ambient temperature variation was 15.3–36.9 °C in exposed sunlight between mid December 2005 and mid March 2006, when the experiments were carried out.
We then performed biomass measurements of all Ricinus plants that had lost their first leaves (hence, considered as recruited seedlings, or juveniles) at least one week before the measurements. By allowing this time, we assured that seed reserves were exhausted and that plants were only relying on photosynthesis in order to obtain energy. We tried to obtain as many measurements as possible in order to assess resource portioning in plants submitted to different degrees of competition. We gently removed all the content of the pots and washed away the soil from the roots, whose growth apparently was not strongly limited by the pot dimensions during the experiment period. We separated each plant in three parts: roots, stems plus leaf petioles (hereafter called just stems) and leaf blades (hereafter called just leaves). We measured the root length and stem length with a millimetric ruler, counted the number of leaves and obtained the leaf area with a leaf area meter (LI-COR, Inc., model LI-3100, Lincoln, Nebraska, USA). Roots, stems, and leaves were dehydrated in an 80 °C cabinet for more than two days, after which we obtained the dry masses of roots (DM root), stems (DM stem) and leaves (DM leaf) using an analytical scale. We calculated total dry mass (DM total), root mass ratio (RMR), root/aerial system ratio (R/AS), stem mass ratio (SMR), stem robustness (ROB), specific leaf mass (SLM), leaf mass ratio (LMR) and leaf area ratio (LAR) as follows (Hunt Citation1982; Lee et al. Citation1996):
Juvenile biomass in different intensities and spectral qualities of light
In order to analyse Ricinus response to intensities and spectral qualities of light, we performed an experiment in which we manipulated the PPFD and R/FR of natural incident sunlight. We sowed four freshly harvested Ricinus seeds without elaiosome (manually removed) in pots filled with soil. In order to decrease PPFD, we used one to three layers of fine plastic mesh (three treatments) suspended on an iron frame above the tables; to alter R/FR, we used a purple cloth made of 100% synthetic fabric, whose pigmentation absorbs red light but not far-red radiation, thus reducing R/FR (only one treatment). The purple cloth was also suspended on an iron frame above the table. Seeds in control pots were not covered with any kind of layer, so that the alteration in intensity or spectral quality of light would be minor (Sanches Citation2004). The control treatment was the same for the PPFD treatments and the R/FR treatment. Pots of each treatment (control, the three PPFD treatments and the R/FR treatment) were laid on a different table.
We performed PPFD measurements at all the experimental treatments, using a radiation linear sensor (Line Quantum Sensor, model LI 191 AS) coupled to a datalogger (model LI 1000). We also measured red and far-red radiations in the control and purple cloth treatments, using a radiation sensor (650/730 nm, Skye Instruments; ). All measurements were performed in spring on a bright sunny day, around noon. Besides changing intensity and spectral quality of light, the plastic meshes and purple cloth may also have altered temperature and humidity in the different treatments, as one would expect to occur under canopies in natural systems.
Table 1 Photosynthetic photons flux density (PPFD) and ratio of red to far-red wavelengths (R/FR) at five treatments: zero to three layers of fine plastic mesh and a common purple cloth sustained above pots containing freshly harvested seeds of Ricinus communis without elaiosome (manually removed).
The Ricinus seeds were placed equidistantly at 1 cm depth in pots of same volume (5 L) and size (25 cm of girth). The pots were filled with a similar quantity of soil and there were 10 pots for each light treatment. We checked the experiment every 3 days for a 51-day period, when we recorded the number of Ricinus juveniles. During the experiment in October and November 2005, ambient temperature variation was 13.8–36.9 °C in exposed sunlight.
At the end of this experiment, we performed biomass measurements exactly as described in the previous section. They enabled us to determine how R/AS, number of leaves, leaf area and biomass of Ricinus juveniles were altered as PPFD and R/FR were reduced.
Statistical analyses
To compare the proportion of Ricinus juveniles among density treatments in the intraspecific competition experiment and in the interspecific competition experiment, we used Kruskal–Wallis tests. The proportion of Ricinus juveniles was calculated as the number of juveniles per pot by the end of the experiments divided by the number of Ricinus seeds originally sowed in each pot. To compare the proportion of juveniles between the same density treatment in the intra- and interspecific experiments, we used Mann–Whitney tests. For statistical analyses, we transformed the proportions of juveniles per pot into the arcsine of the square root of the proportion (Zar Citation1999). Instead of controlling the number of Ricinus plants per pot by selective weeding, we chose to use seedling recruitment as a response variable in order to assess how intra- and interspecific competition alters the number of individuals of this species in a given area.
For analysis of the biomass measurements obtained from Ricinus juveniles in the competition experiments, we disregarded the original density treatments and used the number of Ricinus juveniles per pot for the intraspecific competition experiment, and the number of Ricinus juveniles plus the number of established B. decumbens plants per pot for the interspecific experiment. This was done because we obtained a highly variable number of Ricinus juveniles per pot of the same density treatment in both experiments. Therefore, we had no or just a few replicates of the same density treatment to perform a categorical analysis.
To compare the biomass measurements obtained from Ricinus juveniles in the intra- and interspecific competition experiments, we used correlation analyses. The independent variable was the number of plants per pot and the dependent variable was the mean biomass per pot of Ricinus juveniles growing at each density. We performed a correlation analysis for each of the biomass measurements.
In order to compare the biomass measurements of Ricinus juveniles growing in pots with co-specifics only or in pots with co-specifics and B. decumbens, we created two categories: 1. four or fewer established plants per pot (either Ricinus juveniles in the intraspecific competition experiment or Ricinus juveniles plus B. decumbens plants in the interspecific competition experiment); and 2. five or more established plants per pot. We then compared both categories between the intra- and interspecific experiments using a t-test or Mann–Whitney test when data showed no normality (Zar Citation1999). The cut-off between four and five plants per pot was necessary in order to have similar sample sizes in the two density categories. We were not able to use more density categories (i.e. low, medium and high density) because there would have been only a small number of plants for comparison.
To compare the number of Ricinus juveniles growing under different PPFD, we used a Kruskal–Wallis test. To compare the number of Ricinus juveniles growing under different R/FR, we used a Mann–Whitney test (Zar Citation1999).
To compare the biomass measurements among Ricinus juveniles growing under different PPFD, we used analyses of variance (Bonferroni for ad hoc tests) or Kruskal–Wallis tests (Mann–Whitney for a posteriori tests) when data showed no normality. To compare the biomass measurements between juveniles growing in the control treatment and in the purple cloth treatment, we used t-tests or Mann–Whitney tests when data showed no normality (Zar 1999). We used an alpha level of 5% for every statistical analysis.
Results
Juvenile biomass in intra- and interspecific competition
In the intraspecific competition experiment, we found no difference in the proportion of Ricinus juveniles among density treatments (P>0.05). However, there was a decrease in the number of leaves with increasing number of juveniles per pot (F-ratio=2.52, Sum of squares=15.16, P=0.031), whereas SMR increased with increasing number of Ricinus juveniles per pot (F-ratio=3.23, Sum of squares=0.10, P=0.010; ). The other biomass measurements did not differ among density treatments in the intraspecific competition experiment (P>0.05).
Figure 1 Leaves and stem mass rations of Ricinus communis juveniles growing in pots at densities of 1–57 plants per pot. A, Number of leaves (No. leaves; r 2 = 0.26). B, Stem mass ratio (SMR; r 2 = 0.08) Each point represents the mean value per pot of the two variables measured.
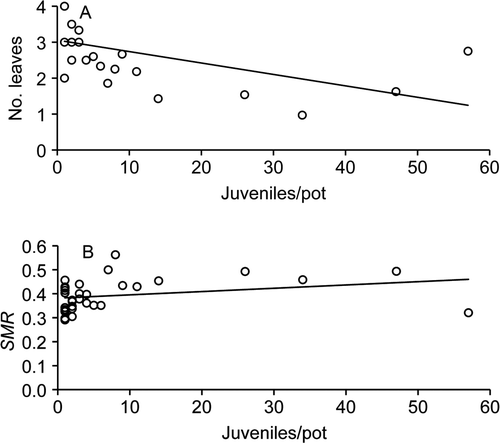
In the interspecific competition experiment, we also found no difference in the proportion of Ricinus juveniles among density treatments (P>0.05). However, there was an increase in DM root (F-ratio=14.88, Sum of squares=0.03, P<0.001), DM total (F-ratio=4.61, Sum of squares=0.03, P=0.014), RMR (F-ratio=8.30, Sum of squares=0.52, P=0.002) and R/AS (F-ratio=60.95, Sum of squares=36.90, P<0.001) with increasing number of plants per pot, whereas there was a decrease in DM stem (F-ratio=3.32, Sum of squares=0.01, P=0.04) and SMR (F-ratio=4.66, Sum of squares=0.42, P=0.015) with increasing number of plants per pot (). The other biomass measurements did not differ among density treatments in the interspecific competition experiment (P>0.05).
Figure 2 Root and stem masses of Ricinus communis juveniles growing with Brachiaria decumbens in pots at densities of 3–53 plants per pot. A, Root dry mass (DM root; r 2 = 0.04). B, Stem dry mass (DM stem; r 2 = 0.11). C, Total dry mass (DM total; r 2 = 0.00). D, Root mass ratio (RMR; r 2 = 0.02). E, Root/aerial system ratio (R/AS; r 2 = 0.09). F, Stem mass ratio (SMR; r 2 = 0.08). Each point represents the mean value per pot of the variables measured in Ricinus juveniles.
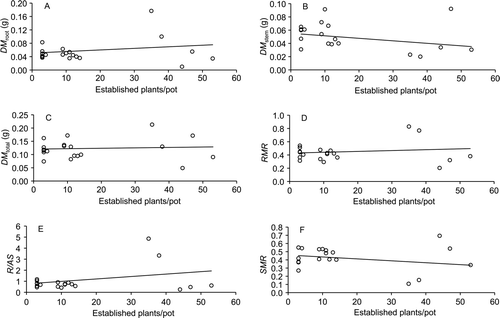
There was no difference in the proportion of Ricinus juveniles between the same density treatment in the intra- and interspecific competition experiments (P>0.05). Juveniles growing in pots with four or fewer plants and in pots with five or more plants had greater root length, stem length, leaf area, DM root, DM stem, DM leaf, DM total, ROB, LMR and LAR in the intraspecific competition experiment than in the interspecific competition experiment. Number of leaves was greater for juveniles growing in pots with four or fewer plants in the intraspecific competition experiment than in the interspecific competition experiment; however, it was similar for juveniles growing in pots with five or more plants in both experiments. RMR, R/AS and SMR were greater for juveniles growing in pots with four or fewer plants in the interspecific competition experiment than in the intraspecific competition experiment; however, they were similar for juveniles growing in pots with five or more plants in both experiments (). There was no difference in SLM for juveniles growing in both intra- and interspecific experiments (P>0.05).
Table 2 Means (±SD, when data showed normality) or medians (with confidence interval of 95%, when data showed no normality) of biomass measurements of Ricinus communis juveniles growing in pots with co-specifics only (Intra) and Ricinus juveniles growing in pots with Brachiaria decumbens (Inter) at two plant density categories: 1. four or fewer established plants per pot, and 2. five or more established plants per pot.
Juvenile biomass in different intensities and spectral qualities of light
There was no difference in the number of Ricinus juveniles growing under different PPFD and R/FR (P>0.05). There was a tendency to increase the root length, DM root, DM stem, RMR, R/AS, ROB and SLM with increasing light intensity. However, there was a tendency to decrease the stem length, number of leaves, leaf area, DM total, SMR, LMR and LAR with increasing light intensity (P ≤ 0.05; ). DM leaf did not differ among Ricinus juveniles growing under different PPFD (P>0.05).
Figure 3 Means (± SD, when data showed normality) or medians (with confidence interval of 95%, when data showed no normality) of biomass measurements of Ricinus communis juveniles growing under different photosynthetic photon flux densities (PPFD). Each point was calculated with the mean value per pot of the variables measured in Ricinus juveniles growing in 10 pots at each light treatment. No. leaves, number of leaves; DM root, root dry mass; DM stem, stem dry mass; DM total, total dry mass; RMR, root mass ratio; R/AS, root/aerial system ratio; SMR, stem mass ratio; ROB, stem robustness; SLM, specific leaf mass; LMR, leaf mass ratio; LAR, leaf area ratio. Same numbers represent statistically similar groups (P>0.05) and different numbers represent statistically distinct groups (P ≤ 0.05).
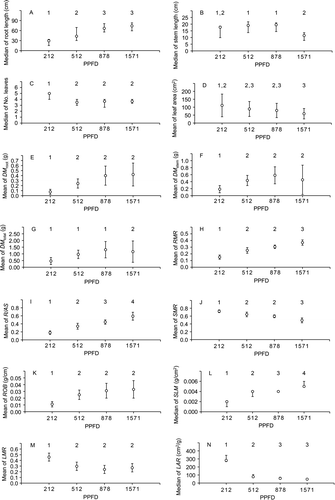
Ricinus juveniles in the control treatment had greater root length (t=6.76, d.f.=18, P<0.001), DM root (t=5.43, d.f.=18, P<0.001), RMR (t=11.22, d.f.=18, P<0.001), R/AS (t=10.63, d.f.=18, P<0.001), ROB (U=92.00, d.f.=1, P=0.001) and SLM (U=100.00, d.f.=1, P<0.001) than juveniles in the purple cloth treatment. Juveniles in the control treatment had lower stem length (U=10.00, d.f.=1, P=0.002), leaf area (t=3.56, d.f.=18, P=0.002), SMR (t=10.47, d.f.=18, P<0.001), LMR (t=−3.23, d.f.=18, P=0.005) and LAR (t=−10.34, d.f.=18, P<0.001) than juveniles in the purple cloth treatment (). Number of leaves, DM stem, DM leaf and DM total did not differ between Ricinus juveniles growing under different R/FR (P>0.05).
Figure 4 Means (± SD, when data showed normality) or medians (with confidence interval of 95%, when data showed no normality) of biomass measurements of Ricinus communis juveniles growing under different spectral qualities of light (R/FR). Each point was calculated with the mean value per pot of the variables measured in Ricinus juveniles growing in 10 pots at each light treatment. DM root, root dry mass; RMR, root mass ratio; R/AS, root/aerial system ratio; SMR, stem mass ratio; ROB, stem robustness; SLM, specific leaf mass; LMR, leaf mass ratio; LAR, leaf area ratio.
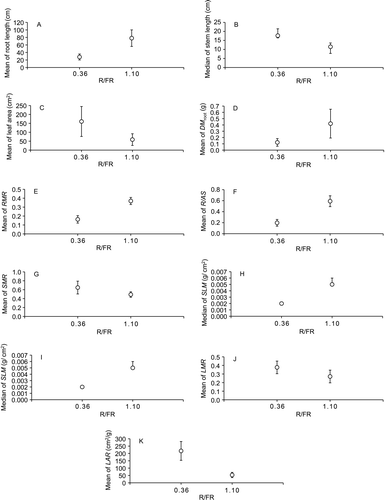
Discussion
Responses of Ricinus seedlings to intra- and interspecific competition
Competition for space and/or nutrients in the soil is considered to be one of the main factors controlling plant growth processes and is crucial for seedling establishment (Whitmore Citation1996). However, our results show that intraspecific competition does not restrict the recruitment of Ricinus seedlings, because the same proportion of juveniles was found at different densities of co-specifics, a pattern also reported for other invasive species (e.g. Barrat-Segretain Citation2005; Burns & Winn Citation2006). This indicates that Ricinus is a poor intraspecific competitor, which is supported by the small differences in biomass allocation of the juveniles growing at different densities of co-specifics: only the number of leaves decreased and the contribution of stem dry mass to the total biomass increased with increasing plant density. Such poor intraspecific competition ability has been documented for many invasive plant species (e.g. Fogarty & Facelli Citation1999; Barrat-Segretain Citation2005; Osunkoya et al. Citation2005; Burns & Winn Citation2006).
Interspecific competition also does not restrict the recruitment of Ricinus seedlings, because the same proportion of juveniles was found at different densities of a potential competitor of another plant species (B. decumbens). This pattern has also been reported for other invasive species (e.g. Barrat-Segretain Citation2005; Burns & Winn Citation2006) and may indicate that Ricinus acts as a strong interspecific competitor when grown with B. decumbens. By contrast, increased plant densities in the interspecific competition experiment resulted in marked differences in biomass allocation of Ricinus juveniles, which may indicate that this species acts as a poor interspecific competitor in relation to B. decumbens. All differences in biomass allocation tended towards a greater investment in root growth when Ricinus was subjected to high densities of co-specifics and of plants of another species. Root growth may be an important mechanism to escape from interspecific competition by reaching deeper soil layers (Harris & Facelli Citation2003).
When comparing the biomass measurements of Ricinus juveniles growing in the same density category of plants of the same species, and of co-specifics and B. decumbens, we noticed that interspecific competition results in a greater impact on Ricinus than intraspecific competition. In general, Ricinus juveniles co-occurring exclusively with co-specifics had more biomass than juveniles co-occurring also with the grass, as reported for other invasive species subjected to interspecific competition (e.g. Fogarty & Facelli Citation1999; Barrat-Segretain Citation2005; Osunkoya et al. Citation2005; Burns & Winn Citation2006). This result indicates that Ricinus is a better intraspecific competitor than it is an interspecific competitor. Because it is a poor intraspecific competitor, we can infer that this species is also a poor interspecific competitor in relation to B. decumbens, even though the latter does not restrict its recruitment. However, Ricinus juveniles subjected to interspecific competition showed a greater contribution of root and stem dry masses to the total biomass, and a greater root biomass in relation to the aerial system biomass when compared with juveniles subjected to intraspecific competition. These biomass alterations can also be attributed to a mechanism of escape from interspecific competition by concentrating resources in roots and stems for further growth in length (Harris & Facelli Citation2003).
It might seem counterintuitive to be calling Ricinus a poor interspecific competitor in relation to B. decumbens, because the former is confined to the margins of vacant lots in Brazil when the two species grow together in the wild. Nevertheless, one alternative explanation for the clumped distribution pattern of Ricinus adults under natural conditions is that the poor interspecific competition ability of this species only translates into less vigorous juveniles and does not lead to a reduction in the population size. Therefore, Ricinus plants in the clumps may be less vigorous when surrounded by B. decumbens than when the latter is not present in the vacant lots, although no mortality should be expected to occur. Further studies should evaluate whether Ricinus adults in clumps surrounded by B. decumbens are, indeed, less vigorous than those that do not co-occur with the grass.
It is important to notice that B. decumbens is a very aggressive competitor (Pivello et al. Citation1999) and also releases allelopathic substances in the soil that inhibit the growth of interspecific plants in its surroundings (Barbosa et al. Citation2008). However, we did not observe any allelopathic effects in our interspecific competition experiment and do not believe that our results have been biased because of them. Although Ricinus recruitment was not negatively influenced by B. decumbens, the former species was outcompeted by this grass even though it may act as a very strong interspecific competitor to other alien or native species. Further studies should evaluate the responses of Ricinus grown with such species in order to elucidate its interspecific competition behaviour.
Responses of Ricinus seedlings to different intensities and spectral qualities of light
Although light acts as a strong selection pressure for plants (Grime Citation1979), Ricinus presented equally successful recruitment when subjected to different intensities and spectral quantities of light. The same number of plants growing under different light intensities was also found by Osunkoya et al. (Citation2005) for the invasive Acacia mangium in Borneo, although McAlpine & Jesson (Citation2007) found reduced survivorship in the shade of the alien Berberis darwinii in New Zealand. Therefore, invasive species seem to present different levels of shade tolerance to recruitment.
When subjected to shading, Ricinus juveniles presented lower root biomass and length, as well as reduced stem biomass. Nevertheless, they invested more in stem length and allocated more resources to leaves (number and area), as described for shade-intolerant species (e.g. Smith Citation1982; McAlpine & Jesson Citation2007). The only unexpected result found by us was the greater total biomass of juveniles growing in the shade, which should be addressed in future studies.
Ricinus juveniles growing under reduced R/FR also presented responses that are consistent with the morphological alterations of shade-intolerant species subjected to this spectral quality of light. On the one hand, they did not present many differences in biomass allocation (only in root dry mass), which was also found for other plant species (e.g. van Tienderen & van Hinsberg 1996; van Hinsberg & van Tienderen 1997). On the other hand, the juveniles allocated more resources to leaves (area and contribution of dry mass to the total biomass) and stem (elongation and contribution of dry mass to the total biomass; Dale & Causton Citation1992; van Hinsberg & van Tienderen 1997). As an unexpected result, Ricinus juveniles also invested more in roots, which may enable nutrient uptake from deeper soil layers (Harris & Facelli Citation2003).
Many species are able to regenerate in both high- and low-light environments (Welden et al. Citation1991), so they are not easily classified into successional status (McAlpine & Jesson Citation2007). Ricinus seems to be one such species: although it presented responses which are consistent with the morphological alterations of shade-intolerant species subjected to shade, it is not truly intolerant to shade, because the species recruits successfully when subjected to different light conditions.
This study has documented developmental effects of light in different plant organs, but the PPFD and R/FR conditions in our experiments are likely to have provided Ricinus juveniles with mixed signals. In the light-intensity experiment, we managed to decrease PPFD without reducing R/FR; in fact, there was an increase in R/FR in the three-layer treatment (). Therefore, the results of the light-intensity experiment are independent of the reduction in R/FR. However, both PPFD and R/FR were drastically reduced in the spectral quality of light experiment (), resulting in Ricinus juveniles with mixed signals. From this experiment, we can only conclude that the development differences we found are due to extreme shading and it is not possible to untangle the effects of intensity and spectral quality of light on Ricinus juveniles. However, Lee et al. (Citation1996) observed little interaction between intensity and spectral quality of light in controlling seedling morphology of Asian tropical rain forest tree seedlings. Further studies are necessary in order to untangle the possible effects of intensity and spectral quality of light on Ricinus juveniles.
Conclusion
Our results show that Ricinus presents high phenotypic plasticity and develops morphological alterations according to the intensity and spectral quality of light; high-plasticity responses were also observed in plants subjected to intra- and interspecific competition. These plastic responses, associated with high seed production (Hogan Citation1992), early maturity, autochorous dispersal, generalist germination behaviour, and rapid growth and recovery after disturbance, probably guarantee the invasion ability of this species (Martins, Guimarães et al. 2009; Martins, Haddad & Semir 2009).
Acknowledgements
We thank Ivany FM Valio and two anonymous reviewers for valuable suggestions, and Guilherme B Sanvido for help during with fieldwork. This research was financed by a Fundação de Amparo à Pesquisa do Estado de São Paulo grant (# 03/11123-4) to the first author.
References
- Barbosa , EG , Pivello , VR and Meirelles , ST . 2008 . Allelopathic evidence in Brachiaria decumbens and its potential to invade the Brazilian Cerrados . Brazilian Archives of Biology and Technology , 51 : 625 – 631 .
- Barrat-Segretain , M-H . 2005 . Competition between invasive and indigenous species: impact of spatial pattern and developmental stage . Plant Ecology , 180 : 153 – 160 .
- Bianchini , M and Pacini , E . 1996 . The caruncle of Ricinus communis L. (castor bean): its development and role in seed dehydration, rehydration, and germination . International Journal of Plant Science , 157 : 40 – 48 .
- Burns , JH and Winn , AA . 2006 . A comparison of plastic responses to competition by invasive and non-invasive congeners in the Commelinaceae . Biological Invasions , 8 : 797 – 807 .
- Camargo AP , Pinto HS , Brunini O , Pedro MJ Jr. , Ortolani AA , Alfonsi RR 1966 . Clima do Estado de São Paulo . In : Setzer J Atlas climático e ecológico do Estado de São Paulo . São Paulo, Brazil, Comissão Interestadual da Bacia Paraná-Uruguai . Pp. 51 87 .
- Corré , WJ . 1983 . Growth and morphogenesis of sun and shade plants. I. The influence of light intensity . Acta Botanica Neerlandica , 31 : 49 – 62 .
- Dale , MP and Causton , DR . 1992 . The ecophysiology of Veronica chamaedrys, V. montana and V. officinalis. I: light quality and light quantity . Journal of Ecology , 80 : 483 – 492 .
- Fogarty , G and Facelli , JM . 1999 . Growth and competition of Cytisus scoparius, an invasive shrub, and Australian native shrubs . Plant Ecology , 144 : 27 – 35 .
- Garwood NC 1989 . Tropical soil seed banks: a review . In : Leck MA , Parker VT , Simpson RL Ecology of soil seed banks . San Diego, Academic Press 149 209 .
- Grime JP 1979 . Plant strategies and vegetation processes . Chichester, Wiley
- Harris , MR and Facelli , JM . 2003 . Competition and resource availability in an annual plant community dominated by an invasive species, Carrichtera annua (L. Aschers.), in South Australia . Plant Ecology , 167 : 19 – 29 .
- Herrera , CM , Jordano , P , López-Soria , L and Amat , JA . 1994 . Recruitment of a mast-fruiting, bird-dispersed tree: bridging frugivore activity and seedling establishment . Ecological Monographs , 64 : 315 – 344 .
- Hinsberg A van , Tienderen PH van 1997 . Variation in growth form in relation to spectral light quality (red/far-red ratio) in Plantago lanceolata L. in sun and shade populations . Oecologia 111 : 453 459 .
- Hogan EL 1992 . Sunset Western garden book . Sunset Publishing Menlo Park, CA
- Holmes , MG and Smith , H . 1977 . The function of phytochrome in the natural environment. I. Characterisation of daylight for studies in photomorphogenesis and photoperiodism . Photochemistry and Photobiology , 25 : 533 – 538 .
- Hunt R 1982 . Plant growth curves: the functional approach to growth analysis Edward Arnold London
- Lagôa , AMMA and Pereira , MF . 1987 . The role of the caruncle in the germination of seeds of Ricinus communis . Plant Physiology and Biochemistry , 25 : 125 – 128 .
- Latham , RE . 1992 . Co-occurring tree species change rank in seedling performance with resources varied experimentally . Ecology , 73 : 2129 – 2144 .
- Lee , DW , Krishnapilay , B , Mansor , M , Mahamad , H and Yap , SK . 1996 . Irradiance and spectral quality affect Asian tropical rain forest tree seedling development . Ecology , 77 : 568 – 580 .
- Levine , JM , Vilà , M , D'Antonio , CM , Dukes , JS , Grigulis , K and Lavorel , S . 2003 . Mechanisms underlying the impact of exotic plant invasions . Proceedings of the Royal Society Series B – Biological Sciences , 270 : 775 – 781 .
- Lisci , M , Bianchini , M and Pacine , E. 1996 . Structure and function of the elaiosome in some angiosperm species . Flora , 191 : 131 – 141 .
- Mabberley , DJ . 1998 . The plant book: a portable dictionary of the vascular plant. 2nd edition , Cambridge : Cambridge University Press .
- Martins , VF , Guimarães , PR , Haddad , CRB and Semir , J . 2009 . The effect of ants on the seed dispersal cycle of the typical myrmecochorous Ricinus communis . Plant Ecology , 205 : 213 – 222 .
- Martins , VF , Guimarães , PR , Silva , RR and Semir , J . 2006 . Secondary seed dispersal by ants of Ricinus communis (Euphorbiaceae) in the Atlantic forest in Southeastern Brazil: influence on seed germination . Sociobiology , 47 : 265 – 274 .
- Martins , VF , Haddad , CRB and Semir , J . 2009 . Seed germination of Ricinus communis in predicted settings after autochorous and myrmecochorous dispersal . Journal of the Torrey Botanical Society , 136 : 84 – 90 .
- McAlpine , KG and Jesson , LK . 2007 . Biomass allocation, shade tolerance and seedling survival of the invasive species Barberis darwinii (Darwin's barberry) . New Zealand Journal of Ecology , 31 : 1 – 12 .
- Osunkoya , OO , Othman , FE and Kahar , RS . 2005 . Growth and competition between seedlings of an invasive plantation tree, Acacia mangium, and those of a native Borneo heath-forest species, Melastoma baccarianum . Ecological Research , 20 : 205 – 214 .
- Pivello , VR , Shida , CN and Meirelles , ST . 1999 . Alien grasses in Brazilian savannas: a threat to the biodiversity . Biodiversity Conservation , 8 : 1281 – 1294 .
- Pons , TL . 1993 . “ Seed responses to light ” . In Seeds: the ecology of regeneration in plant communities , Edited by: Fenner , M . 259 – 284 . Wallingford, , UK : CAB International .
- Probert , RJ . 1993 . “ The role of temperature in germination ecophysiology ” . In Seeds: the ecology of regeneration in plant communities , Edited by: Fenner , M . 285 – 325 . Wallingford, , UK : CAB International .
- Ryser , P and Eek , L . 2000 . Consequences of phenotypic plasticity vs interspecific differences in leaf and root traits for acquisition of aboveground and belowground resources . American Journal of Botany , 87 : 402 – 411 .
- Sanches MC 2004 . Crescimento e atividade fotossintética em duas espécies de lianas de uma floresta estacional semidecidual . Unpublished PhD thesis, State University of Campinas, Campinas, SP .
- Schupp , EW . 1995 . Seed–seedling conflicts, habitat choices, and patterns of plant recruitment . American Journal of Botany , 82 : 399 – 409 .
- Singh , D . 1976 . “ Castor – Ricinus communis (Euphorbiaceae) ” . In Evolution of crop plants , Edited by: Simmonds , NW . 84 – 86 . New York : Longman .
- Smith , H . 1982 . Light quality, photoreception and plant strategy . Annual Review of Plant Physiology , 33 : 481 – 518 .
- Sterner , RW , Ribic , CA and Schatz , GE . 1986 . Testing for life historical changes in spatial patterns of four tropical tree species . Journal of Ecology , 74 : 621 – 633 .
- Stratton , LC and Goldstein , G . 2001 . Carbon uptake, growth and resource-use efficiency in one invasive and six native Hawaiian dry forest tree species . Tree Physiology , 21 : 1327 – 1334 .
- Tienderen PH van , Hinsberg A van 1996 . Phenotypic plasticity in growth habit in Plantago lanceolata: how tight is a suite of correlated characters? Plant Species Biology 11 : 87 96 .
- Valladares , F , Wright , SJ , Lasso , E , Kitajima , K and Pearcy , RW . 2000 . Plastic phenotypic response to light of 16 congeneric shrubs from a Panamanian rainforest . Ecology , 81 : 1925 – 1936 .
- Welden , CW , Hewett , SW , Hubbell , SP and Foster , RB . 1991 . Sapling survival, growth, and recruitment: relationship to canopy height in a neotropical forest . Ecology , 72 : 35 – 50 .
- Whitmore , TC . 1996 . “ A review of some aspects of tropical rain forest seedling ecology with suggestions for further enquiry ” . In Ecology of tropical forest tree seedlings , Edited by: Swaine , MD . 3 – 39 . Carnforth, , UK : UNESCO/Parthenon .
- Zar JH 1999 . Biostatistical analysis , 4th edition . Prentice-Hall Englewood Cliffs, NJ