Abstract
New Zealand's reputation as a supplier of high quality food products is vital to the national economy; international consumers are acutely aware of food safety issues and markets are increasingly demanding higher standards. Filter feeding bivalves are particularly sensitive to the nature of the environment in which they are grown, and quality assurance is a major preoccupation of the shellfish aquaculture industry. With the exception of a couple of incidents, most notably the Gymnodinium catenatum blooms in 2000–2003, paralytic shellfish toxin (PST) contamination has, to date, not had an important effect on the economics and sustainability of the industry. However, the dinoflagellate species responsible for producing these toxins are not uncommon in New Zealand coastal phytoplankton communities, and it is important that awareness of the potential risk is maintained. This review summarises what we know about the causes and incidence of PST contamination from research and monitoring over the last 20 years, since it was first identified in New Zealand. It describes the dynamics of major events and their consequences, and evaluates what is likely to happen in the future as aquaculture expands into new areas with known histories of this problem.
Introduction
Contamination of filter feeding bivalves with paralytic shellfish poisoning (PSP) toxins is a public health hazard and an important quality assurance problem for the New Zealand shellfish aquaculture industry. The toxins involved in PSP are secondary metabolites produced by planktonic dinoflagellates collectively known as paralytic shellfish toxin (PST). These toxins include numerous (> 30) chemical analogues with varying potency, all of which are based on the parent saxitoxin (STX) molecule. They have high affinity for voltage-gated sodium ion channels in nerve cell membranes, inhibiting the generation of nerve impulses and causing the neurological effects, including paralysis, associated with this food poisoning syndrome (Baden et al. Citation1995). Because STX is amongst the most potent natural toxin known, there is a high level of awareness about the risk of PST internationally, and their levels in seafood (expressed as saxitoxin equivalents) are tightly regulated.
There have been several recent incidents that have highlighted the potential seriousness of this issue and which are especially relevant to the New Zealand shellfish industry. During the week of 10 December 2012, at least 20 people were reported to have become ill from consuming surf clams (tuatua; Paphies subtriangulata) collected by recreational gatherers from Bay of Plenty beaches between Mt Maunganui and Papamoa (ESR Citation2012). A public health notice warning against the harvesting of shellfish in the region because of PST contamination was in force at the time. Seventeen people were admitted to hospital and at least two serious cases required treatment in the intensive care unit for several days. The patients exhibited classic symptoms of PSP, shellfish specimens from a batch that caused human poisoning contained high levels of STX, and neoSTX and toxin residues were identified in the urine from an affected individual (T. Harwood, Cawthron Institute, pers. comm. January 2013). This was the most serious documented PSP event that has occurred in New Zealand to date.
Since the summer of 2011 it has been recognised that there is a chronic problem with the occurrence of toxic Alexandrium catenella blooms in Queen Charlotte Sound (QCS). This has resulted in widespread contamination of shellfish with PST (MacKenzie et al. Citation2011, Citation2012, Citation2013). Alexandrium catenella (MacKenzie et al. Citation2004) has been a common member of the phytoplankton on the east coast of the North Island for many years, but it was only identified in QCS in 2010. Alexandrium catenella may be in the process of colonising the coastal waters of the northern South Island. If it becomes established in the main mussel-growing areas of Port Underwood, Pelorus Sound and Tasman and Golden bays, the shellfish aquaculture industry may have to manage production around annual region-wide closures of two to three months in late summer and autumn.
In August 2012 and September 2013, after an apparent absence from the phytoplankton for at least five years, the PST-producing dinoflagellate Gymnodinium catenatum reappeared in samples from the North Island west coast (Manukau and Kaipara harbours, Port Taranaki; ) associated with low levels of contamination of shellfish in the region. In 2000–2001, a major bloom of G. catenatum led to the widespread contamination of shellfish around much of the North Island. This event was the most extensive, and one of the most economically harmful, toxic algae blooms to be documented in New Zealand to date. There were few accounts of human illness associated with the bloom; however, it led to health authorities issuing warnings to the public to refrain from collecting shellfish from over 1500 km of coastline, for periods of over nine months in some regions. Although the main commercial shellfish growing areas (Marlborough Sounds, Coromandel) were only marginally affected, the bloom had an important impact on the shellfish industry throughout the country. This was due to prohibitions being placed on the movement of juvenile shellfish (mussels and oysters) from affected to non-affected areas, because of the risk of the translocation of G. catenatum resting cysts. Unfortunately, much of the premium seed source for the oyster and mussel industries lay within affected areas and, as a result, there was a shortage that influenced production in subsequent years.
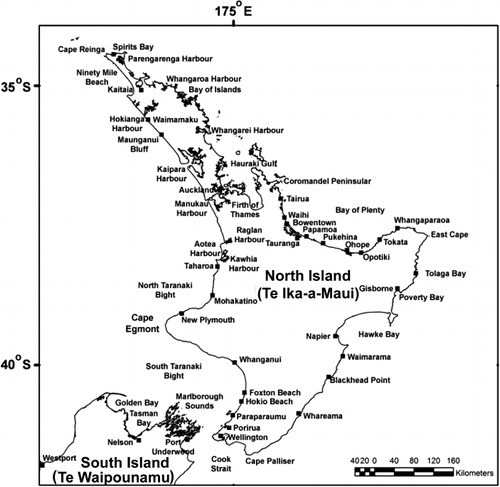
In October 2012, the Australian shellfish industry suffered a setback when Japanese health authorities found unacceptable levels of PST in a shipment of blue mussels (Mytilus galloprovincialis) originating from the east coast of Tasmania (Campbell et al. Citation2013). This resulted in a global recall of products from this source and extended closures (up to 100 days) of important bivalve production areas. The shellfish poisoning was the consequence of a widespread, but undetected, bloom of the toxic dinoflagellate A. tamarense. This species had been known to be present in this region for some time (de Salas et al. Citation2001). The event caused an estimated direct revenue loss of around A$6.3 million to the mussel industry, and around A$0.8 million and A$1 million to the scallop and rock lobster fisheries respectively. When an economic multiplier was applied to this loss of revenue, the total economic impact was estimated at approximately A$25.6 million. These losses were attributed to a breakdown in the Tasmanian Biotoxin Management Plan procedures, which resulted in inadequate phytoplankton and shellfish toxin monitoring being carried out in the affected region before and while the shellfish were being harvested. This resulted in failure to detect the A. tamarense bloom and the PST in the shellfish, and led to the sale and export of contaminated products.
The Tasmanian event serves as a reminder to the New Zealand industry that complacency about the risks of PSP can have catastrophic effects. New Zealand's reputation as a supplier of high quality and safe seafood provides a competitive advantage (Busby & Seamer Citation2004) and it is crucial that shellfish products containing unacceptable levels of PST residues do not slip through the surveillance system and appear on local or international markets. To ensure this, participants in the shellfish industry need to be fully informed about the nature and risks of these phenomena as we currently understand them. The aim of this review is to present a synopsis of what is now known about PST contamination of shellfish in New Zealand, from research and monitoring that has taken place over the two decades since it was first identified. The review will:
Summarise research on the toxicity and ecology of the dinoflagellate species that are responsible for the production of PST in New Zealand
Explain changes that have taken place in the toxin monitoring methods.
Review the history and dynamics of the major PST events that have taken place over the last 20 years.
Describe the impacts that major blooms have had on the aquaculture industry and the various measures that have been taken to mitigate these effects.
Paralytic shellfish poisoning regulation, testing and monitoring
Agreements between the New Zealand Ministry for Primary Industries (MPI) and major international food safety agencies, such as the US Food and Drug Administration (USFDA) and European Food Safety Authority (EFSA), permit MPI to manage surveillance and monitoring of export shellfish for algal-biotoxin contamination. Biotoxin regulation and monitoring procedures for commercial shellfish harvesting in New Zealand are specified within the Animal Products (Regulated Control Scheme – Bivalve Molluscan Shellfish) Regulations and Specifications 2006. MPI also administers the monitoring programme that oversees non-commercial shellfish gathering.
Important changes have been made in the last few years to the method used to test for PST in shellfish in New Zealand. Until 2010 all testing was carried out using the standard mouse bioassay (MBA) method (APHA Citation1970; AOAC Citation1990). This method quantified PSP toxicity by the time it took mice to die with the characteristic symptoms of PSP, within one hour of injection of acidic shellfish extracts. The prohibited level was > 80 µg saxitoxin equivalents/100 g of shellfish flesh. This method has been used internationally for at least six decades and has a limit of detection of approximately 37 µg STX equivalents/100 g. In 2010, because of technical (false positive and negative results, poor sensitivity) and ethical problems associated with the MBA, including European Union (EU) legislation limiting the use of animals for regulatory food testing, an assessment of alternative analytical methods was made (Holland et al. Citation2010a). A chemical analysis (the Lawrence HPLC/pre-column oxidation method; Lawrence & Niedzwiadek Citation2001; Lawrence et al. Citation2004, Citation2005) was selected and authorised for routine use by MPI in mid-2010, after a thorough evaluation of its performance (Holland et al. Citation2010b). An effect-based assay, such as the MBA, provides an approximate measure of the total toxicity of the sample in one step. Chemical analysis requires the quantification of each individual toxin type in the sample (there are >12 common and >20 rarer analogues with varying potencies), calculation of the toxicity (in STX equivalents) contributed by each analogue, and summing of these values to arrive at an estimate of total toxicity. The method is complex, but it is sensitive, and provides accurate estimates of total toxicity (Harwood et al. Citation2013). All public health and shellfish industry testing is now carried out this way in New Zealand. To simplify the method, and make it more cost effective, a two-tier system has been adopted. This involves a rapid screening method that detects toxins and assigns the analogues to broad categories. Most shellfish samples are negative and this efficiently identifies these, while for those samples that contain toxins it provides an approximate toxicity value. To obtain a definitive estimate of toxicity (if required), a second confirmation analysis needs to be carried out. Estimates of total toxicity critically depend on the toxicity equivalency factors used in the calculations, which are currently being experimentally revised (Munday & Reeve Citation2013; Munday et al. Citation2013). Total toxicity is now reported in mg STX equivalents/kg of shellfish flesh. The current internationally accepted regulatory level is 0.8 mg STX equivalents/kg and the Lawrence method has a reporting limit of 0.1 mg STX equivalents/kg (Harwood et al. Citation2013). In 2009, the European Food Safety Authority (EFSA) carried out a toxicological evaluation of the acceptable level of PST in shellfish (EFSA Citation2009) based on the Authority's review of reports of human intoxications. It suggested that the acceptable level should be reduced to as low as 0.075 mg STX equivalents/kg, which is more than 10 times less than the current level. However, the EFSA conceded that there were too many uncertainties regarding dietary exposure levels and differences in extraction methods for it to make a definite recommendation. If this lower level was adopted, it would be very harmful to shellfish industry interests. It would be a standard that would be very difficult, if not impossible, to meet in practice because 0.075 mg STX equivalents/kg is below the detection level of currently validated methods (i.e. the MBA and the Lawrence HPLC methods) and would substantially lengthen closure periods. The conclusions of the EFSA have been challenged by Kiermeier et al. (Citation2009) who identified significant weaknesses in several aspects of the EFSA analysis. These included: the paucity and quality of data on exposure of consumers to STX-group toxins; biased consumption data; uncritical evaluation of epidemiological data; lack of peer review; and ignoring the recommendation of the Codex Alimentarius Committee on Fish and Fisheries products (FAO/WHO Citation2006), which stated that no change in the current level of 0.8 mg STX equivalents/kg was necessary.
Although there had been some prior research and monitoring aimed at identifying PST in the Marlborough Sounds (MacKenzie Citation1989), a nationwide shellfish biotoxin monitoring programme did not begin until 1993 (Trusewich et al. Citation1996). This was as a result of the neurotoxic shellfish poisoning (NSP) crisis on the North Island east coast that year (Chang et al. Citation1995; MacKenzie et al. Citation1995a). During the first year of the programme 165–200 sites per week were sampled around the country, at a cost of around NZ$4.3 million/annum. This was scaled back to 120 sites per week for the 1994–1995 year (at a cost of around NZ$3.2 million/annum). This trend has continued as priority areas have been identified and phytoplankton monitoring has played a greater, more cost effective, role in the programme (Todd Citation1997; Chang Citation2004; NZFSA Citation2007; Rhodes et al. Citation2001, Citation2013). In 1996, the programme was divided into two: an industry programme run and funded by local Shellfish Quality Assurance Delivery Centres; and a government-funded, non-commercial, public health protection programme. In 2013, shellfish from about 20 sites per week were analysed for PST for the shellfish industry and about eight per week for the public health programme.
Toxic Alexandrium species in New Zealand
Of the 25 morpho-species of the dinoflagellate genus Alexandrium described by Balech (Citation1995), at least 10 have been identified in New Zealand coastal waters (Cembella et al. Citation1987; MacKenzie et al. Citation1996a; Chang et al. Citation1997; MacKenzie & Berkett Citation1997; MacKenzie & Todd Citation2002; MacKenzie et al. Citation2004). New Zealand isolates of four of these species, A. catenella, A tamarense, A. ostenfeldii and A. minutum, produce PST in culture (MacKenzie et al. Citation1996a; MacKenzie & Berkett Citation1997; MacKenzie & Taylor Citation2004). However, only A. catenella, A. tamarense and A. minutum have been implicated in actual shellfish poisoning events. These species are well known as the cause of PSP elsewhere in the world (e.g. Moore et al. Citation2009; Aguilera-Belmonte et al. Citation2011) and pose the greatest risk to aquaculture. Cultured isolates of A. ostenfeldii have included highly toxic and completely non-toxic strains (MacKenzie et al. Citation1996a). The cysts of this species are common in coastal sediments around New Zealand, but it is only rarely observed within the plankton. This is probably the reason why no PST contamination events have been attributed to it. Alexandrium catenella and A. tamarense isolates from the North Island east coast are morphologically difficult to discriminate by light microscopy (they differ only by the presence or absence of a ventral pore in the 1st apical plate). Molecular analysis of A. catenella and A. tamarense isolates from elsewhere in the world (e.g. Lilly et al. Citation2007; Lilibeth et al. Citation2012) have shown that these species are a complex which cannot be distinguished on morphological grounds. Likewise, New Zealand isolates of these species have been shown to have identical toxin profiles and LSU rRNA gene sequences (MacKenzie et al. Citation2004). Records of ‘A. catenella’ from this region are, therefore, likely to represent a mix of both morpho-types.
Alexandrium catenella
Cells of A. catenella (28–36 µm wide × 22–30 µm long) occur as solitary cells or in pairs and chains of variable lengths of up to 16 cells per chain (). Alexandrium catenella is a prolific producer of benthic resting cysts (hyponozygotes) that are smooth walled and oval shaped. The interior of the cyst is clear with a prominent pigment accumulation body and they are often surrounded by a fragmentary mucilaginous capsule (). Cells of A. catenella have been observed at numerous locations around the North Island coast over the last 20 years, but it is most frequently observed, and generally occurs in highest numbers, on the North Island's east coast from the Bay of Plenty northwards (). All the records of A. catenella in the South Island are from the Marlborough Sounds. These records only date from 2010 when the first cells were seen in routine monitoring samples from QCS. However, it is now known, from the distribution of cysts in sediment cores, that this species must have been resident in the region for an extended period before this (see below).
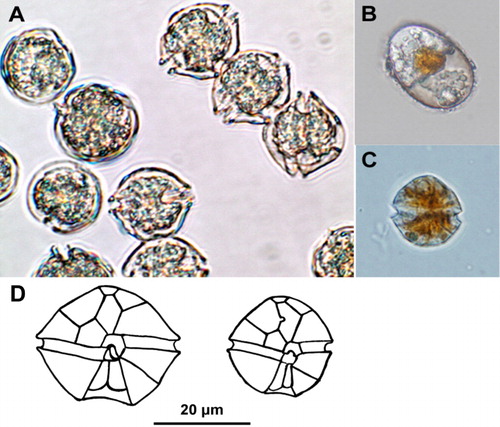
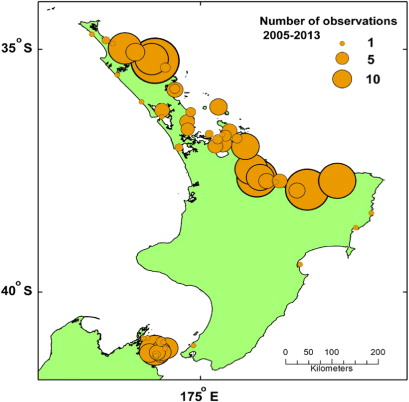
The toxin profiles of wild and cultured cells of A. catenella and shellfish that have been exposed to the dinoflagellate have been determined on a number of occasions. MacKenzie et al. (Citation2004) analysed 14 cultured isolates from the Bay of Plenty using the post column oxidation HPLC method of Oshima (Citation1995). The toxin profiles were dominated by almost equal proportions of the low toxicity N-sulfo-carbamoyl analogues (C1,2 and C3,4), with minor amounts of the higher toxicity analogues GTX1,4 and neoSTX. The total mean toxin content of these cells was rather high (150 fmol/cell) but the predominance of the low toxicity C toxins resulted in a low mean specific toxicity (3.4 pg STX equivalents/cell). The toxin profiles of Greenshell™ mussels (Perna canaliculus) were very similar to those of the dinoflagellate to which they were exposed (MacKenzie et al. Citation1997). Analysis of the toxin profiles of A. catenella isolates from QCS using the Lawrence pre-column oxidation HPLC method (Harwood et al. Citation2013; MacKenzie et al. Citation2013) have shown that these South Island strains also produce c. 50% low toxicity N-sulfocarbamoyl toxins (C1–4 and GTX5–6) but also a high proportion (c. 50%) of the higher potency GTX1–4 toxins (). The toxin profiles of New Zealand strains of A. catenella closely resemble those from elsewhere in the world; for example, A. catenella isolates from the northeast Pacific (Cembella et al. Citation1987) and Korea (Kim et al. Citation2005). Chilean strains of A. catenella, reputedly responsible for several hundred poisonings and 25 deaths over three decades, are also characterised by the dominance of N-sulfocarbamoyl (C1,2) and gonyautoxin (GTX1,4) analogues (Krock et al. Citation2007; Varela et al. Citation2012). However, a genetic and toxicological study (Aguilera-Belmonte et al. Citation2011) of seven Chilean isolates has shown that some strains had toxin profiles containing significant proportions of high toxicity analogues (neoSTX and STX). Extremely high levels of toxicity (> 127 mg STX equivalents/kg) have been found in Chilean mussels due to contamination by A. catenella (Benavides et al. Citation1995).
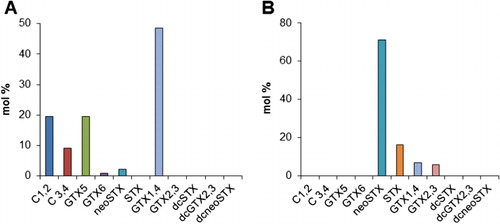
Alexandrium minutum
The morphology of Bay of Plenty and Marlborough Sounds isolates of A. minutum were described by Chang et al. (Citation1997) and MacKenzie & Berkett (Citation1997) respectively. This species is mainly distinguished from A. catenella by its smaller size (–). The cells are slightly longer than wide (22–25 µm × 20–21 µm) and generally oval in ventral view, with the epitheca more highly domed than the hypotheca, which is almost hemispherical. There are two other small Alexandrium species (A. angustitabulatum and A. camurascutulum) described from New Zealand waters. These are very similar in general size and shape to A. minutum and are unlikely to be discriminated from this species during routine monitoring. Alexandrium angustitabulatum was identified (February 1983) from a ‘red tide’ sample from Whangarei Harbour, Northland, and was shown by Cembella et al. (Citation1987) to produce PST (96% GTX1,4) in culture. Alexandrium camurascutulum was described from the Marlborough Sounds (MacKenzie & Todd Citation2002). It is unknown whether this species produces PST because live specimens have not been isolated and cultured. Chang et al. (Citation1997) analysed the PST profiles of two cultured isolates of A. minutum from the Bay of Plenty (). They found that neoSTX was the principal toxin (> 65 mol%) with STX and GTX1,4 as lesser components. The specific toxicity of these cells was at the high end (6.0–11.5 pg STX equivalents/cell) of the range of toxicity reported for A. minutum strains elsewhere in the world. They are somewhat higher than the toxicity of A. minutum strains (1.8–2.4 pg STX equivalents/cell) isolated from the Marlborough Sounds (MacKenzie & Berkett Citation1997). These had toxin profiles dominated by GTX1,4 and GTX2,3 (33 mol% and 23 mol% respectively) but also with significant amounts of neoSTX and STX (18 mol% and 26 mol% respectively) as well. The low toxicity N-sulfocarbamoyl C-toxins (C1–4) or gonyautoxins (GTX5–6) are not produced by any New Zealand A. minutum isolates. The greater proportion of the more highly toxic PST analogues in A. minutum compared to A. catenella may make contamination by this species more dangerous to human consumers. This is the likely reason why the December 2012 shellfish poisoning event in the Bay of Plenty was so severe.
Alexandrium blooms in the Bay of Plenty
A large aquaculture development (Eastern Seafarms Ltd) is underway in the eastern Bay of Plenty. Resource consent has been granted for a 3800 ha mussel farm a few kilometres off the Opotiki coast (). The farm has the potential to produce around 20,000 tonnes of mussels per annum when fully established and it is hoped it will become an important new employment opportunity in the region. This development, which is still in an experimental phase, is in a region that has a chronic problem with PST contamination. As shellfish production comes onstream, special care will need to be taken to ensure that contaminated products do not enter the food chain. The PSP problem in the Bay of Plenty springs from the frequency of blooms of A. minutum and A. catenella, which result in the periodic widespread contamination of shellfish along the bay's coastline.
The long-term retention of toxin residues in surf clams (tuatua, Paphies spp.), which are abundant along the sandy surf beaches of the bay, is a particular problem. These species have always figured disproportionately in national shellfish biotoxin contamination statistics. In the early years of monitoring in New Zealand, large numbers of sites around the entire country were sampled weekly and screened for water soluble and lipophilic algal toxins by MBA. These data provided an excellent nationwide picture of the real magnitude of these phenomena. Between January 1994 and January 1995, 5327 MBAs to detect PSP toxins, were carried out on a variety of shellfish species from an average of 102 sites per week. Of the species analysed, 10% were tuatua. Only c. 3% of the bioassays on all species returned positive results, of which c. 80% were in tuatua, and all but one were the result of low level residues in Bay of Plenty samples. By 1999, after a substantial reduction in the number of monitoring sites, 1660 MBAs were carried out annually. Of these, 8% detected PSP toxins, 85% of which were due to low level residues in Bay of Plenty tuatua.
Over the last 11 years (2003–2013) public health warnings cautioning people against consuming shellfish have been in place for 61 out of 132 months (i.e. 46% of the time) in the Bay of Plenty (Toi Te Ora Public Health Service, Bay of Plenty District Health Board data). Some years (e.g. 2003, 2004, 2005, 2008) only low levels of toxicity have been detected and the beaches have been open for shellfish gathering most of the time. Other years (e.g. 2006, 2010, 2012, 2013) the beaches have been closed the entire year. MacKenzie et al. (Citation1996b) examined changes in the toxicity and PST profiles in populations of Paphies subtriangulata from the Bay of Plenty. This was undertaken during the contamination phase caused by a bloom of A. minutum in January 1993 and over a six-month period one year later when low level toxin residues persisted in these shellfish. During the peak of toxicity (4.1 mg STX equivalents/kg) the toxin profiles resembled those of A. minutum, with a mixture of STX, neoSTX, GTX1,4 and GTX1,3. One year later, when the toxicity had declined to a stable level of around 0.4 mg STX equivalents/kg, STX was overwhelmingly dominant (94%–100%) and was located almost exclusively in the syphons. The change over time of the toxin profiles and long residence time of toxins in P. subtriangulata syphons is similar to the characteristics of other surf clam species elsewhere in the world (Shumway et al. Citation1994).
Not much is known about the environmental drivers, seasonality and dynamics of Alexandrium blooms in the Bay of Plenty, or why there appears to be alternating periods of abundance and shellfish contamination by A. minutum and A. catenella, but the former may be more common in the western side of the bay and the latter on the eastern side. The only existing data on the spatial distribution of cells in the bay during a bloom were provided by Chang et al. (Citation1996). They first identified A. minutum in the western side of the bay in January 1993, as the result of a high cell density (>1.2 × 105 cells/litre) bloom that occurred when surface seawater temperatures and salinities were, respectively, lower and higher than normal for the time of year. Cell numbers of A. minutum were highest close to the shore and generally decreased eastwards from Tauranga/Mt Maunganui. They believed the bloom was associated with the nearshore upwelling of nutrient rich waters in the western bay, possibly associated with the El Niño southern oscillation phase persisting at the time.
The first major PSP-toxin contamination event attributable to A. catenella occurred in the eastern Bay of Plenty (Whangaparaoa, Ohope Beach) in late March 1996 (MacKenzie et al. Citation1996c). The toxicity (determined by MBA) in mussels (Perna canaliculus) at Whangaparaoa reached a level of around 9 mg STX equivalents/kg. The appearance of toxicity in shellfish progressed from east to west along the shore of the bay and, in late May, tuatua at western sites (Papamoa Beach) showed an increase in toxicity over the normal background. The toxicity in the Whangaparaoa mussels declined rapidly and, by early May, no trace of PSP toxicity could be detected. Typically, the toxicity of the Ohope Beach tuatua declined slowly and was still above the quarantine level in late September.
An A. catenella bloom reoccurred the following year and high levels of toxicity (> 10 mg STX equivalents/kg) were found in mussels in the eastern Bay of Plenty (Whangaparaoa, Tokata) in April 1997 (MacKenzie et al. Citation1997). The contamination from this bloom progressed across the bay westwards as far as Ohope and Pukehina beaches (). Shellfish on beaches further to the northwest (Waihi and Papamoa) remained at normal low background levels. Maximum numbers of A. catenella (1.1 × 105 cells/litre) were observed at Te Kaha on 29 April 1997, associated with reports of cloudy, discoloured (brown) water in the area at the time.
Examination of the Bay of Plenty shellfish toxicity data () shows that significant contamination events are almost an annual occurrence. In some years this is due to blooms of A. catenella, in others to A. minutum. Blooms can occur in spring, summer or autumn with midwinter being the only time when contamination events are rare. Routine public health monitoring of toxicity and phytoplankton in the Bay of Plenty clearly identified the cause of the December 2012 poisoning incident as due to a bloom of A. minutum (). The actual toxicity values in need to be treated with some caution. These figures represent an HPLC screen test using a method (Assure Qual) designed to alert health authorities to the presence (or absence) of toxins, not their accurate quantification (Jim Sim, MPI, pers. comm. January 2013). Independent analysis (T. Harwood, Cawthron Institute, pers. comm. January 2013) of a tuatua sample from Papamoa Beach at the time of the poisonings using the validated Lawrence method (Holland et al. Citation2010a,Citationb; Harwood et al. Citation2013), returned screen and confirmation estimates of 31 and 14 mg STX equivalents/kg respectively.
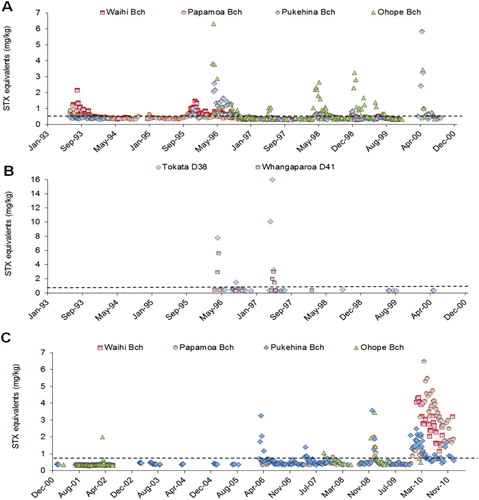
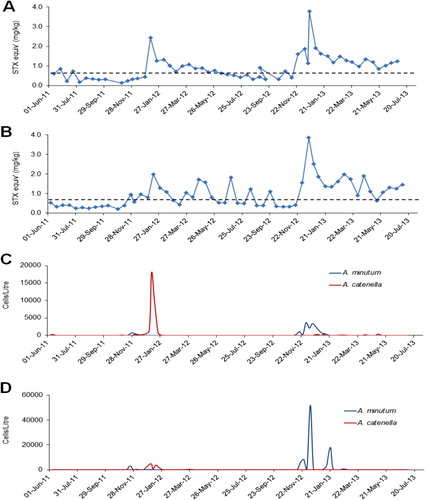
It is unknown whether the location of the Opotitki mussel farm is susceptible to the influence of these blooms as no testing at the actual farm site has been carried out so far. However, it would be surprising if the farm was not affected to some extent. The Ohope Beach monitoring site, which has a long record of PST-positive samples, is almost directly inshore of the farm. Once the farm is in operation it may only be possible to harvest during periods between bloom events. To be forewarned of impending blooms, it will be necessary to carefully monitor shellfish and phytoplankton, informed by shoreline testing and knowledge of water movements in the bay. Because of the large size of the farm (3800 ha) a spatially representative sampling protocol will need to be established.
Alexandrium blooms in the ‘Top of the South’
The ‘Top of the South’ region, encompassing the Marlborough Sounds (Pelorus Sound, QCS, Port Underwood and Croisilles Harbour) and Tasman and Golden bays, is the major shellfish (mainly mussels) growing area in the country. It has an annual production of around 70,000 tonnes/year valued at around NZ$160 million/year in exports. Shellfish aquaculture began in this region in the 1970s, and although there were several minor PST contamination episodes, until 2011 (excluding the Gymnodinium catenatum/Kaitaia spat issue; see below) these had little direct effect on the industry.
Alexandrium minutum in the Marlborough Sounds
Occasional sightings are made of A. minutum in the Marlborough Sounds phytoplankton, but associated shellfish PST contamination is rare. There have been two minor incidents over the last 20 years, one of which resulted in harvest closures (MacKenzie et al. Citation1994). During the late winter, spring and summer 1993, A. minutum was observed in moderate numbers (≤ 1.5 × 103 cells/litre) on several occasions in water samples from Croisilles Harbour. During this period, low level PST-positive mouse assays (≤ 0.4 mg STX equivalents/kg) were detected and there was an association between A. minutum at concentrations > 500 cells/litre and this toxicity. In December 1993, A. minutum appeared in samples from Anakoha Bay in the outer sounds (4–7 × 103 cells/litre), along with positive bioassays for PST in mussels (> 0.5–1.3 mg STX equivalents/kg). A survey of the phytoplankton and bioassay of mussels in the outer sounds showed that low level contamination was confined to Anakoha and Forsyth bays. A limited harvest closure of these areas was imposed for several weeks, but the impact on the industry as a whole was minor.
Alexandrium catenella in Queen Charlotte Sound
Although weekly phytoplankton and shellfish biotoxin monitoring has been taking place at five to six sites in QCS since the early 1990s (), A. catenella was not observed in this region until March 2011. At this time, a major bloom developed that resulted in high levels of toxicity in cultured mussels and oysters (–). This caused a prolonged harvest closure in the area and raised fears that A. catenella may spread to the main mussel production areas of the sounds (MacKenzie et al. Citation2011; Harwood et al. Citation2013). The first cells were seen in Tory Channel and a low level of toxicity was detected (0.7 mg/STX equivalents/kg) at the Hitaua Bay (Tory Channel) monitoring site in early March 2011. Within one week, cell numbers and toxicity increased substantially at this location. The bloom became more widely dispersed, extending as far as East Bay, where numbers and toxicity peaked (at 1.3 × 105 cells/litre and 17 mg STX equivalents/kg respectively) on 20 April 2011 (). The suite of PST analogues produced by A. catenella isolates from QCS are dominated by low toxicity N-sulfocarbamoyl analogues (principally C1,2) and GTX1,4. Nevertheless, the total PSP-toxin concentrations measured in these shellfish were amongst the highest ever recorded in New Zealand. The dinoflagellate bloom persisted until mid to late April, although the prohibition on commercial shellfish harvesting at East Bay did not end until 20 June 2011. The maximum closure period was 97 days (). Surveys and monitoring of cell numbers and resting cyst concentrations in the sediments throughout QCS (MacKenzie et al. Citation2012, Citation2013) have identified Opua Bay (Tory Channel) as the origin of annually recurrent blooms of A. catenella. Very high numbers of planktonic cells (> 1.0 × 106 cells/litre) and cysts (up to 1.5–3.5 × 107 cyst/m2) have been observed at this location (). Monitoring of the seasonal development of the bloom at this site has enabled predictions of the onset of toxicity at other sites in QCS. A few motile cells of A. catenella have been observed in water samples from outer Pelorus Sound and in Port Underwood, but no resting cysts have been found in the sediments of these areas. The dinoflagellate also does not appear to have become established outside QCS as yet.
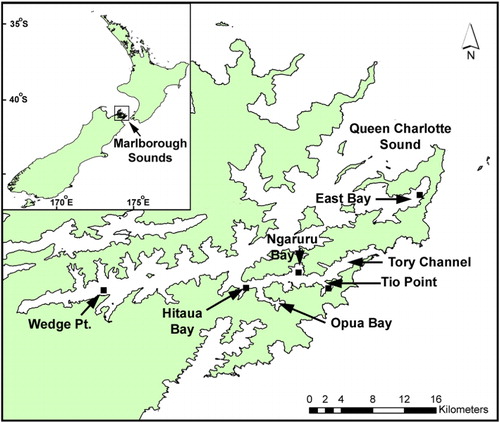
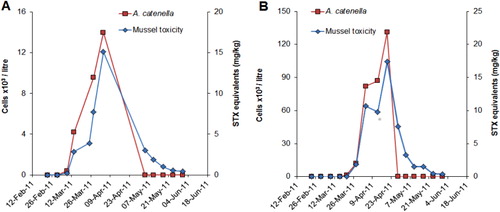
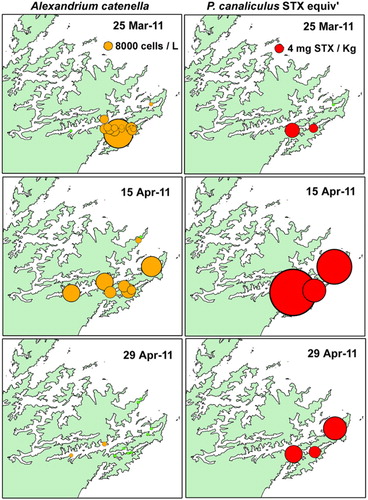
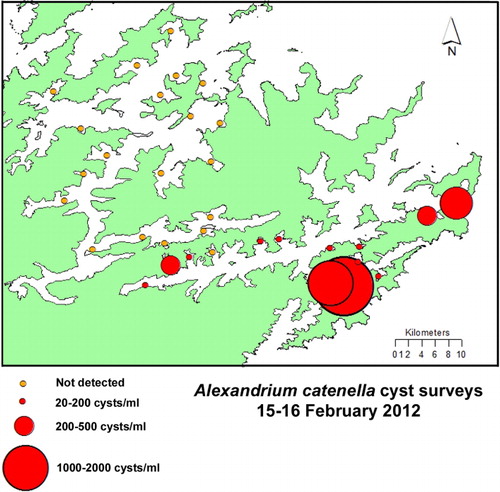
Table 1 Shellfish harvesting closure and opening dates in commercial and recreational gathering areas of Queen Charlotte Sound (QCS) due to A. catenella.
Investigations focused on developing an understanding of the autecology of A. catenella in QCS in general, and Opua Bay in particular, have been carried out over the bloom period (January–May) each year since 2011 (MacKenzie et al. Citation2011, Citation2012, Citation2013). The timing of bloom development in Opua Bay, presumably due to cyst germination, is predictable and it is highly likely that they will continue to occur every year into the future. Analysis of cyst distribution and isotope dating of strata in sediment cores from Opua Bay suggests that A. catenella has been depositing cysts at this location for at least three decades (A.L. MacKenzie, Cawthron Institute, unpubl. data). In Opua Bay, A. catenella cells occur in the water column from late spring onwards. However, the bloom does not enter its exponential growth phase until late January to early February, when cell numbers increase rapidly if conditions are favourable. An important factor in bloom development is the establishment and maintenance of a stable, thermally stratified, water column in this sheltered inlet. Opua Bay is exposed to cool fertile waters within Tory Channel. These waters have a high inorganic nutrient content (especially of nitrate) all year, which originates from the deep offshore waters of Cook Strait (Marlborough District Council water quality data). The magnitude of the A. catenella blooms in Opua Bay are affected by year-to-year changes in physical and biological conditions within the water column. During the 2011–2012 summer, bloom development was suppressed by strong southeasterly winds in late February and early March, which lowered water temperatures and disrupted water column stratification. From late March to early April, A. catenella increased in abundance again as stratification became re-established. It did not become dominant again because of competition from a co-occurring non-toxic dinoflagellate (Akashiwo sanguinea) and the lateness of the season. The bloom did not become established to any great extent beyond Opua Bay and no shellfish closures were necessary that year. In contrast, Marlborough experienced an exceptionally fine and sunny summer in 2012–2013. This created the ideal conditions (high light, stable stratified water column) that resulted in the proliferation of A. catenella in Opua Bay. An intense bloom (with cell concentrations exceeding 1.0 × 106 cells/litre) developed in mid-March throughout the bay, causing extensive areas of visible ‘red tide’. The bloom extended to other areas of QCS, and the timing of shellfish contamination at these locations was similar to that in 2011, but the magnitude and duration was less. In Tory Channel (Hitaua Bay) and East Bay, harvesting was suspended from 25 March to 16 May (71 days). The toxicity at sites affected by Tory Channel water flows were probably due to the export of cells from Opua Bay. At East Bay, however, it is likely that the bloom developed independently from cyst beds that are known to exist there ().
Although A. catenella has clearly been resident and blooming annually in isolated embayments of QCS for at least several decades, it is unknown why, within the last few years, it has apparently extended its range. Weekly phytoplankton monitoring has been in progress in QCS since the early 1990s and it is unlikely that it would have escaped attention if it had been present in significant numbers within the wider Sound over that period. The situation in QCS is similar to that in Puget Sound (Washington State, USA). In this area, the link between A. catenella and an associated PST contamination has been known for centuries (Trainer et al. Citation2003) and routine monitoring has been in place since the 1940s. There are frequent closures of commercial and recreational shellfish harvesting in Puget Sound. In the 1980s, PST spread into previously unaffected areas in southern Puget Sound, which contains some of the most productive recreational and commercial shellfish regions on the US west coast. Large-scale oceanographic changes (Pacific Decadal Oscillation) and increased eutrophication of nearshore areas were suggested as possible reasons for the increased problem with PSP in this region. Both QCS and Puget Sound are complex fjords with numerous enclosed embayments off the main waterways, where high resting cyst numbers are found in the sediments and where A. catenella thrives under summer stratified conditions. Sequim Bay and Quartermaster Harbour in Puget Sound are two embayments that have high cyst densities in the sediments (Cox et al. Citation2008; Tobin & Horner Citation2011; Feifel et al. Citation2012) and are the origin of annually recurrent blooms. Sequim Bay is flooded by freshly upwelled, high nitrate water from the Straits of Juan de Fuca, similar to the way that Opua Bay receives high nitrate water from Tory Channel.
Alexandrium catenella/tamarense blooms have been associated with fish deaths on salmon farms in Canada (Cembella et al. Citation2002), the Faroe Islands (Mortensen Citation1985) and Chile (Fuentes et al. Citation2008). In the Aysén region of Chile in 2002, a bloom of A. catenella caused over 50 human cases of illness, three fatalities and losses of > 1800 tonnes of farmed salmon (Fuentes et al. Citation2008). In 2006, a reoccurrence of the bloom again caused salmon losses of around 1800 tonnes valued at US$9.2 million. During bloom episodes, A. catenella has been observed within salmon farms in QCS, but cell numbers have generally been low and no adverse effects on the health of the fish have been reported.
Gymnodinium catenatum in New Zealand
Gymnodinium catenatum is the only non-thecate, gymnodinoid dinoflagellate known to produce PST. It occurs in temperate and tropical regions worldwide (Hallegraeff et al. Citation2012) and has caused problems for shellfish aquaculture in a number of countries (e.g. Spain, Portugal, Tasmania, Japan, Mexico), including New Zealand. For example, in Spain, G. catenatum was first detected as the cause of a PSP epidemic in several European countries after people consumed commercially exported mussels from the northwestern Spanish Rias (Luthy Citation1979). This led to the initiation of shellfish and toxic phytoplankton monitoring in Spain.
In May–June 2000, G. catenatum was first observed in seawater samples from the North Island's west coast and PST were detected in shellfish from this region. This signalled the beginning of a major bloom which led to the widespread contamination of shellfish around much of the North Island (MacKenzie & Beauchamp Citation2011). This was the most extensive, and one of the most harmful, toxic algae blooms to be documented in New Zealand to date. Gymnodinium catenatum persisted in some areas (e.g. Kaipara Harbour, Hawke Bay) for several years after the major blooms in 2000–2003, but its incidence gradually dwindled until it appeared to vanish from the plankton in 2007. In August 2012 and September 2013 it again reappeared in samples from the North Island west coast (Manukau and Kaipara Harbours, Port Taranaki), associated with low levels of PST in shellfish in the region. It is likely that, in the future, a repeat of the extensive bloom of early 2000 will take place and the shellfish industry will again confront biosecurity issues regarding the transport of mature and juvenile shellfish around the country. It is important that the aquaculture industry is informed about what is known about the dynamics of the blooms that took place then, their consequences and the management measures that were taken to mitigate these effects.
Gymnodinium catenatum morphology
The morphology of motile vegetative cells and resting cysts of New Zealand strains of G. catenatum are identical to descriptions of this species (e.g. Blackburn et al. Citation1989) from elsewhere in the world (). In nature and in culture it exists as solitary cells or, more usually, in chains of variable length of eight to 16 cells and up to 64 cells per chain (). The vegetative cells can become swollen and distorted after preservation with Lugol's iodine, but despite this are usually identifiable by skilled observers. In some cases, preserved cells can be confused with cells of other non-toxic chain-forming gymnodinoid dinoflagellates such as Gymnodinium impudicum and, when low numbers of solitary cells are present in preserved samples, definitive identification can be difficult on morphological grounds alone. The spherical resting cyst (hypnozygote) of G. catenatum is 45–50 µm in diameter (–) and has a prominent red pigment accumulation granule within the globular cytoplasm. The surface of the cysts has a distinctive honeycomb-like reticulate pattern that is visible with phase contrast microscopy ().
Development and progression of the 2000–2001 bloom
Contamination of mussels with low levels of PST (0.35 mg STX equivalents/kg) were first detected in samples collected on 15 May 2000 from the Manukau Harbour (northwest coast of the North Island) during a monitoring programme undertaken by the New Zealand Ministry of Health (). Toxicity was also detected in shellfish samples from Maunganui Bluff and in Raglan Harbour on 20 June, before the first seawater samples were collected from the Manukau on 20 June, within which G. catenatum cells were observed (MacKenzie & Adamson Citation2000). From then, the spatial extent and frequency of sampling was increased and it became apparent that the bloom was intensifying and expanding, both in terms of cell numbers and shellfish toxicity levels (–). The results of the shellfish toxin assays show the spread and the waxing and waning of toxin levels around the North Island coast (). The highest level of PSP toxicity recorded was 40.3 mg STX equivalents/kg in Greenshell™ mussels (Perna canaliculus) from Waimamaku in Northland (). Of all the positive bioassays attributable to G. catenatum contamination (a total of 537 up to 9 February 2001), 17.5% and 7.3% were at levels > 5.0 and > 10.0 mg STX equivalents/kg respectively.
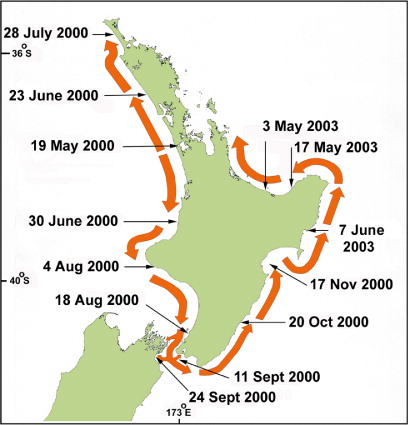
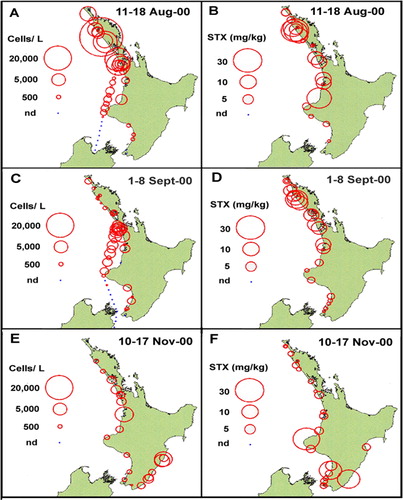
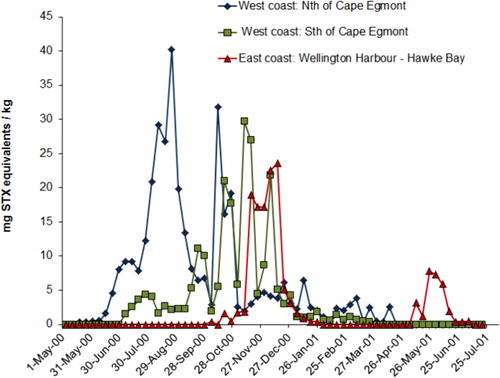
In general, the appearance of G. catenatum in water samples was concurrent with, and often a predictor of, the appearance of PST in shellfish. However, a close correlation between cell numbers and shellfish toxicity cannot be expected on exposed coasts where water sampling is difficult. Along much of the affected coastline, most of the water samples were collected within the surf zone and are therefore not truly representative of cell abundance in the adjacent water column. Nevertheless, cell numbers > 1.0 × 103 cells/litre were common, while at any given site the cell numbers in surf zone samples could vary widely (e.g. from undetectable to 2.7 × 105 cells/litre) in subsequent weeks at one location.
The northern extent of shellfish contamination was the north end of Ninety Mile Beach. Evidence from sampling stations at Spirits Bay and Parengarenga () showed that the bloom did not spread around Cape Reinga to affect the northeastern coast. The bloom spread steadily southwards along the west coast and, by mid-June, it had reached a point just north of Cape Egmont. One week later, shellfish on beaches south of Cape Egmont were showing contamination and, by late August, it had reached Porirua near the southern tip of the North Island. By late September, contamination appeared in Wellington Harbour. From Wellington its northward passage up the east coast was rapid, probably assisted by the north flowing Wairarapa coastal current, until it appeared in Hawke Bay in early November. By mid-November high levels of PST (> 10.0 mg STX equivalent/kg) were detected in this area (). From mid-September to early December 2000, G. catenatum cells were often observed in Cook Strait and in waters around the offshore margins of the Marlborough Sounds. No cells were seen within the major mussel farming area of Pelorus Sound. Because of the discovery of low numbers (500 cells/litre) of G. catenatum cells in the water and resting cysts in oyster cages and on mussel ropes in Port Underwood and Tory Channel in late September 2000, the industry placed a voluntary ban on the movement of spat and mature product from these areas. No PST was detected in shellfish and, fortunately, G. catenatum populations failed to become established in these areas. After surveys of water, sediments and growing structures in Port Underwood did not detect any sign of G. catenatum motile cells or live cysts, the ban was lifted in late January 2001.
Gymnodinium catenatum blooms in 2003
Gymnodinium catenatum cells reappeared again in a seawater sample from Kawhia Harbour (west coast of the North Island) at the end of May 2003, and PST appeared in mussels at Mohakatino (North Taranaki) in late June. There were two bloom episodes in the north Taranaki region in 2003, the first peaking in early August and the second in mid-November (). It is possible this was due to inshore/offshore excursions of bloom-affected waters. Data on the spread of the bloom from this region is less comprehensive than that collected in 2000 because there were fewer sites, sampled less frequently. However, a pattern of a gradual intensification of the bloom northwards into the Manukau Harbour and along the northwest coast of Northland was apparent (). High levels of toxicity (22.4 mg STX equivalents/kg) occurred in shellfish from Hui Bank, Manukau Harbour on 17 October 2003. By mid-November 2003, PST (10 mg STX equivalents/kg) were found in tuatua from Ninety Mile Beach. Low numbers of G. catenatum cells were observed in Wellington Harbour in mid-October and low levels of toxin were detected in shellfish from lower North Island sites (Foxton Beach, Paraparaumu, Porirua) in October, although the bloom did not reach the intensity of the one in 2000 in this region. On 3 May 2003, G. catenatum cells (6.4 × 103 cells/litre) were observed in seawater samples from Ohope Beach, Bay of Plenty. Gymnodinium catenatum persisted in samples from this location until 31 May 2003 and, on one occasion (17 May 2003), was also seen in samples from Te Kaha in the eastern bay. This was the first record of a bloom (albeit a minor one) of G. catenatum in the Bay of Plenty, although some cells had previously been discovered in a water sample taken from the Port of Tauranga on 3 December 2000 (Taylor & MacKenzie Citation2001). Gymnodinium catenatum was present at a maximum cell density of 2.5 × 104 cells/litre in samples from Pania Reef (Hawke Bay) in May 2003, where it persisted until late June. Low cell numbers were also seen in samples from other east coast sites (Tolaga Bay in the north to Waimarama in the south) at that time.
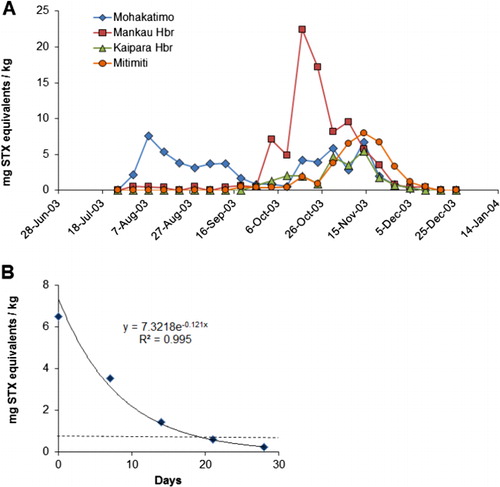
Gymnodinium catenatum in Hawke Bay
A resource consent has been granted to Napier Mussels Ltd for the development of a large (2548 ha) offshore marine farm in Hawke Bay. The development is still in an experimental phase, but it is hoped it will be an important economic opportunity for local iwi (Ngāti Kahungunu) who are major shareholders. Because of the history of G. catenatum blooms in this region (), it is important that this risk is recognised. The appearance of G. catenatum in this area in 2000, and its reappearance for several years thereafter, suggests that for a few years the dinoflagellate established a reservoir of cysts that led to the subsequent blooms. However, the dinoflagellate apparently did not become permanently entrenched in this region, as the last cells were observed in monitoring samples from Hawke Bay in 2007.
Harvest closures due to Gymnodinium catenatum
There have been relatively few closures of commercial mussel-growing areas due to G. catenatum; however, extensive areas of the North Island coast were closed to recreational harvesting on several occasions during 2000–2004. After a PST contamination event the New Zealand Marine Biotoxin Management Plan specifies that there has to be two consecutive weeks of PST levels below the regulatory action level of 0.8 mg STX equivalents/kg before an area can be reopened again for harvesting. To obtain an indication of how long Greenshell™ mussel cultivation areas may be closed if they become contaminated with G. catenatum toxins, the duration of harvesting closures for wild Greenshell™ mussels in various North Island areas are tabulated (). An annual closure period of 70 days (about the average for Greenshell™ mussels) in a major growing area such as Pelorus Sound would have a significant economic impact. The toxins produced by G. catenatum are water soluble and are rapidly released from mussel tissues with a half-life of about six days (). This is much shorter than the lipid soluble marine biotoxins (e.g. yessotoxin) that are slowly eliminated from mussels with half-lives of up to 50 days (MacKenzie et al. Citation2002). From the clearance curve in the approximate depuration time to reach the 0.8 mg STX equivalents/kg level can be calculated according to the equation:
Table 2 Examples of the duration of Greenshell™ mussels harvest closuresFootnotea as a result of contamination by PSP-toxins from G. catenatum.
Sampling from commercial shipping during Gymnodinium catenatum blooms
In September 2000, as the bloom was rapidly spreading southwards along the Taranaki coast, and was approaching the main mussel growing areas within the Marlborough Sounds, a water sampling programme was initiated utilising two of the Cook Strait ferries (Strait Shipping Ltd). Routine sampling of surface waters on the Wellington to Nelson and Wellington to Picton routes of the MV Straitsman and MV Suilven respectively, commenced in mid-September 2000 and were carried out en route every two to three days until March 2001. Due to the reappearance of G. catenatum in Hawke Bay in May 2002, sampling recommenced at weekly intervals on the MV Straitsman between Wellington and Nelson on 7 May 2002 and continued until 13 August 2002. Samples were collected using a small bucket on a rope at approximately 10–12 km intervals along each ship's route while the vessels were underway (). This sampling proved useful in rapidly providing data on the distribution of G. catenatum cells in offshore waters and in the vicinity of the Marlborough Sounds (; –).
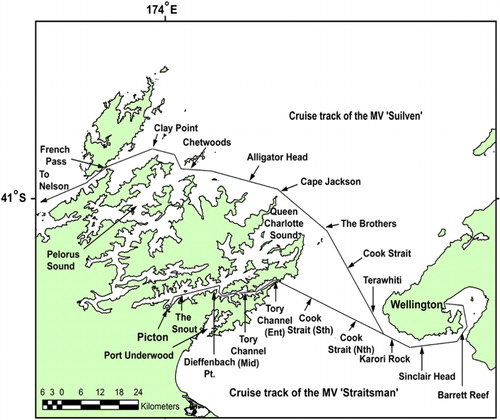
Table 3 Gymnodinium catenatum cell counts in selected samples collected by the MV Straitsman en route from Wellington to Nelson.
Table 4 Gymnodinium catenatum cell counts on selected dates from samples collected by the MV Suilven en route from Wellington to Picton.
In the samples collected from the MV Straitsman on its voyages between Wellington and Nelson, the highest cell numbers were usually found in Wellington Harbour and from mid-Cook Strait eastwards. High numbers were also frequently found off Cape Jackson and the Brothers Islands on the western side of Cook Strait (; ). Cell numbers peaked on 27 September 2000 with 2.0 × 104 cells/litre at Barrett Reef, 9.0 × 103 cells/litre at mid-Cook Strait and 5.0 × 103 cells/litre at the Brothers Islands. Lower numbers of cells were found on almost every sampling occasion (38) on the eastern side of the Strait from 13 September until 29 December 2000, after which no cells were observed. On a few occasions, cells were detected (4–40 cells/litre) in the western outer Sounds region, in the vicinity of the Chetwood Islands and French Pass. On five occasions (11, 13 and 20 October, 6 and 13 December 2000) cells were seen in samples to the west of D'Urville Island and French Pass in Tasman Bay. On 11 October 2000 low numbers of cells were observed in samples from Cape Soucis and off Nelson in Tasman Bay. In the samples collected from the MV Suilven on its voyages between Wellington and Picton (; ), cells were frequently found in all samples from Wellington Harbour across the Strait and throughout the length of Tory Channel as far as Dieffenbach Point. Moderate cell numbers (1.0–9.0 × 103 cells/litre) were sometimes seen in samples from mid-Cook Strait, though the usual pattern was for higher numbers nearer the North Island coast. A small number of cells were also seen in samples from inside Picton Harbour (1 and 3 November 2000).
On two occasions (16–18 August 2000 and 2–3 September 2000) sampling was carried out from the coastal container vessel, MV Spirit of Resolution (Pacifica Shipping Ltd). This vessel was transiting between the Port of Nelson and the Manukau Harbour (Onehunga), and between Onehunga and Lyttelton along the North Island west coast, through the Cook Strait and down the east coast of the South Island. Samples were collected at one hour (c. 15 km) intervals for the duration of the voyages (20–32 h). These are the only data that give some idea of the offshore extent of the 2000–2001 G. catenatum bloom. On both occasions, the highest numbers of cells were observed on the Manukau Harbour bar (6.0–15.0 × 103 cells/litre), but there were also moderate numbers of cells (> 1.0 × 103 cells/litre) in surface waters at all sites sampled across the North Taranaki Bight, up to at least 70 km offshore (). It is likely that cell numbers were much higher beneath the surface at these sites. These data shows there was an abundant and very widespread population of G. catenatum, extending well offshore north of Cape Egmont, that was more closely confined to the coast south of this point.
Gymnodinium catenatum cysts in seafloor sediments
A survey of surface sediments at various locations, including the major ports, around the New Zealand coast was carried out between October 2000 and February 2001 (). This showed that G. catenatum cysts were widespread along the North Island west coast. High numbers of cysts were found in sediment samples collected offshore of Ninety Mile Beach, but none were detected in any samples collected from 10 intertidal sites. All sediment samples collected from the Kaipara Harbour contained cysts. Cysts were found at a number of sites in the Manukau Harbour, the iron sand loading terminal at Taharoa, and in Port Taranaki (New Plymouth). No cysts were found in any samples from the northeast coast of the North Island (Whangaroa Harbour, Bay of Islands, Mahurangi Harbour), although they were detected in a sample from Port Napier (Hawke Bay) and Wellington Harbour. No cysts were found at any South Island locations. Irwin et al. (Citation2003) enumerated G. catenatum cysts and dated (210Pb) sediment strata in cores from the Manukau, Hokianga and Wellington harbours to attempt to answer the question of whether this species had recently arrived in New Zealand or was indigenous. The results of their study demonstrated that G. catenatum had been present in the west coast harbours since at least the late 1930s, but that it was probably a new arrival in Wellington Harbour.
Table 5 The abundance of G. catenatum resting cysts in surface sediments throughout the North and South Islands, October 2000–February 2001 (data from Taylor & Mackenzie Citation2001).
Gymnodinium catenatum risk assessment
Based on a knowledge of the distribution of motile cells and resting cysts of G. catenatum in 2000–2003, an assessment of the risk of blooms in the major shellfish growing areas of the country was made (–). There is a high risk that blooms will again develop on the North Island west coast and affect shellfish aquaculture in this region (). Also, because this region is still the major source of juveniles for other areas (Marlborough, Coromandel, Stewart Island), blooms in this region present a risk to other aquaculture zones that may not be directly affected. Apart from the Marlborough Sounds, the risk of the natural appearance of G. catenatum in South Island waters is probably low ().
Table 6 The risk of the natural development of G. catenatum blooms in North Island shellfish growing areas.
Table 7 The risk of the natural development of G. catenatum blooms in South Island mussel growing areas.
Gymnodinium catenatum cysts in ‘Kaitaia spat’
The New Zealand mussel industry is critically dependent on natural spat falls on drift algae cast up on Ninety Mile Beach (Northland) for around 80% of its seed. For many years, this material, colloquially known as ‘Kaitaia spat’, has provided a cheap and convenient source of juveniles, even if at times the supply has been somewhat erratic (Alfaro et al. Citation2004). The potential biosecurity risk of transferring toxic dinoflagellate cysts first arose in the early 1990s when cysts of Alexandrium ostenfeldii were discovered in Kaitaia spat (MacKenzie & Kappa Citation1992). As a result of these findings, and the discovery of PST in surf clams from Ninety Mile Beach in 1993, methods of minimising this risk were investigated (Hayden Citation1993; Hickman et al. Citation1994; Hay et al. Citation1994). In samples of Kaitaia spat collected in June 2000, low numbers (89 cysts/kg weed) of G. catenatum resting cysts were first observed and the industry immediately imposed a voluntary ban on its transport and use. This occurred during a period when mussel farmers would normally be expecting the best spat falls of the year and were preparing to restock their farms. Subsequent examinations revealed very high numbers of cysts (> 40,000 cysts/kg of weed) on some batches of Kaitaia spat and it became obvious that the ban on the use of this material would be long term and could seriously affect the future production and viability of the mussel industry. In response, the New Zealand Mussel Industry Council Ltd (Citation2001) developed a code of practice designed to control mussel spat movement and therefore prevent the transfer of G. catenatum to other parts of the country. The protocols that were developed outlined the monitoring requirements, closure and opening criteria, procedures and responsibilities associated with spat movements between affected and non-affected areas.
For the estimation of the number of cysts in the seaweed containing the mussel spat (Kaitaia spat), the protocol described by Todd (Citation1999) was used. Essentially, this involved thoroughly washing cysts off subsamples of the weed with high pressure water jets, trapping the cysts on screens and counting by microscopy. A practical detection limit for lot testing of Kaitaia spat was set at 100 cysts/kg.
Between June 2000 and January 2001 some very high cyst numbers (a maximum of 40,534 cysts/kg on 10 August 2000) were encountered (). After April 2001 (apart from one sample on 5 June 2001 of 124 cysts/kg) all batches returned nil results (New Zealand Mussel Industry Council Ltd Citation2001). Based on the first risk assessment a conservative opening criteria of 25 clear samples (i.e. containing no cysts) of Kaitaia spat recorded over a period of six weeks was in operation at this time. In July 2002, an analysis of the accumulated data showed that although occasional cysts were detectable in spat samples these were always below 100 cysts/kg, there were no increasing or decreasing trends and cysts were clearly at stable background levels. For this reason, the New Zealand Mussel Industry Council Ltd. (Citation2002) amended the opening criteria to 10 samples taken over a period of no less than six weeks with <100 cysts/kg. Because the primary criteria (i.e. no sign of G. catenatum in Northland waters) and secondary criteria (i.e. no spat samples at > 100 cysts/kg for six weeks) had been met, the decision to open the beach was made on 26 July 2002. In hindsight, by applying the amended more liberal opening criteria, untreated or only primary treated (washed) Kaitaia spat could have been available from early August 2001 onwards. With the return of the G. catenatum bloom on the North Island west coast in 2003 (), Kaitaia spat was again contaminated with cysts and movement control procedures were activated. No further restrictions on the movement of Kaitaia spat have been necessary since, although it is probable that they will need to be invoked again sometime in the future.
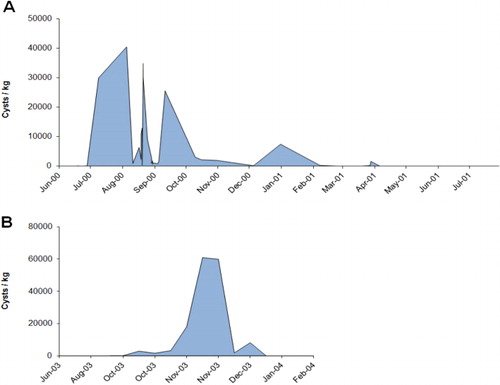
As a consequence of the lack of Kaitaia spat, more effort was put into catching mussel spat in ‘clean’ areas, and there was an intense effort to develop methods of separating the mussel spat from the weed and the dinoflagellate cysts. When considering options for the treatment of Kaitaia spat it was decided in the early stages that attempts to selectively kill the cysts would be futile, although heat treatment remained a viable option for the treatment of oyster spat sticks. Alongside the various Kaitaia spat cleaning techniques that were developed, a rigorous monitoring and quality control programme was put in place. Some decontamination methods were successful and several cleansing plants were established. In January 2001, the first cleaned mussel spat originating from Ninety Mile Beach was permitted to be placed in ‘clean’ growing areas of the Marlborough Sounds.
The North Island oyster industry was also affected as many of the best oyster spat catching areas are within the west coast estuaries, such as the Kaipara Harbour where G. catenatum was present. From here, spat is routinely transported to ongrowing areas on the east coast. In June, when high numbers of cysts were seen on oyster nursery sticks from the Kaipara Harbour, this transfer was immediately halted. Mussel spat catching lines in Aotea Harbour were also found to be contaminated with cysts.
Aside from effects on the molluscan shellfish industry, the bloom also affected the spiny lobster (Jasus edwardsii) fishery when it was found that PST with the characteristic toxin analogue profiles of G. catenatum were present in the digestive gland of the animals (Anon. Citation2001). Edible portions such as the tail flesh remained free of contamination, but because most product is exported as whole live animals, a precautionary ban was placed on this trade. After analyses showed that the toxin burden in whole crayfish was small, and having taken advice from overseas authorities with experience of PST in crustaceans, this ban was eventually lifted. A monitoring procedure has been developed by the New Zealand Rock Lobster Industry Council should it be necessary to again limit exports in the future.
The New Zealand and Australian abalone fisheries have also faced similar questions regarding the potential for PST contamination of wild abalone harvested during Alexandrium spp. and G. catenatum blooms. Low levels of PST have been found in the digestive gland of New Zealand pāua (Haliotis iris) during G. catenatum blooms (MPI biotoxin data) and in the black-lipped abalone (Haliotis rubra) from areas off the eastern coast of Tasmania (Abalone Council Australia Ltd Citation2013). The risk to consumers is probably low, but a joint New Zealand and Australian industry research project is currently in progress to provide data that will assist in the future management of this issue.
Toxin profiles in New Zealand Gymnodinium catenatum
Cultured cells of New Zealand G. catenatum isolates predominately produce toxin analogues within the low potency N-sulfocarbamoyl group, mainly C3,4 and with smaller amounts of C1,2, GTX5 and GTX6 (). The more potent GTX1,4 analogues only make up a small proportion of the toxin but contribute substantially to the total toxicity. The toxin profiles of New Zealand G. catenatum isolates are very similar to those in the same species elsewhere in the world (Costa et al. Citation2014) and contain a comprehensive suite () of the low toxicity hydroxybenzoate analogues (‘GC-toxins’) that are unique to this species (Negri et al. Citation2003, Citation2007). Because of the lack of analytical standards for the GC-toxins these compounds cannot be accurately quantified as yet.
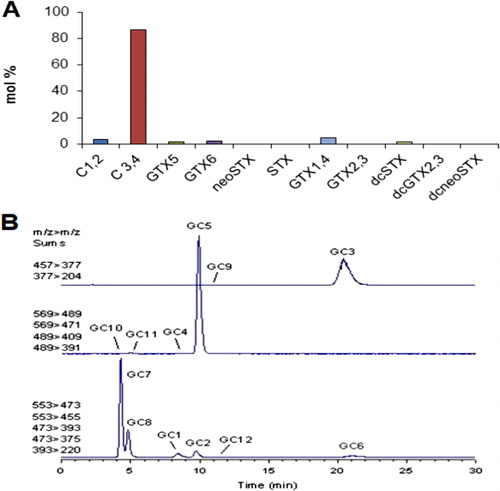
Effects on human health
There is no doubt that human poisoning can occur due to the consumption of shellfish contaminated with G. catenatum toxins (e.g. Rodrigues et al. Citation2012). However, it proved difficult for health agencies to accurately determine the effects of the 2000–2003 blooms on public health because only a small number of cases meeting the case definition of ‘PSP intoxication’ were reported (Beauchamp Citation2000). In some areas, public health warnings were probably effective in preventing most people from gathering and eating shellfish. In other areas, such as Northland, where levels of toxicity have been highest and where shellfish are an important item of the diet, these warnings may have been ignored by some sections of the community. It was suspected (T. Beauchamp, Northland Health, pers. comm. November 2000) that there were unreported cases of mild poisoning, but had more serious cases occurred they would almost certainly have come to the attention of the public health system. The predominance of low specific toxicity N-sulfocarbamoyl toxins in the algae and shellfish may explain the few reports of human illness. Because most of the areas affected by the G. catenatum blooms in 2000–2003 were outside the main commercial areas, most of the financial burden for the extra monitoring was borne by central government via the Ministry of Health (MOH). The MOH commissioned a review of the options (Hay et al. Citation2000) to rationalise the existing monitoring programme in an effort to reduce these costs. It looked at retaining a viable system that still minimised the risk of poisoning to consumers of non-commercial shellfish. This report identified key primary sampling sites based on an analysis of data collected during the bloom and made recommendations that reduced the number of sampling sites and the frequency of sampling, and substituted phytoplankton monitoring for flesh testing at a number of sites. As a consequence of these cut backs, the detailed extensive spatial coverage that provided a near real-time picture of the spread of the bloom in 2000–2001 will probably not be available when there is a repetition of such a large-scale event in the future.
Conclusions
The organisms responsible for producing PSP in New Zealand are the same species (Gymnodinium catenatum, Alexandrium catenella/tamarense and Alexandrium minutum) that are notorious in temperate environments elsewhere in the world. Contamination by PST has not been a major impediment to shellfish aquaculture in New Zealand, but there have been several bloom events that have had important economic effects. Large-scale aquaculture developments in new areas with known histories of PST contamination, such as the Bay of Plenty and Hawke Bay, will need to work around this problem. There is also the possibility that A. catenella may become a chronic problem in the Top of the South region in the future, if it extends its range beyond QCS. The prohibition on the relocation of Kaitaia spat during the 2000–2003 G. catenatum blooms had the greatest effect on the industry as a whole. It is probable that a similar event will reoccur at some time in the future and the industry needs to be prepared for this. New biotoxin phenomena, such as the appearance of tetrodotoxin (TTX) in sea slugs in Auckland in 2010 (McNabb et al. Citation2010), occur in New Zealand from time-to-time. In this case the precise biosynthetic origin of the toxin is still unknown. There is no such uncertainty with regard to the PSP-causing toxins. The dinoflagellates that produce the toxins are well known and we now know how spatially extensive or constrained blooms can be in specific locations and, in general, how these will play out over time. We are developing a good idea of the major biological and environmental drivers of blooms in some locations (e.g. Opua Bay), but our knowledge is inadequate in other important areas (e.g. Bay of Plenty). There is generally a good relationship between cell numbers in the plankton and levels of toxicity in shellfish if sampling is carried out rigorously at appropriate locations. Phytoplankton monitoring usually gives ample early warning of the appearance of toxicity in shellfish. The cessation of MBA testing for PST in 2010 was an important step forward for the industry as this was becoming increasingly untenable on animal welfare grounds. Technical improvements to test methods (e.g. the adoption of mass spectrometric analysis and molecular methods for cell and cyst enumeration) can be expected in the future, which will make these even more robust, accurate and cost effective than they are at present. The recent serious PSP incident in the Bay of Plenty, and the Tasmanian shellfish industry's experience with the export of contaminated products, are reminders that toxic phytoplankton and shellfish biotoxin monitoring is essential for quality assurance and the future of shellfish growing in New Zealand.
Acknowledgements
Thanks to the Ministry for Primary Industries (MPI) for use of the public health shellfish biotoxin monitoring data, Aquaculture NZ (AQNZ) for permission to use information on the Kataia spat analyses, and the Marlborough Sounds Shellfish Quality Programme (MSQP) for the use of the Queen Charlotte Sound monitoring data. Thanks to Trieste Ngawhika, Toi Te Ora Public Health Service for the Bay of Plenty closure data, Straits Shipping Company for carrying out the Cook Strait sampling and Dr Michael Quilliam, Institute for Marine Biosciences National Research Council Canada for the mass spectrometer analysis of the G. catenatum GC-toxins. Thanks to Cherie Johansson at Cawthron for her thorough editorial review of the manuscript. The collection of other data and the preparation of this review was funded through the MBIE Research for Industry ‘Seafood Safety Programme’; MBIE contract CAWX1317.
References
- Abalone Council Australia Ltd 2013. Progress report; CRC project 2010/737: Marine biotoxins and market access. http://abalonecouncil.com.au/wp-content/uploads/2010/05/ACA-project-update-October-2013.pdf (accessed October 2013).
- Aguilera-Belmonte A, Inostroza I, Franco JM, Riobo P, Gomez PI 2011. The growth, toxicity and genetic characterisation of seven strains of Alexandrium catenella (Whedon & Kofoid) Balech 1985 (Dinophyceae) isolated during the 2009 summer outbreak in southern Chile. Harmful Algae 12: 105–112. 10.1016/j.hal.2011.09.006
- Alfaro AC, Jeffs AG, Creese RG 2004. Bottom-drifting algal/mussel spat associations along a sandy coastal region in northern New Zealand. Aquaculture 241: 269–290. 10.1016/j.aquaculture.2004.07.029
- Anon. 2001. Toxic algal blooms: challenging the NZ rock lobster industry. Seafood New Zealand 9: 10–11.
- AOAC 1990. Official Method 959.08. In: Hellrich K ed. Paralytic shellfish poison biological method. 15th edition. Arlington, VA, Association of Official Analytical Chemists. Pp. 881–882.
- APHA 1970. Part V bioassay for shellfish toxins. In: Neter E ed. Recommended procedures for the examination of seawater and shellfish. New York, American Public Health Association. Pp. 57–66.
- Baden D, Bean JA, Fleming LE 1995. Marine toxins In: de Wolff FA ed. Handbook of clinical neurology: intoxications of the nervous system part ii. Natural toxins and drugs. Amsterdam, Elsevier Press. Pp. 141–175.
- Balech E 1995. The genus Alexandrium halim (Dinoflagellata). Cork, Ireland, Sherkin Island Marine Station, Sherkin Island Co. 151 p.
- Beauchamp T 2000. PSP regulatory levels and Gymnodinium catenatum – its relevance, concrete interpretation and the risk to science. Proceedings of the Marine Biotoxin Science Workshop No 14, 24 November 2000. Wellington, Ministry of Agriculture and Forestry. Pp. 33–51.
- Benavides HL, Pardo L, Diaz S, Carreto JI 1995. An exceptional bloom of Alexandrium catenella in the Beagle Channel, Argentina. In: Lassus P, Arzul G, Erard-Le Denn E, Gentien P, Marcaillou-Le Baut C eds. Harmful marine algal blooms. Paris, Lavoisier Intercept Ltd. Pp. 113–119.
- Blackburn SI, Hallegraeff GM, Bolch CJ 1989. Vegetative reproduction and sexual life cycle of the toxic dinoflagellate Gymnodinium catenatum from Tasmania Australia. Journal of Phycology 25: 577–590. 10.1111/j.1529-8817.1989.tb00264.x
- Busby P, Seamer C 2004. Shellfish safety and quality as a source of competitive advantage – the New Zealand experience. In: Henshilwood K, Deegan B, McMahon T, Cusack C, Keaveney S, Silke J et al. eds. Molluscan shellfish safety proceedings of the 5th International Conference on Molluscan Shellfish Safety, Galway, Ireland, 14–18 June. Pp. 424–428.
- Campbell A, McLeod C, Pointon A, Hudson, D, Nicholls C 2013. The 2012–13 paralytic shellfish toxin event in Tasmania associated with the dinoflagellate alga, Alexandrium tamarense. A SafeFish Review. FRDC Project 2012/060. Adelaide: Fisheries Research and Development Corporation and South Australian Research and Development Institute. http://www.frdc.com.au/research/Documents/Final_reports/2012-060-DLD.pdf (accessed May 2014)
- Cembella AD, Quilliam MA, Lewis NI, Bauder AG, Dell'Aversano C, Thomas K et al. 2002. The toxigenic dinoflagellate Alexandrium tamarense as the probable cause of mortality of caged salmon in Nova Scotia. Harmful Algae 1: 313–325. 10.1016/S1568-9883(02)00048-3
- Cembella AD, Sullivan JJ, Boyer GL, Taylor FJR, Andersen RJ 1987. Variation in PSP toxin composition within the Protogonyaulax tamarensis/catenella species complex: red tide dinoflagellates. Biochemical Systematics and Ecology 15: 171–186.
- Chang FH 2004. A review of the currently monitored toxigenic phytoplankton species in New Zealand. A report for the New Zealand Food Safety Authority. NIWA Client Report: WLG2004–80. Wellington, NIWA. 47 p.
- Chang FH, Anderson DM, Kulis DM, Till DG 1997. Toxin production of Alexandrium minutum (Dinophyceae) from the Bay of Plenty New Zealand. Toxicon 35: 393–409.
- Chang FH, MacKenzie L, Till D, Hannah D, Rhodes L 1995. The first toxic shellfish outbreaks and the associated phytoplankton blooms in early 1993 in New Zealand. In: Lassus P, Erard E, Gentien P, Marcaillou C eds. Harmful marine algal blooms. Paris, Lavoisier Science Publishers. Pp. 145–150.
- Chang, FH, Sharples J, Grieve JM 1996. Temporal and spatial distribution of toxic dinoflagellates in the Bay of Plenty, New Zealand during the early 1993 toxic shellfish outbreaks. In: Yasumoto T, Oshima Y, Fukuyo Y eds. Harmful and toxic algal blooms. Paris, Intergovernmental Oceanographic Commission of UNESCO. Pp. 235–238.
- Costa PR, Moita T, Rodrigues SM 2014. Estimating the contribution of N-sulfocarbamoyl paralytic shellfish toxin analogs GTX6 and C3+4 to the toxicity of mussels (Mytilus galloprovincialis) over a bloom of Gymnodinium catenatum. Harmful Algae 31: 35–40. 10.1016/j.hal.2013.09.009
- Cox AM, Shull DH, Horner RA 2008. Profiles of Alexandrium catenella cysts in Puget Sound sediments and the relationship to paralytic shellfish poisoning events. Harmful Algae 7: 379–388. 10.1016/j.hal.2007.01.006
- de Salas MF, van Emmerik, MJ, Hallegraeff GM, Negri AP, Vaillancourt RE, Bolch CJS 2001. Toxic Australian Alexandrium dinoflagellates: introduced or indigenous? In: Hallegraeff GM, Bolch CJS, Blackburn S, Lewis RJ, eds. Harmful algal blooms 2000. Paris, UNESCO. Pp. 214–217.
- EFSA (European Food Safety Authority) 2009. Scientific opinion of the panel on contaminants in the food chain on a request from the European Commission on marine biotoxins in shellfish-saxitoxin group. The EFSA Journal 1019: 1–76.
- ESR (Environmental Science Research) 2012. Notifiable disease surveillance report, December 2012. http://www.surv.esr.cri.nz/PDF_surveillance/MthSurvRpt/2012/201212DecRpt.pdf (accessed May 2014)
- FAO/WHO 2006. Food Standards Programme Codex Committee on fish and fisheries products, 28th session, Beijing, China, 18–22 September. www.codexalimentarius.org/input/download/report/666/al30_18e.pdf (accessed May 2014)
- Feifel KM, Moore SK, Horner RA 2012. An Alexandrium spp. cyst record from Sequim Bay, Washington State, USA, and its relation to past climate change. Journal of Phycology 48: 550–558. 10.1111/j.1529-8817.2012.01175.x
- Fuentes C, Clement A, Aguilera A 2008. Summer Alexandrium catenella bloom and the impact on fish farming in the XI Aysen region Chile. In: Moestrup Ø, Doucette G, Enevoldsen H, Godhe A, Hallegraeff G, Lewis J et al. eds. Proceedings of the 12th International Conference on Harmful Algae. Copenhagen, Denmark, International Society for the Study of Harmful Algae. Pp. 183–186.
- Hallegraeff GM, Blackburn SI, Doblin MA, Bolch CJS 2012. Global toxicology, ecophysiology and population relationships of the chain forming dinoflagellate Gymnodinium catenatum. Harmful Algae 14: 130–143. 10.1016/j.hal.2011.10.018
- Harwood DT, Boundy M, Selwood AI, van Ginkel R, MacKenzie L, McNabb PS 2013. Refinement and implementation of the Lawrence method (AOAC 2005.06) in a commercial laboratory: assay performance during an Alexandrium catenella bloom event. Harmful Algae 24: 20–31. 10.1016/j.hal.2013.01.003
- Hay B, Grant C, Beauchamp T 2000. A review of the marine biotoxin monitoring for PSP toxicity arising from the current Gymnodinium catenatum event. A report to the Ministry of Health, December 2000. Auckland, AquaBio Consultants Ltd. 47 p.
- Hay BE, Grant CM, Homan LE 1994. Experimental investigation of techniques used to separate mussel seed (Perna canaliculus) from ‘Kaitaia spat’. A report for the Mussel Industry Council by AquaBio Consultants Ltd, July 1994. Auckland, AquaBio Consultants Ltd. 17 p.
- Hayden B 1993. Dinoflagellate cysts in Kaitaia spat and Marlborough Sounds sediments. A report to MAFQual by MAF Fisheries, 27 September. Wellington, MAF Fisheries. 10 p.
- Hickman RW, Redfearn P, Illingworth J, Murray-Brown VH 1994. Minimum risk methods for transferring ‘Kaitaia spat’. A report to the Kaitaia Spat Action Group of the Mussel Industry Council. MAF Fisheries, 3 February. Wellington, Aquaculture Research Centre. 9 p.
- Holland PT, McNabb P, Van Ginkel R, Selwood A 2010a. Saxitoxins in seafood: assessment of test methods to replace the PSP mouse bioassay. Cawthron Report no. 1717. Nelson, Cawthron Institute. 29 p.
- Holland PT, Van Ginkel R, Selwood AI, McNabb PS 2010b. Validation of the Lawrence Method for screening saxitoxins in shellfish. A report for the New Zealand Food Safety Authority. Cawthron Report no. 1738. Nelson, Cawthron Institute. 12 p. + appendices.
- Irwin A, Hallegraeff GM, McMinn A, Harrison J, Heijnis H 2003. Cyst and radionuclide evidence demonstrate historic Gymnodinium catenatum dinoflagellate populations in Manakau and Hokianga harbours, New Zealand. Harmful Algae 2: 61–74. 10.1016/S1568-9883(02)00084-7
- Kiermeier A, McLeod C, Sumner J 2009. ‘Marine biotoxins in shellfish-saxitoxin group: scientific opinion of the panel on contamination in the food chain’ by the European Food Safety Authority (EFSA). A review by the South Australian Research and Development Institute (SARDI). September 2009. p. 11. http://safefish.com.au/wp-content/uploads/2013/03/Marine-Toxin-Regulatory-levels.pdf (accessed May 2014)
- Kim C-J, Kim C-H, Sako Y 2005. Paralytic shellfish poisoning toxin analysis of the genus Alexandrium (Dinophyceae) occurring in Korean coastal waters. Fisheries Science 71: 1–11. 10.1111/j.1444-2906.2005.00924.x
- Krock B, Seguel CG, Cembella AD 2077. Toxin profile of Alexandrium catenella from Chilean coast as determined by liquid chromatography with fluorescence detection and liquid chromatography coupled with tandem mass spectrometry. Harmful Algae 6: 734–744. 10.1016/j.hal.2007.02.005
- Lawrence JF, Niedzwiadek B 2001. Quantitative determination of paralytic shellfish poisoning toxins in shellfish by using pre-chromatographic oxidation and liquid chromatography with fluorescence detection. Journal of AOAC International 84: 1099–1108.
- Lawrence JF, Niedzwiadek B, Menard C 2004. Quantitative determination of paralytic shellfish poisoning toxins in shellfish using pre-chromatographic oxidation and liquid chromatography with fluorescence detection: inter-laboratory study. Journal of AOAC International 87: 83–100.
- Lawrence JF, Niedzwiadek B, Menard C 2005. Quantitative determination of paralytic shellfish poisoning toxins in shellfish using pre-chromatographic oxidation and liquid chromatography with fluorescence detection: collaborative study. Journal of AOAC International 88: 1714–1732.
- Lilibeth NM, Yunyun Z, Huan Z, Senjie L, 2012. Phylogenetic analysis guided by intra-genomic SSU rDNA polymorphism refines classification of ‘Alexandrium tamarense’ species complex. Harmful Algae 16: 35–48. 10.1016/j.hal.2012.01.002
- Lilly EL, Halanych KM, Anderson DM 2007. Species boundaries and global biogeography of the Alexandrium tamarense complex (Dinophyceae). Journal of Phycology 43: 1329–1338. 10.1111/j.1529-8817.2007.00420.x
- Luthy J 1979. Epidemic paralytic shellfish poisoning in Western Europe, 1976. In: Taylor, DL, Seliger, HH eds. Toxic dinoflagellate blooms. New York, Elsevier. Pp. 15–22.
- MacKenzie AL 1989. Surveys for the detection of paralytic shellfish poisoning in the Marlborough Sounds December 1985 – February 1989. New Zealand Journal of Marine and Freshwater Research 24: 75–80. 10.1080/00288330.1990.9516403
- MacKenzie L, Adamson J 2000. The biology and toxicity of Gymnodinium catenatum: an overview with observations from the current bloom. Proceedings of the Marine Biotoxin Science Workshop no. 14, 24 November. Wellington, MAF. Pp. 18–27.
- MacKenzie L, Adamson J, White D, Todd K 1996c. Alexandrium catenella and PSP-toxin contamination of shellfish in the Bay of Plenty: progress to date on an investigation of the April/May 1996 bloom. Proceedings of the 6th Marine Biotoxin Science Workshop, 18 October. Wellington, MAF. Pp. 45–53.
- MacKenzie L, Adamson J, Todd K 1997. Alexandrium spp. and associated PSP-toxin contamination of shellfish on the North Island east coast during 1997. In: Proceedings of the 8th Marine Biotoxin Science Workshop, 10 November. Wellington, MAF.
- MacKenzie L, Beauchamp T 2001. Gymnodinium catenatum in New Zealand: a new problem for public health and the shellfish industry. Cawthron Report no. 633. Nelson, Cawthron Institute. 10 p.
- MacKenzie AL, Berkett N 1997. Cell morphology and PSP-toxin profiles of Alexandrium minutum in the Marlborough Sounds, New Zealand. New Zealand Journal of Marine and Freshwater Research 31: 403–409. 10.1080/00288330.1997.9516773
- MacKenzie AL, de Salas M, Adamson J, Beuzenberg, V 2004. The dinoflagellate genus Alexandrium (Halim) in New Zealand coastal waters: comparative morphology, toxicity and molecular genetics. Harmful Algae 3: 71–92. 10.1016/j.hal.2003.09.001
- MacKenzie AL, Harwood T, Boundy M, Smith K, Knight B, Jiang W et al. 2011. An Alexandrium catenella bloom and associated saxitoxin contamination of shellfish, Queen Charlotte Sound, March–June 2011. A report for MAF Food Safety. Cawthron Report no. 1945. Nelson, Cawthron Institute. 38 p. http://www.foodsafety.govt.nz/elibrary/industry/psp-bloom-report-final.pdf (accessed May 2014)
- MacKenzie L, Harwood T, Tonks A, Robinson J, Knight B 2013. Seafood safety risks from paralytic shellfish poisoning dinoflagellate blooms in New Zealand; 2012–2013. A report for the Ministry for Primary Industries (MPI) Food Safety. Cawthron Report 2346. Nelson, Cawthorn Institute. 38 p. http://www.foodsafety.govt.nz/elibrary/industry/seafood-risks-paralytic-shellfish-blooms.pdf (accessed May 2014)
- MacKenzie L, Harwood T, Watts A, Webber S 2012. Alexandrium catenella blooms and associated saxitoxin contamination of shellfish, March to June 2012. A report for the Ministry of Primary Industries Food Safety. Cawthron Report no. 2182. Nelson, Cawthorn Institute. 37 p. http://www.foodsafety.govt.nz/elibrary/industry/alexandrium-catenella-blooms.pdf (accessed May 2014)
- MacKenzie L, Holland P, McNabb P, Beuzenberg V, Selwood A, Suzuki T 2002. Complex toxin profiles in phytoplankton and Greenshell mussels (Perna canaliculus) revealed by LC-MS/MS analysis. Toxicon 40: 1321–1330. 10.1016/S0041-0101(02)00143-5
- MacKenzie, L, Kapa J 1992. Alexandrium spp. in New Zealand and dinoflagellates in Kaitaia spat. A report for the New Zealand Mussel Industry Council. 29 September. Cawthron Report no. 868. Nelson, Cawthron Institute. 8 p.
- MacKenzie AL, Rhodes LL, Till D, Chang FH, Kaspar H, Haywood A et al. 1995a. A Gymnodinium sp. bloom and the contamination of shellfish with lipid soluble toxins in New Zealand, January–April 1993. In: Lassus P, Erard E, Gentien P, Marcaillou C eds. Harmful marine algal blooms. Paris, Lavoisier Science Publishers. Pp. 795–800.
- MacKenzie L, Taylor M. 2004. Risk assessment of the transfer of Kaitaia spat contaminated with Gymnodinium catenatum cysts. A report prepared for the New Zealand Mussel industry Council. Cawthron Report no. 868, January. Nelson, Cawthron Institute. 32 p.
- MacKenzie L, Todd K 2002. Alexandrium camurascutulum sp. nov. (Dinophyceae): a new dinoflagellate species from New Zealand. Harmful Algae 1: 295–300. 10.1016/S1568-9883(02)00045-8
- MacKenzie AL, White D, Adamson J 1996b. Temporal variation and tissue localisation of paralytic shellfish toxins in the New Zealand Tuatua (surf-clam), Paphies subtriangulata. Journal of Shellfish Research 15: 735–740.
- MacKenzie AL, White D, Oshima Y, Kapa J 1996a. The resting cyst and toxicity of Alexandrium ostenfeldii (Dinophyceae) in New Zealand. Phycologia 35: 148–155. 10.2216/i0031-8884-35-2-148.1
- MacKenzie L, White D, Rhodes L, Berkett N, Burke B, Haywood A 1994. Alexandrium and PSP in New Zealand – another chapter in the marine biotoxin saga. Seafood New Zealand 2: 72–25.
- McNabb P, Selwood AI, Munday R, Wood SA, Taylor DI, MacKenzie AL et al. 2010. Detection of tetrodotoxin from the grey side-gilled sea slug Pleurobranchea maculata and associated dog neurotoxicosis on beaches adjacent to the Hauraki Gulf, Auckland, New Zealand. Toxicon 56: 466–473. 10.1016/j.toxicon.2010.04.017
- Moore SK, Mantua NJ, Hickey BM, Trainer VL 2009. Recent trends in paralytic shellfish toxins in Puget Sound, relationships to climate, and capacity for prediction of toxic events. Harmful Algae 8: 463–477. 10.1016/j.hal.2008.10.003
- Mortensen AM 1985. Massive fish mortalities in the Faroe Islands caused by a Gonyaulax excavata red tide. In: Anderson DM, White AW, Baden DG eds. Toxic dinoflagellates. New York, Elsevier Science Publishing. Pp. 165–170.
- Munday R, Reeve J 2013. Risk assessment of shellfish toxins. Toxins 5: 2109–2137. 10.3390/toxins5112109
- Munday R, Thomas K, Gibbs R, Murphy C, Quilliam MA 2013. Acute toxicities of saxitoxin, neosaxitoxin, decarbamoyl saxitoxin and gonyautoxins 1 and 4 and 2 and 3 to mice by various routes of administration. Toxicon 76: 77–83. 10.1016/j.toxicon.2013.09.013
- Negri AP, Bolch CJS, Geier S, Green DH, Park T-G, Blackburn SI 2007. Widespread presence of hydrophobic paralytic shellfish toxins in Gymnodinium catenatum. Harmful Algae 6: 774–780. 10.1016/j.hal.2007.04.001
- Negri AP, Stirling D, Quilliam M, Blackburn S, Bolch C, Burton I et al. 2003. Three novel hydroxybenzoate saxitoxin analogues isolated from the dinoflagellate Gymnodinium catenatum. Chemical Research in Toxicology 16: 1029–1033. 10.1021/tx034037j
- New Zealand Aquaculture Council Inc 2001. An analysis of the risk associated with the transfer of beach harvested seaweed containing mussel spat to mussel farming areas of New Zealand following the Gymnodinium catenatum bloom of 2000/2001. Nelson, New Zealand Aquaculture Council Inc. 8 p. + appendices.
- New Zealand Food Safety Authority (NZFSA) 2007. Data analysis for ‘Right-sizing’ Marine Biotoxin Programme'. NZFSA report, 24 May. Wellington, NZFSA. 17 p.
- New Zealand Mussel Industry Council Ltd (NZMIC) 2001. Mussel industry spat transfer programme. Blenheim, NZMIC. 10 p. + appendices.
- New Zealand Mussel Industry Council Ltd (NZMIC) 2002. National spat transfer programme. Blenheim, NZMIC. 15 p. + appendices.
- Oshima Y 1995. Post-column derivatization liquid chromatographic method for paralytic shellfish toxins. Journal of AOAC International 78: 528–532.
- Rhodes LL, MacKenzie AL, Kaspar HF, Todd KE 2001. Harmful algae and mariculture in New Zealand. ICES Journal of Marine Research 58: 398–403. 10.1006/jmsc.2000.1023
- Rhodes L, Smith K, Moisan C 2013. Shifts and stasis in marine HAB monitoring in New Zealand. Environmental Science and Pollution Research 20: 6872–6877. 10.1007/s11356-012-0898-9
- Rodrigues SM, de Carvalho M, Mestre T, Ferreira JJ, Coelho M, Peralta R et al. 2012. Paralytic shellfish poisoning due to ingestion of Gymnodinium catenatum contaminated cockles – application of the AOAC HPLC official method. Toxicon 59: 558–566. 10.1016/j.toxicon.2012.01.004
- Shumway SE, Sherman SA, Cembella AD, Selvin R 1994. Accumulation of paralytic shellfish toxins by surf-clams Spisula solidissima (Dillwyn 1797) in the Gulf of Maine: seasonal cages, distribution between tissues, and notes on feeding habits. Natural Toxins 2: 236–251. 10.1002/nt.2620020413
- Taylor MD, MacKenzie L 2001. Delimitation survey of the toxic dinoflagellate Gymnodinium catenatum in New Zealand. A report prepared for the New Zealand Ministry of Fisheries. Cawthron Report no. 661, July. Nelson, Cawthron Institute. 32 p.
- Tobin ED, Horner RA 2011. Germination characteristics of Alexandrium catenella cysts from surface sediments in Quartermaster Harbor, Puget Sound, Washington, USA. Harmful Algae 10: 216–223. 10.1016/j.hal.2010.10.002
- Todd K 1997. Management of environmental risk for shellfish harvesting. A report for the NZ Fishing Industry Board. Cawthron Report no. 406. Nelson, Cawthron Institute. 132 p.
- Todd K 1999. Cawthron quality system manual 16. Method 70.001. Nelson, Cawthron Institute.
- Trainer VL, Eberhart B-TI, Wekell JC, Adams NG, Hanson L, Cox F et al. 2003. Paralytic shellfish poisoning in Puget Sound Washington State. Journal of Shellfish Research 22: 213–223.
- Trusewich B, Sim J, Busby P, Hughes C 1996. Management of marine biotoxins in New Zealand. In: Yasumoto T, Oshima Y, Fukuyo Y eds. Harmful and toxic algal blooms. Paris, Intergovernmental Oceanographic Commission of UNESCO. Pp. 27–30.
- Varela D, Paredes J, Alves-de-Souza C, Seguel M, Sfeir A, Frangópulos M 2012. Intraregional variation among Alexandrium catenella (Dinophyceae) strains from southern Chile: morphological, toxicological and genetic diversity. Harmful Algae 15: 8–18. 10.1016/j.hal.2011.10.029