ABSTRACT
Many fish species rely on estuarine nursery habitats as they transition to adult life stages. Quantifying nursery value, however, requires identification of the life stages (often small and short-lived) that utilise nursery habitats, and survey methods that provide comparable estimates across habitats. We focused our surveys on post-settlement snapper (Chrysophrys auratus) using video camera deployments across habitats within a northeastern New Zealand harbour. Post-settlement snapper abundance was higher among structured habitat types relative to bare sediments, with the type of structured habitat not influential. The exception appeared to be for reef habitat (although sampling was limited). Reef sites were structurally complex, but largely inhabited by older life stages (snapper and other fish species). Overall, nursery value for snapper appears to be connected to structure, rather than structure type. This result emphasises the importance of a broadened scope to coastal fishery management, one that incorporates not just fish extraction, but also habitat maintenance or restoration.
Introduction
Many marine fish require estuarine nursery habitats. They harbour higher densities of juvenile life stages, enhance growth and survival, and consequently provide a disproportionate contribution to adult populations (Beck et al. Citation2001). Because of the specific fish–habitat relationships that provide these benefits, nursery habitats represent a critical requirement for some fish species. From a fisheries and coastal management perspective, it is therefore important that nursery habitats are identified and the broader ecosystem processes underpinning their values are understood and maintained (Sheaves et al. Citation2015; Whitfield & Pattrick Citation2015). A particular habitat that has received a large amount of attention as a juvenile fish nursery is seagrass (Beck et al. Citation2001; Heck et al. Citation2003). The predominance of nursery research conducted on seagrass is probably due to the high densities of juvenile fishes associated with this habitat (see references within Beck et al. Citation2001; Heck et al. Citation2003). Indeed, some studies have observed large-scale increases in juvenile fish density associated with natural recovery of seagrass beds (Warren et al. Citation2010), while others have demonstrated that seagrass beds positively influence the density of adult fish on nearby reefs by acting as a source of recruits (Nagelkerken et al. Citation2002; Dorenbosch et al. Citation2004). While the nursery function of seagrass beds may often be greater compared with unstructured habitats (measured through increased density, survival and growth of juvenile fishes), there is limited evidence that this is also true when seagrass is compared with other structured habitat types (see references within Heck et al. Citation2003).
Previous reviews have suggested that southern hemisphere seagrass beds may have reduced nursery value for juvenile fishes (Butler & Jernakoff Citation2000; Beck et al. Citation2001; Heck et al. Citation2003). A significant amount of research, however, has investigated the nursery function of seagrass in New Zealand (Francis et al. Citation2005, Citation2011; Parsons et al. Citation2013; Morrison et al. Citation2014; Parsons et al. Citation2015). In general, New Zealand seagrass beds possess higher densities and diversity of juvenile fishes compared with bare sediments, although local and regional-scale variables are also influential (Morrison et al. Citation2014). Of the fish captured during these seagrass surveys, the one most likely to meet nursery hypothesis criteria is the highly valued coastal fish, snapper (Chrysophrys auratus) (Parsons et al. Citation2014b).
In order to move towards evaluating the nursery hypothesis for snapper, and understand the relative importance of seagrass as a potential nursery habitat, multiple aspects need to be considered.
First, nursery-occupying species generally have different juvenile and adult habitats. The nursery-occupying stages are often small, short-lived and require specifically focused surveys (Beck et al. Citation2001). In New Zealand, ontogenetic shifts in habitat utilisation have been observed for snapper, with post-settlers <60 mm fork length (FL) present in nursery habitats between February and April (Parsons et al. Citation2014b). The majority of observations of these post-settlement snapper are from sheltered estuarine habitats including biogenic structure such as horse mussels and sponges (Morrison & Carbines Citation2006; Usmar Citation2009), with especially high densities associated with shallow seagrass beds (Francis et al. Citation2005; Sim-Smith et al. Citation2012; Parsons et al. Citation2013; Morrison et al. Citation2014). Larger coastal juveniles (60–230 mm FL) are of less interest as a nursery candidate as they co-occur with adults across a broader range of estuarine and more exposed coastal habitats including rocky reefs (Kingett & Choat Citation1981; Ross et al. Citation2007), bare soft sediment muds and sands (Francis Citation1995), as well as soft sediment features such as small-scale depressions, sand waves and biogenic structures (Thrush et al. Citation2002).
Second, suitable comparisons of multiple habitats are required. This can be difficult to achieve as the chosen sampling method must be capable of providing comparable estimates from a variety of habitat types and depths (Beck et al. Citation2001; Heck et al. Citation2003), but see Becker et al. (Citation2010, Citation2011) for examples of methods that have allowed across-habitat comparisons. For snapper, opportunistic use of a range of methods including fish traps (Thrush et al. Citation2002), diver transects (Kingett & Choat Citation1981; Ross et al. Citation2007), towed camera (Morrison & Carbines Citation2006), beam trawl (Morrison & Carbines Citation2006; Usmar Citation2009), otter trawl (Francis Citation1995) and beach seine (Morrison et al. Citation2014) has not been able to provide the across-habitat comparison required to assess the relative importance of different habitat types.
Here, recent advances in cost-effective camera technology allowed a comparison of the relative abundance of post-settlement snapper across habitats within an estuarine system. Four main questions were addressed:
Are higher abundances of post-settlement snapper associated with structured habitat types?
Do shallow seagrass beds have higher abundances of post-settlement snapper compared with other structured habitat types?
Do other fish species and snapper size classes have similar responses to habitat type as those of post-settlement snapper?
Is the distribution of post-settlement snapper influenced by environmental variables such as water depth and tidal water velocity?
Methods
Sampling was conducted within Whangarei Harbour, northeastern New Zealand (). Whangarei Harbour is a drowned river valley of more than 100 km2, although only about half of this area is flooded at low tide (Hume et al. Citation2007). While extensive seagrass beds were formerly present within the harbour, these largely disappeared during the last half of the 20th century (Reed et al. Citation2004). Recently, extensive seagrass recovery (c. 5 km extent) has taken place along the southern shoreline of the middle harbour (D. Parsons, pers. obs.).
Figure 1. Sites within Whangarei Harbour where camera deployments were made (stars) to estimate abundance of post-settlement snapper and other fish. Habitat type labelled at each site. North Island, New Zealand and Whangarei Harbour inset.
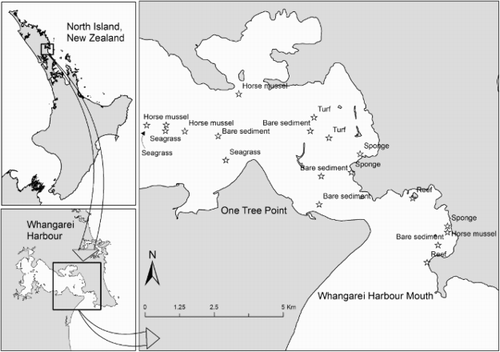
In November 2014, a habitat survey was conducted of the areas within Whangarei Harbour where post-settlement snapper are known to occur (Parsons et al. Citation2015). A combination of sonar imaging (StructureScan HD) and a surface-deployed drop camera to provide real-time video footage to a surface monitor was used. This allowed identification of areas of structure compared with bare sediment, and categorisation of the type of habitat structure where it occurred. Although this was not a comprehensive mapping exercise, this survey provided confidence that the fish sampling (below) encompassed the range of habitat types that post-settlement snapper may utilise within the harbour.
In March 2015, the time of year when post-settlement snapper abundance is at its peak (Parsons et al. Citation2014b), a fish survey was conducted across the locations assessed above. Sites were selected throughout the middle and lower harbour, ensuring that all structured habitat types, as well as bare sediment control sites, received some sampling effort. At each site, three GoPro Hero 3 cameras on 15 cm high steel stands were deployed. At sites where our previous survey had identified structured habitat, divers would search until habitat structure was found and then position a camera next to an individual structural element. Care was taken to ensure the structural element and seabed were in the field of view and the camera was placed 1 m away from the structure. This process was repeated for the other two cameras, ensuring that each camera was separated by at least 20 m. At reef and seagrass sites, where habitat elements are more continuous, divers would position cameras 1 m from the edge of the reef or seagrass patch. At previously surveyed bare sediment sites, divers confirmed the lack of structure before cameras were placed facing a patch of bare sediment. At all sites, recording cameras were left undisturbed for an hour before being retrieved via a float and thin surface line attached to the metal stand.
The first aspect of video analysis was to categorise the habitat type where the camera was deployed. Next, fish abundance was analysed using a subsampling procedure similar to that of Schobernd et al. (Citation2014). Here, 10 random times were selected within the hour of video footage obtained from each camera deployment (excluding the first 10 min to remove the potential of diver disturbance). At each of these times, 2 min of video footage was watched (ensuring that the random times selected were ≥2 min apart), counting the maximum number of fish observed at one time (within a number of species and age classes described below). Only fish that were around the habitat element or habitat edge, or closer to the camera were counted. For bare sediment sites, a similar depth of field was estimated from footage taken while the diver set the camera in place. It is possible, however, that for these bare sites fish were counted from a larger volume, which would make fish abundance estimates from sites with habitat structure conservative.
In terms of the species and size categories that abundance estimates were categorised for, the primary focus was for 0+ snapper, which should be near the end of their post-settlement stage when surveyed during March. At this time of year there should be a c. 6 cm or c. 100% difference in the length of post-settlement and 1+ snapper (Francis Citation1994), providing adequate resolution to categorise snapper into post-settlement and ≥1+ age classes. A similar methodology was followed for other common estuarine species.
Statistical analysis
The three response variables of interest for analysis were the abundance of post-settlement snapper, the number of fish species age classes observed, and the proportion of individual fish in the 0+ year class. For each video camera deployment, 10 × 2 min segments of video were viewed. The observations were averaged, resulting in one observation for each response variable for each camera deployment. While three camera deployments were made at each site, at five of 19 sites a camera either fell over or became obscured, resulting in two replicates at that site. After initial attempts to analyse these data with parametric statistical techniques (either log transforming data or directly fitting to a Poisson distribution), residuals from these models contained questionable distributions. As a result, non-parametric ANCOVA (within the PERMANOVA + routine, Primer v6) was used to analyse a resemblance matrix of Euclidian distances calculated from each response variable. To account for the likelihood that replicates within a site would be more similar than between sites, site was treated as a random variable, while habitat type was treated as a fixed variable. Continuous covariate environmental variables from each site were also offered to the model, including water depth (m below chart datum at each site as indicated on the local hydrographic chart) and horizontal water velocity (ms−1, estimated from a hydrodynamic model of Whangarei Harbour [Lundquist & Broekhuizen Citation2012]). Non-significant terms were iteratively pooled, and each model was run with 9999 permutations.
Results
The habitat survey revealed a number of different subtidal habitat types within Whangarei Harbour. These included: bare sediments (mud, sand and compacted shells); turfing algae (expanses of sediment and compacted shells with a layer of turfing red and brown algae of <5 cm height, see Neill et al. [Citation2012] for a list of species occurring within Whangarei Harbour); reef (structurally complex rocky reefs dominated by large, potentially well over 1 m in height, brown algae e.g. Ecklonia and Carpophyllum sp.); horse mussels (sediment containing patches of horse mussels [Atrina zealandica], up to 10 per m2 and of c. 5–15 cm in height); sponges (sediment interspersed with sponges [e.g. Dactylia varia, and Chondropsis kirkii], ascidians and rhodolith algae of c. 20 cm in height); and seagrass (a large expanse [>5 km] of seagrass [Zostera muelleri], with blade lengths c. 20 cm in height, located around One Tree Point).
During the fish survey, 52 camera replicates were deployed across 19 sites (two turfing algae, two reef, three sponge, three seagrass, four horse mussel and five bare sediment) within Whangarei Harbour. Using these camera deployments, it was possible to observe fish across all habitats. Fish communities were dominated by juvenile, sub-adults and adults from six species including: snapper, spotty (Notolabrus celidotus), trevally (Pseudocaranx dentex), goatfish (Upeneichthys lineatus), leatherjacket (Parika scaber) and parore (Girella tricuspidata). Small benthic species that also utilise estuarine habitats as adults (triplefins Forsterygion sp. and Grahamina sp. and goby Favonigobius exquisitus) as well as non-site attached pelagic fish (piper Hyporhamphus ihi) were not counted as they would not provide insight about the relative importance of juvenile fish habitat.
Post-settlement snapper were one of the most abundant fish species size classes observed, occurring at the most camera replicates (29 of 52) and having the second-highest overall abundance (an average of 0.75 post-settlement snapper per 2 min observation across all sites) behind 0+ spotty. Post-settlement snapper were not observed at reef or turfing algae sites, and were rare at bare sediment sites (A) (only observed at three of the 14 camera replicates at bare sediment sites). Alternatively, post-settlement snapper were frequently observed at horse mussel, sponge and seagrass sites (B), present at 26 of the 28 camera replicates at the sites represented by these habitat types. The final permutation based ANCOVA model of post-settlement snapper abundance retained a pooled variable containing the random factor site (d.f. = 13, pseudo–F = 3.15, P = 0.0038) and a separate variable for the fixed factor habitat (d.f. = 5, pseudo–F = 5.54, P = 0.0064). Pairwise comparisons of the different levels of the habitat variable (see for P values) suggested that the strongest differences were driven by a higher abundance of post-settlement snapper at sponge sites compared with bare sediment, reef and turfing algae, and also a higher abundance at seagrass sites compared with bare sediment. In addition, there was some indication that seagrass sites may have a higher abundance of post-settlement snapper compared with reef and turfing algae and that horse mussel sites may have a higher abundance than bare sediment. There was no evidence of any difference in abundance between the three habitat types with the highest post-settlement snapper abundance (sponge, seagrass and horse mussel).
Figure 2. Response of post-settlement snapper and other fish by habitat type within Whangarei Harbour. A, The average number of post-settlement snapper; B, the average number of fish species age classes (0+ and ≥1+ for each species); C, the proportion of individual fish that were in the 0+ age class. Response variables are calculated from 2 min video observations and represent the overall habitat average of site averages. Error bars are ±1 SEM. Statistical comparisons between habitat types are expressed in , and 3.
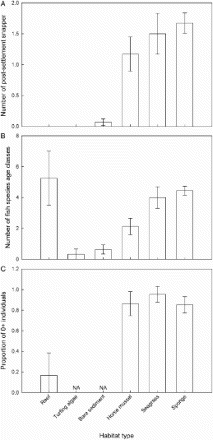
Table 1. Pairwise comparisons for the abundance of post-settlement snapper by habitat type.
The number of fish species age classes (hereafter termed diversity) observed across habitat types followed a similar trend to post-settlement snapper, with low diversity at turfing algae and bare sediment sites, and higher diversity at horse mussel, seagrass and sponge sites (B). The main exception was at reef habitat sites. While no post-settlement snapper were observed at reef sites, they had the highest overall fish diversity (B). The final permutation-based ANCOVA model of diversity retained a pooled variable containing the random factor site (d.f. = 13, pseudo–F = 3.17, P = 0.0048) and a separate variable for the fixed factor habitat (d.f.= 5, pseudo–F = 12.043, P = 0.0005). Pairwise comparisons of the different levels of the habitat variable (see for P values) suggested that the strongest differences were driven by higher diversity at sponge and reef sites compared with bare sediment and turfing algae, as well as higher diversity at seagrass sites compared with bare sediment. Horse mussel sites had an intermediate level of fish diversity, with some indication of higher diversity compared with bare sediment and turfing algae sites, and some indication of lower diversity compared with sponge, reef and seagrass sites.
Table 2. Pairwise comparisons for the number of fish species age classes (0+ and ≥1+ for each species) by habitat type.
The proportion of 0+ aged individual fish was generally higher for horse mussel, seagrass and sponge habitats than it was for reef habitat (C; comparisons for bare sediment and turfing algae were disregarded because no fish were observed for most of these comparisons). The final permutation-based ANCOVA model of 0+ proportion retained a pooled variable containing the random factor site (d.f. = 13, pseudo–F = 2.370, P = 0.054) and a separate variable for the fixed factor habitat (d.f. = 5, pseudo–F = 10.271, P = 0.0012). Pairwise comparisons of the different levels of the habitat variable (see for P values) suggested that a lower proportion of 0+ individuals occurred at reef sites compared with all other habitat types, with some evidence that sponge sites had a lower 0+ proportion than seagrass habitat.
Table 3. Pairwise comparisons for the proportion of 0+ individual fish by habitat type.
Discussion
Video surveys conducted throughout Whangarei Harbour demonstrated a clear response of post-settlement snapper abundance to structured habitat types. This result was not surprising, as post-settlement snapper have previously been shown to respond positively to the amount of available structure (density of artificial strucure elements, Parsons et al. Citation2013) and similar responses have been documented for other fish species (Nagelkerken et al. Citation2000). Further to this abundance response, however, post-settlement snapper were equally abundant across various forms of structured habitat including sponge, seagrass and horse mussels. This also agrees with previous findings which suggest that juvenile fish respond to structure per se (Heck et al. Citation2003), rather than a particular aspect of seagrass itself. This would suggest that while seagrass plays a valuable role in the life history requirements of some New Zealand fishes (Morrison et al. Citation2014), alternative habitats are also capable of fulfilling this function. It is important to note, however, that only one aspect of nursery value (abundance) was assessed. It is possible that differences in other aspects of nursery value (e.g. growth and survival, Beck et al. Citation2001) could occur between the structured habitats that post-settlement snapper used here. For example, in a similar study, Becker et al. (Citation2010) observed that zooplanktivorous fish only fed when among reed habitat, which might suggest that this habitat has a higher nursery value regardless of fish abundance. For snapper, larvae are known to respond to seagrass odour (Radford et al. Citation2012), which could suggest that specific aspects of seagrass can be important to snapper.
The response of post-settlement snapper to structure was not universal, however, with no snapper of this age class being observed in association with the most structurally complex habitat type, reef (although spatial interspersion of reef sites was limited, see below). Other fish species and older snapper did utilise the reef habitat, with overall demography dominated by sub-adult and adult individuals, as opposed to the predominance of 0+ individuals among seagrass, sponge and, to some extent, horse mussel habitats. A bias towards juvenile fish in non-reef habitats and adults on reefs is a reasonably common phenomenon (Nagelkerken et al. Citation2000; Grol et al. Citation2011; Kimirei et al. Citation2015). Habitat-related age distributions such as this are compatible with both habitat-related ontogeny (Dahlgren & Eggleston Citation2000; McDermott & Shima Citation2006) and the nursery hypothesis in general (Beck et al. Citation2001). The mechanisms explaining habitat-related age distributions were not examined here, but it has been investigated for other fish species. One possibility is competition for shelter with older congeners on reef habitat, which can lead to increased predator vulnerability (Bailey et al. Citation2001; Mudrak & Szedlmayer Citation2012). In general, predatory fish abundance and mortality of juvenile fish are also higher among reef habitat (Dorenbosch et al. Citation2009; Grol et al. Citation2011), which may also explain the predominance of juveniles among non-reef habitats.
Previous research has suggested that rocky reef habitats are of importance to juvenile snapper (Kingett & Choat Citation1981; Ross et al. Citation2007). These surveys focused on snapper of <130 and <100 mm FL, respectively (Kingett & Choat Citation1981; Ross et al. Citation2007), corresponding to either 1+ snapper, or 0+ snapper at the end of their first year, depending on the timing of the surveys conducted (Francis Citation1994). The results of these studies are therefore compatible with those found here (we also observed snapper of these sizes among rocky reef habitat) and as such do not provide insight about habitat utilisation of post-settlement snapper (<60 mm FL and present between February and April). These distinctions emphasise the importance of incorporating life cycle ontogeny when assessing nursery value (Beck et al. Citation2001; Parsons et al. Citation2014b).
One of the main limitations of the present study was the incomplete spatial interspersion of habitat types, and as a result our sampling sites, throughout Whangarei Harbour. As such, some of the habitat related responses of post-settlement snapper could alternatively be explained by environmental variables. For example, in a previous study, water velocity was identified as important to post-settlement snapper (Parsons et al. Citation2015), but a similar relationship was not observed here for either water velocity or depth. This lack of response may have been due to the minimal capacity to assess the effect of environmental variables (both by themselves and their interaction with habitat type) within our sampling design. The positive response of post-settlement snapper abundance to structure in general, however, remains more certain because bare sediment and structured sites were well interspersed; this effect has been demonstrated previously for post-settlement snapper (Parsons et al. Citation2013). Regarding the influence of water depth, others have suggested that juvenile fish will be most abundant in shallow water habitats, such as seagrass, where predators are often rare (the shallow water refuge hypothesis, Ryer et al. Citation2010). While we may have reduced capacity for inference, we can say that post-settlement snapper were not restricted to just shallow seagrass sites, with reasonable abundances observed down to 6.5 m below chart datum.
Overall, the results show that structured, but non-reef, estuarine habitats are likely to provide nursery value for juvenile fishes such as post-settlement snapper. For coastal managers, this suggests that any efforts to promote conditions that maintain biogenic habitats—such as changes to land-use practice that lead to improved water quality (Morrison et al. Citation2009)—have the capacity to benefit fish populations. Furthermore, attempts to restore biogenic habitats (McLeod et al. Citation2014; Gillies et al. Citation2015) may also be relevant, although the benefits of additional habitat to fishery recruitment would need to be established before justifying such efforts (Blandon & Ermgassen Citation2014; Parsons et al. Citation2014a). Specifically regarding seagrass, although it probably serves as an important nursery habitat in some regions, in others it is extremely rare and likely to be of little functional value to post-settlement snapper (e.g. the Hauraki Gulf, Morrison et al. Citation2014). Our results suggest that alternative biogenic habitat types might fulfil a nursery role for post-settlement snapper where seagrass is absent. Not all estuarine systems, however, may operate in the same way as our survey location, Whangarei Harbour. For example, in another northeastern New Zealand harbour, much higher abundances of post-settlement snapper have been observed associated with seagrass (c. 60 individuals within similar 2 min video observations, M. Lowe and M. Morrison, NIWA, unpubl. data). Beyond this, the value of more exposed coastal systems to post-settlement snapper remains poorly understood. Accounting for spatial dynamics such as these, and ecosystem complexity in general, would be a prudent step towards incorporating an appreciation of nursery habitat value (Sheaves et al. Citation2015) into a broader ecosystem-based management strategy (Rice Citation2011).
Acknowledgements
Thanks to Juliane Chetham of Patuharakeke and Ricky Eyre of Northland Regional Council for ongoing support of this project and Meredith Lowe, Ian Tuck, Mark Morrison, Bruce Hartill and Drew Lohrer (NIWA) for general project advice as well as Michelle Kelly and Roberta D'Archino (NIWA) for assistance with sponge and algae habitat descriptions.
Associate Editor: Professor Kendall Clements.
Disclosure statement
No potential conflict of interest was reported by the authors.
Additional information
Funding
References
- Bailey HKIV, Cowan JH Jr, Shipp RL. 2001. Experimental evaluation of potential effects of habitat size and presence of conspecifics on habitat association by young-of-the-year red snapper. Gulf Mexico Sci. 19:119–131.
- Beck MW, Heck KL, Able KW, Childers D, Eggleston D, Gillanders BM, Halpern B, Hays C, Hoshino K, Minello T, et al. 2001. The identification, conservation, and management of estuarine and marine nurseries for fish and invertebrates. BioSci. 51:633–641. doi: 10.1641/0006-3568(2001)051[0633:TICAMO]2.0.CO;2
- Becker A, Cowley PD, Whitfield AK. 2010. Use of remote underwater video to record littoral habitat use by fish within a temporarily closed South African estuary. J Exper Mar Biol Ecol. 391:161–168. doi: 10.1016/j.jembe.2010.06.028
- Becker A, Whitfield AK, Cowley PD, Jarnegren J, Naesje TF. 2011. An assessment of the size structure, distribution and behaviour of fish populations within a temporarily closed estuary using dual frequency identification sonar (DIDSON). J Fish Biol. 79:761–775. doi: 10.1111/j.1095-8649.2011.03057.x
- Blandon A, Ermgassen PSEZ. 2014. Quantitative estimate of commercial fish enhancement by seagrass habitat in southern Australia. Estuar Coast Shelf Sci. 141:1–8. doi: 10.1016/j.ecss.2014.01.009
- Butler AJ, Jernakoff P. 2000. Seagrass in Australia: strategic review and development of an R & D plan. Collingwood, Victoria: CSIRO Publishing.
- Dahlgren CP, Eggleston DB. 2000. Ecological processes underlying ontogenetic habitat shifts in a coral reef fish. Ecology. 81:2227–2240. doi: 10.1890/0012-9658(2000)081[2227:EPUOHS]2.0.CO;2
- Dorenbosch M, Grol MGG, de Groene A, van der Velde G, Nagelkerken I. 2009. Piscivore assemblages and predation pressure affect relative safety of some back-reef habitats for juvenile fish in a Caribbean bay. Mar Ecol Prog Ser. 379:181–196. doi: 10.3354/meps07896
- Dorenbosch M, van Riel MC, Nagelkerken I, van der Velde G. 2004. The relationship of reef fish densities to the proximity of mangrove and seagrass nurseries. Esuar Coast Shelf Sci. 60:37–48. doi: 10.1016/j.ecss.2003.11.018
- Francis MP. 1994. Growth of juvenile snapper, Pagrus auratus. New Zeal J Mar Freshw Res. 28:201–218. doi: 10.1080/00288330.1994.9516608
- Francis MP. 1995. Spatial and seasonal variation in the abundance of juvenile snapper (Pagrus auratus) in the north-western Hauraki Gulf. New Zeal J Mar Freshw Res. 29:565–579. doi: 10.1080/00288330.1995.9516688
- Francis MP, Morrison MA, Leathwick J, Walsh C. 2011. Predicting patterns of richness, occurrence and abundance of small fish in New Zealand estuaries. Mar Freshw Res. 62:1327–1341. doi: 10.1071/MF11067
- Francis MP, Morrison MA, Leathwick J, Walsh C, Middleton C. 2005. Predictive models of small fish presence and abundance in northern New Zealand harbours. Estuar Coast Shelf Sci. 64:419–435. doi: 10.1016/j.ecss.2005.03.007
- Gillies CL, Fitzsimons JA, Branigan S, Hale L, Hancock B, Creighton C, Alleway H, Bishop MJ, Brown S, Chamberlain D, et al. 2015. Scaling-up marine restoration efforts in Australia. Ecol Manage Restor. 16:84–85. doi: 10.1111/emr.12159
- Grol MGG, Nagelkerken I, Rypel AL, Layman CA. 2011. Simple ecological trade-offs give rise to emergent cross-ecosystem distributions of a coral reef fish. Oecologia. 165:79–88. doi: 10.1007/s00442-010-1833-8
- Heck KL Jr, Hays G, Orth RJ. 2003. Critical evaluation of the nursery role hypothesis for seagrass meadows. Mar Ecol Prog Ser. 253:123–136. doi: 10.3354/meps253123
- Hume TM, Snelder T, Weatherland M, Liefting R. 2007. A controlling factor approach to estuary classification. Ocean Coast Manag. 50:905–929. doi: 10.1016/j.ocecoaman.2007.05.009
- Kimirei IA, Nagelkerken I, Slooter N, Gonzalez ET, Huijbers CM, Mgaya YD, Rypel AL. 2015. Demography of fish populations reveals new challenges in appraising juvenile habitat values. Mar Ecol Prog Ser. 518:225–237. doi: 10.3354/meps11059
- Kingett PD, Choat JH. 1981. Analysis of density and distribution patterns in Chrysophrys auratus (Pisces: Sparidae) within a reef environment: an experimental approach. Mar Ecol Prog Ser. 5:283–290. doi: 10.3354/meps005283
- Lundquist CJ, Broekhuizen N. 2012. Predicting suitable shelfish restoration sites in Whangarei Harbour. Larval dispersal modelling and verification. Report prepared for Ministry of Science Innovation Envirolink Fund to Northland Regional Council. [cited 2014 Sept 26]. Available from: http://www.envirolink.govt.nz/PageFiles/806/1024-NLRC134%20LTRACK-Predicting%20suitable%20shellfish%20restoration%20sites%20in%20Whangarei%20harbour.pdf
- McDermott CJ, Shima JS. 2006. Ontogenetic shifts in microhabitat preference of the temperate reef fish Forsterygion lapillum: implications for population limitation. Mar Ecol Prog Ser. 320:259–266. doi: 10.3354/meps320259
- McLeod IM, Parsons DM, Morrison MA, Van Dijken SG, Taylor RB. 2014. Mussel reefs on soft sediments: a severely reduced but important habitat for macroinvertebrates and fishes in New Zealand. New Zeal J Mar Freshw Res. 48:48–59. doi: 10.1080/00288330.2013.834831
- Morrison M, Carbines G. 2006. Estimating the abundance and size structure of an estuarine population of the sparid Pagrus auratus, using a towed camera during nocturnal periods of inactivity, and comparisons with conventional sampling techniques. Fish Res (Amsterdam). 82:150–161. doi: 10.1016/j.fishres.2006.06.024
- Morrison MA, Lowe M, Parsons DM, Usmar NR, McLeod IM. 2009. A review of land-based effects on coastal fisheries and supporting biodiversity in New Zealand. New Zealand Aquatic Environment and Biodiversity Report No. 37. Wellington: Ministry of Fisheries; [cited 2015 Nov 20]. Available from: http://www.fish.govt.nz/NR/rdonlyres/0BA191BC-FF2D-41A1-AC6A-5DD76C01FF75/0/MorrisonAEBR37_FINALHR.pdf
- Morrison MA, Lowe ML, Grant C, Smith PJ, Carbines G, Reed J, Bury SJ, Brown J. 2014. Seagrass meadows as biodiversity and productivity hotspots. New Zealand Aquatic Environment and Biodiversity Report No. 137. Wellington: Ministry for Primary Industries; [cited 2015 Sept 30]. Available from: https://www.mpi.govt.nz/document-vault/4409
- Mudrak PA, Szedlmayer ST. 2012. Proximity effects of larger resident fishes on recruitment of age-0 red snapper in the northern Gulf of Mexico. Transa Am Fish Soc. 141:487–494. doi: 10.1080/00028487.2012.667045
- Nagelkerken I, Dorenbosch M, Verberk W, de la Moriniere EC, van der Velde G. 2000. Importance of shallow-water biotopes of a Caribbean bay for juvenile coral reef fishes: patterns in biotope association, community structure and spatial distribution. Mar Ecol Prog Ser. 202:175–192. doi: 10.3354/meps202175
- Nagelkerken I, Roberts CM, van der Velde G, Dorenbosch M, van Riel MC, Cocheret de la Morinière E, Nienhuis PH. 2002. How important are mangroves and seagrass beds for coral-reef fish? The nursery hypothesis tested on an island scale. Mar Ecol Prog Ser. 244:299–305. doi: 10.3354/meps244299
- Neill K, D'Archino R, Farr T, Nelson W. 2012. Macroalgal diversity associated with soft sediment habitats in New Zealand. New Zealand Aquatic Environment and Biodiversity Report No. 87. Ministry for Primary Industries; [cited 2015 Oct 14]. Available from: http://fs.fish.govt.nz/Doc/22978/AEBR_87.pdf.ashx
- Parsons D, Morrison M, Thrush SF, Middleton C, Smith M, Spong KT, Buckthought D. 2013. The influence of habitat structure on juvenile fish in a New Zealand estuary. Mar Ecol. 34:492–500. doi: 10.1111/maec.12050
- Parsons DM, Middleton C, Smith MD, Cole RG. 2014a. The influence of habitat availability on juvenile fish abundance in a northeastern New Zealand estuary. New Zeal J Mar Freshw Res. 48:216–228. doi: 10.1080/00288330.2013.875927
- Parsons DM, Middleton C, Spong KT, Mackay G, Smith MD, Buckthought D. 2015. Mechanisms explaining nursery habitat associationL how do juvenile snapper (Chrysophrys auratus) benefit from their nursery habitat? PlosOne. 10(3):e0122137. doi:10.1371/journal.pone.0122137.
- Parsons DM, Sim-Smith CJ, Cryer M, Francis MP, Hartill B, Jones EG, Le Port A, Lowe M, McKenzie J, Morrison M, et al. 2014b. Snapper (Chrysophrys auratus): a review of life history and key vulnerabilities in New Zealand. New Zeal J Mar Freshw Res. 48:256–283. doi: 10.1080/00288330.2014.892013
- Radford CA, Sim-Smith CJ, Jeffs AG. 2012. Can larval snapper, Chrysophrys auratus, smell their new home? Mar Freshw Res. 63:898–904. doi: 10.1071/MF12118
- Reed J, Schwarz A, Gosai A, Morrison M. 2004. Feasibility study to investigate the replenishment/reinstatement of seagrass beds in Whangarei Harbour—Phase 1. NIWA Client Report to Northland Regional Council; [cited 2014 September 26]. Available from: http://www.nrc.govt.nz/Resource-Library-Summary/Research-and-reports/Coastal/Seagrass-in-Whangarei-Harbour/
- Rice J. 2011. Managing fisheries well: delivering the promises of an ecosystem approach. Fish Fish. 12:209–231. doi: 10.1111/j.1467-2979.2011.00416.x
- Ross PM, Thrush SF, Montgomery JC, Walker JW, Parsons DM. 2007. Habitat complexity and predation risk determine juvenile snapper (Pagrus auratus) and goatfish (Upeneichthys lineatus) behaviour and distribution. Mar Freshw Res. 58:1144–1151. doi: 10.1071/MF07017
- Ryer CH, Laurel BJ, Stoner AW. 2010. Testing the shallow water refuge hypothesis in flatfish nurseries. Mar Ecol Prog Ser. 415:275–282. doi: 10.3354/meps08732
- Schobernd ZH, Bacheler NM, Conn PB. 2014. Examining the utility of alternative video monitoring metrics for indexing reef fish abundance. Can J Fish Aquat Sci. 71:464–471. doi: 10.1139/cjfas-2013-0086
- Sheaves M, Baker R, Nagelkerken I, Connolly RM. 2015. True Value of Estuarine and Coastal Nurseries for Fish: Incorporating Complexity and Dynamics. Estuar Coast. 38:401–414. doi: 10.1007/s12237-014-9846-x
- Sim-Smith CJ, Jeffs AG, Radford CA. 2012. Variation in the growth of larval and early juvenile snapper, Chrysophrys auratus (Sparidae). Mar Freshw Res. 63:1231–1243. doi: 10.1071/MF12176
- Thrush SF, Schultz D, Hewitt JE, Talley D. 2002. Habitat structure in soft-sediment environments and abundance of juvenile snapper Pagrus auratus. Mar Ecol Prog Ser. 245:273–280. doi: 10.3354/meps245273
- Usmar NR. 2009. Ontogeny and ecology of snapper (Pagrus auratus) in an estuary, the Mahurangi Harbour [Unpublished PhD thesis]. Auckland: The University of Auckland.
- Warren MA, Gregory RS, Laurel BJ, Snelgrove PVR. 2010. Increasing density of juvenile Atlantic (Gadus morhua) and Greenland cod (G. ognac) in association with spatial expansion and recovery of eelgrass (Zostera marina) in a coastal nursery habitat. J Exper Mar Biol Ecol. 394:154–160. doi: 10.1016/j.jembe.2010.08.011
- Whitfield AK, Pattrick P. 2015. Habitat type and nursery function for coastal marine fish species, with emphasis on the Eastern Cape region, South Africa. Estuar Coast Shelf Sci. 160:49–59. doi: 10.1016/j.ecss.2015.04.002