ABSTRACT
The dinoflagellate genus Ostreopsis produces palytoxin-like compounds (PLTXs) and the genus Gambierdiscus produces ciguatoxins (CTXs) and maitotoxin (MTX). Human illness has been recorded following the consumption of CTX-contaminated finfish. Their effects on the flora and fauna in the environment are largely unexplored. Toxin profiles of extracts of Ostreopsis and Gambierdiscus isolates from the Pacific region were characterised using liquid chromatography tandem mass spectrometry. Sea urchin (Evechinus chloriticus) fertilisation was unaffected by any algal extracts, but extracts containing maitotoxin-3 (MTX-3, but not MTX or CTXs), or with high concentrations of PLTXs, were toxic to urchin larval development. Gambierdiscus extracts containing CTXs elicited toxic responses in zebrafish (Danio rerio) embryo toxicity assays; extracts containing only MTX-3 elicited a limited response. Ostreopsis extracts containing PLTXs caused no response. The results demonstrate potential impacts of toxic dinoflagellates on larval organisms and the potential of embryonic assays as toxicity screening tools.
Introduction
Blooms of toxin-producing dinoflagellates of the genera Ostreopsis Schmidt and Gambierdiscus Adachi and Fukuyo pose a threat to human and environmental health across a wide and expanding geographic range (Berdalet et al. Citation2012). Ostreopsis siamensis Schmidt blooms have been observed in northern New Zealand, causing mass sea urchin (Evechinus chloroticus) mortalities (Shears & Ross Citation2009), a phenomenon also observed elsewhere (Illoul et al. Citation2012; Privitera et al. Citation2012). Some Ostreopsis spp. produce palytoxin and a variety of structurally related analogues such as ovatoxins, ostreocins and mascarenotoxins (collectively referred to here as PLTXs) (Ciminiello et al. Citation2011). These have been reported to cause human illness through consumption of contaminated seafood or inhalation of toxin bearing aerosols (Tubaro et al. Citation2011). Gambierdiscus blooms have been linked to outbreaks of ciguatera poisoning through consumption of fish that have accumulated ciguatoxins (CTXs) produced by the algae (Fleming et al. Citation2011). Non-CTX producing Fukuyoa paulensis Gomez, Qiu, Lopes and Lin (previously known as Gambierdiscus cf. yasumotoi Holmes and referred to as such here) has recently been found in northern New Zealand (Rhodes et al. Citation2014a) with a perceived threat of range expansion with global climate change. Some Gambierdiscus species also produce maitotoxin (MTX) and all Gambierdiscus tested so far produce maitotoxin-3 (MTX-3), a putative analogue previously described (Holmes & Lewis Citation1994). Maitotoxins are among the most toxic known natural substances but their connection to ciguatera or human illness is not yet fully understood.
Intra- and inter-specific variation in toxicity of Ostreopsis and Gambierdiscus has been well documented (Parsons et al. Citation2012). Therefore, toxicity studies must be strain-specific. New isolates are frequently being collected and described from locations worldwide including the Cook Islands, Korea and New Zealand (Rhodes et al. Citation2010, Citation2014b; Kang et al. Citation2013). Live cultures from the Pacific region and New Zealand are maintained at the Cawthron Institute Culture Collection of Micro-algae (http://cultures.cawthron.org.nz/) ().
The negative human health impacts of Ostreopsis and Gambierdiscus are increasingly documented, but the effects of toxin-producing micro-algae on flora and fauna in situ is not well understood. However, studies have indicated that toxins from these algae represent a risk to some species (Edmunds et al. Citation1999; Pagliara & Caroppo Citation2012). Early life stages may be more at risk as they often lack the detoxifying mechanisms of adults (Vasconcelos et al. Citation2010).
Sea urchins (invertebrate) and zebrafish (vertebrate) are common, internationally accepted model organisms used in toxicity testing. The fish embryo toxicity (FET) test using zebrafish embryos is a standardised OECD protocol (OECD Citation2013) and sea urchin developmental processes (fertilisation and larval development) have been widely used for toxicity testing, mainly for environmental quality assessment of sediments (Nipper et al. Citation1997; Fernández & Beiras Citation2001; Environment Canada Citation2011).
The aim of this study was to assess the toxicity of extracts of toxin and non-toxin producing Ostreopsis and Gambierdiscus to sea urchin and zebrafish embryos. The toxin production of each strain was characterised using liquid chromatography paired with tandem mass spectrometry (LC-MS/MS) methods. This combination of methodologies allows the comparison of toxicity data with toxin profiles of each micro-alga.
Materials and methods
Algae culture and extraction
Seven Ostreopsis and five Gambierdiscus isolates ( and ) were grown solely in f2 medium (Guillard Citation1975) and autoclaved sterile sea water (1:1 v/v) with the exception of CAWD149 that was grown purely in f2. Sequence data is available in GenBank for some strains. Temperature was set at 25 ± 2 °C and 19 ± 2 °C with 40–70 µmol m−2 s−1 and 80 µmol m−2 s−1 photon irradiance (12:12 h light:dark) for Gambierdiscus and Ostreopsis, respectively. Harvesting occurred at the stationary phase. Samples of each culture (0.5 mL) were settled for 4 h in Utermöhl counting chambers with one drop of Lugol’s iodine and manually counted to determine concentration using an Olympus CK2 microscope.
Table 1. Palytoxin equivalents (based on quantitation of the PLTX amine fragment by oxidative cleavage analysis) of Ostreopsis extracts and total number of cells extracted from each culture.
Table 2. Ciguatoxin and maitotoxin contents of Gambierdiscus culture extracts and total number of cells extracted from each culture.
Ostreopsis and Gambierdiscus cultures were centrifuged at 4424 g and 3583 g respectively for 15–25 min at 15–17 °C to form a cell pellet. The supernatant was removed and pellets stored at −18 °C until analysis. Lysates from cell pellets were extracted using 5 mL methanol until pale in colour by alternating ultrasonication (10 min at 59 kHz) and vortex mixing twice before centrifugation of 5 min at 3220 g. Supernatants were retained and pooled. An extra single extraction was carried out for all samples using methanol-water (1:1 v/v). This approach has been shown to be most effective for PLTX extraction (Selwood et al. Citation2012). Extracts were dried under N2 stream at 30 °C and re-suspended in methanol to a concentration of 10 mg mL–1, with the exception of the three Cook Islands Gambierdiscus extracts (CAWD212, GAMB10 and CAWD227) which were made up to a concentration of 5 mg mL–1. Aliquots (1 mL) of the methanol extracts were transferred into 1.5 mL glass vials and dried under N2 stream at 30 °C. Then, 0.5 mL of dimethyl sulfoxide (DMSO) was added and vials placed in an ultra-sonic bath to ensure the extracts were re-suspended for use in the bioassays.
Sample preparation of Ostreopsis extracts for LC-MS/MS analysis
All Ostreopsis extracts were prepared for analysis using a solid phase extraction (SPE) procedure that involved on-column oxidative cleavage of any palytoxin-like compounds contained within the extract. Briefly, 0.5 mL aliquots of the various Ostreopsis extracts, or palytoxin standards (5–50 ng mL–1), were added to 15 mL plastic tubes containing 0.5 mL of Milli-Q water. The whole volume was then subjected to the SPE procedure described by Selwood et al. (Citation2012). Oxidative cleavage of PLTX results in two nitrogen containing fragments, one containing an amino aldehyde and the other an amide aldehyde, both of which are readily detected via LC-MS/MS analysis. The amino fragment is common to all known palytoxin-like toxins tested to date with variations observed in the fragment containing the amide group.
LC-MS/MS analysis of algal extracts
LC-MS/MS was used to determine the presence and concentration of both the amino and amide fragments as a means to determine if PLTXs were present in a sample. A palytoxin calibration curve was prepared using the on-column oxidative cleavage procedure to calibrate the instrument response. Purified palytoxin was from Wako Pure Chemical Industries. Any extract that gave a response greater than the calibration range was diluted 10-fold and re-analysed. Representative LC-MS/MS chromatograms for PLTX standards and CAWD184 used in this study are available in Selwood et al. (Citation2012).
LC-MS/MS analysis was also performed on Gambierdiscus algal extracts to determine the presence of CTXs, MTX and MTX-3. These toxins were monitored as intact structures using methods developed in-house for ciguatoxins (Tim Harwood, Cawthron Institute, pers. comm. 2014) and as described in Selwood et al. (Citation2014) for maitotoxins, where chromatograms are also published. Peak areas in samples were compared to those of CTXs and MTX calibration standards (0–20 ng mL–1), which were spread between the algal extracts to generate a calibration curve. Purified CTXs and MTX are not available commercially and the material used for this project was kindly gifted to us from French Polynesian (CTXs) and Japanese (MTX) scientists. CAWD212 extract dilutions of 1/10, 1/100 and 1/1000 were analysed to assess the effect of algal matrix on CTX ionisation and because the undiluted extract gave a peak area well outside the calibration range. The CTX result represents the sum of the four algal-derived ciguatoxin analogues quantified (CTX-3B, CTX-3C, CTX-4A, CTX-4B). The limit of detection for the analysis of the various marine toxins (PLTXs, CTXs and MTX) was determined to be at least 1 ng mL–1.
Preparation of bioassay test solutions
The same concentrations of algal extract were used for both bioassays. For the sea urchin assay, filtered seawater was collected from Kelly Tarlton’s Sealife Aquarium (Orakei, Auckland, New Zealand) and additionally filtered through two 1 µm filter bags (hereafter called filtered seawater). For the FET assay, fish dilution water was made according to OECD TG 203 Annex 2 (OECD Citation1992). Briefly, CaCl22H2O (29.4 g L–1), MgSO4.7H2O (12.303 g L–1), NaHCO3 (6.47 g L–1) and KCl (0.57 g L–1) stock solutions (10 mL of each) were added to 960 mL of Milli-Q water and aerated until oxygen saturation.
Re-suspended DMSO extract (5 µL) was added to 50 mL of filtered seawater or fish dilution water resulting in 2 mg L–1 of algae in solution for all extracts except CAWD212, GAMB10 and CAWD227, for which it was 1 mg L–1 due to lower dry weights of the extracted cultures. After preliminary experiments, extracts were tested across a concentration range of 0.19, 0.34, 0.62, 1.1 and 2 mg L–1 or 0.095, 0.17, 0.31, 0.56 and 1 mg L–1 (for CAWD212, GAMB10 and CAWD227) to establish a dose-response relationship.
Zinc (as ZnSO4.7H2O) in seawater was used as reference toxicant for the sea urchin test at concentrations ranging from 0.0033 to 0.36 mg L–1. For the FET test reference toxicant, 3,4-dichloroaniline (3,4-DCA) was used at concentrations ranging from 0.38 to 7.2 mg L–1.
Sea urchin bioassay methods
Urchin maintenance and spawning
Sea urchins (Evechinus chloroticus Valenciennes) were collected from Matheson Bay (36°18′17.28″ S, 174°47′ 51.72″ E) north of Auckland and transported in barrels of fresh seawater with ice packs to clean recirculating tanks in the University of Auckland marine laboratory. The facility was temperature controlled at 17–19 °C with a 12:12 h light:dark cycle. Spawning was induced by injection of 3 mL of 0.55 M KCl into the peristomal cavity. Sperm was collected from the gonopore using a glass Pasteur pipette, eggs were collected by inversion of the female over a dish of seawater.
Sea urchin embryo development assay
The method was based on the Environment Canada protocol (Environment Canada Citation2011) with modifications as described by Nipper et al. (Citation1997), Carballeira et al. (Citation2012a,b) and Fernandez & Beiras (Citation2001). Briefly, a sperm dilution of 10 µL in 30 mL of filtered seawater was added to approximately 200 mL of eggs at 3000 eggs mL–1 (Franke Citation2005). This solution was gently mixed on a shaker to achieve > 95% fertilisation. After 20 min, but within 1 h of fertilisation, 100 µL of fertilised egg solution (c. 300 eggs) was added to each 20 mL glass scintillation vial (Interlab) at 10 s intervals. After all additions vials were incubated undisturbed for 96 h in the dark. The test was terminated by the addition of 20% borax buffered formalin (2 mL) in seawater to each vial. Temperature, salinity and pH remained constant at 17 ± 1 °C, 30 ± 2 and 8 ± 0.5, respectively. Larvae were observed using a Sedgewick rafter slide at 20× magnification under a compound microscope. The percentage of normal and abnormal developed larvae was counted from the first 100 observed. Embryos were assessed as abnormal based on observation of obvious abnormalities or stunted growth compared to the controls.
FET bioassay methods
Wild type zebrafish (Danio rerio Hamilton) were held in the University of Auckland School of Biological Sciences facility. The bioassay was used as described in OECD test guideline 236 (OECD Citation2013). Zebrafish embryos were observed 24, 48, 72 and 96 h post-fertilisation for four lethal end points (embryo coagulation, lack of somite formation, non-detachment of the tail and lack of heartbeat) using a Zeiss Axiovert S100 microscope at 20× magnification.
Statistical analysis
For the sea urchin data, the 95% confidence intervals for the binomial proportions were used to assess differences between treatments and controls (Newcombe Citation1998). Dose-response relationships for both assays were analysed using R software (R Core Team Citation2013) with the analysis of dose-response curve data package (Ritz & Streibig Citation2005). Data were fitted to a Weibull model and median effective/lethal concentrations (E/LC50) values calculated for treatments that demonstrated a significant dose-response relationship.
Results
Toxin analysis
Five of seven Ostreopsis extracts tested gave a positive response for the amine oxidative cleavage fragment common to many PLTX. The two negative extracts were CAWD184 from the Cook Islands and the undescribed Cook Islands isolate ().
CAWD212 from the Cook Islands was the only algal species tested shown to produce CTXs, with 795 ng CTXs mg–1 extract. There was a non-linear relationship between the level of CTXs observed in the various dilutions, suggesting probable effects of the sample matrix on the LC-MS ionisation and subsequent quantitation. To account for this, the 1/100 dilution result was used to calculate total CTX level in the extract (). CAWD149 was shown to produce 338 ng MTX mg–1 extract. This was the only algal culture found to produce this large marine toxin ().
The three other Gambierdiscus extracts did not produce CTXs or MTX. All five Gambierdiscus isolates produced MTX-3. Quantitative analysis was not possible for this toxin as no standard or molecular structure information is available. Relative levels in the various extracts were obtained by comparing the MTX-3 peak area, with the following order observed: CAWD149 > CAWD227 > CAWD210 > GAMB10 > CAWD212.
Sea urchin results
Pilot studies using the fertilisation-based assay showed no significant response to any of the algal extracts (data not shown). For the embryo development assay, control and DMSO-treated larvae showed similar percentages of normally developed larvae (P < 0.05 from binomial confidence intervals). Binomial 95% confidence intervals results indicated that GAMB10, CAWD227, CAWD215 and CAWD149 exhibited a significantly different proportion of abnormally developed larvae compared to the controls at the highest test concentrations. Examples of embryo abnormalities observed following exposures to dinoflagellate extracts have been reported in Argyle et al. (Citation2014) and are shown in and .
Figure 1. GAMB10-treated (1 mg L–1) Evechinus chloroticus embryo 96 h post-fertilisation showing lack of body structure during development.
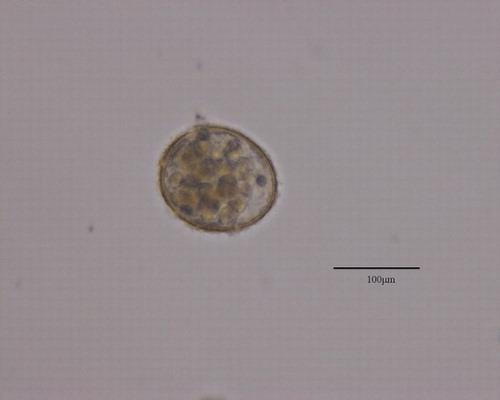
Figure 2. GAMB10-treated (0.56 mg L–1) Evechinus chloroticus embryo 96 h post-fertilisation showing abnormal development with the growth of an additional arm.
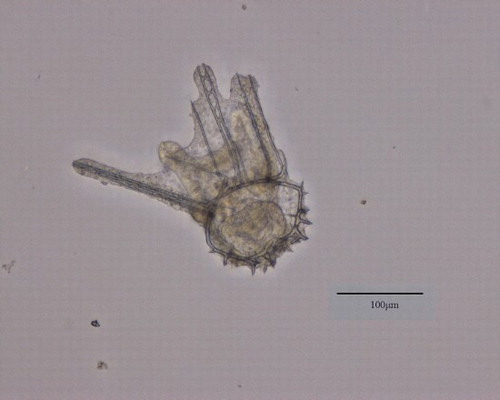
Dose-dependent responses were observed for GAMB10, CAWD227, CAWD215 and zinc using the Weibull model (). The concentration range covered with CAWD149 was insufficient to allow the calculation of a 96hr-LC50. A summary of all of the chemical analyses and bioassay results is provided in .
Table 3. Summary of toxin production of Ostreopsis and Gambierdiscus extracts and their toxicities using sea urchin development and fish embryo toxicity.
Zebrafish FET results
The zebrafish embryos 96hr-LC50 (± 95% CI) for the reference toxicant 3,4-dichloroaniline (DCA) was 3.12 (2.94–3.31) mg L–1 (). None of the Ostreopsis extracts elicited a toxic response at 2 mg L–1. All five Gambierdiscus extracts had a toxic effect at the highest test concentrations. Examples of embryo abnormalities are reported in Argyle et al. (Citation2014) and in –. At least one of the four assessed lethal endpoints was observed in 100% of embryos exposed to CAWD149, CAWD212, GAMB10 and CAWD227, and in 10% of the embryos exposed to CAWD210. When tested across a range of concentrations, the embryo toxicity of two algal extracts, CAWD149 and CAWD212, showed suitable dose-dependent responses to fit to the Weibull model (). When exposed to 1 mg L–1 of CAWD210, toxic responses were observed in 10% of the embryos. This was reduced to 5% of embryos at the lower concentrations, with the exception of 0.17 mg L–1 which had no affected embryos. GAMB10 and CAWD227 were less toxic when tested again at 1 mg L–1 concentration, with a toxic response observed in 20% and 15% of embryos for GAMB10 and CAWD227, respectively. This was reduced to 5% for GAMB10 at all low concentrations tested. CAWD227 affected 15% of embryos at a concentration of 0.56 mg L–1, but none at all lower concentrations.
Figure 3. CAWD212-treated (1 mg L–1) Danio rerio embryo 96 h post-fertilisation showing tail curvature indicating lack of somite formation.
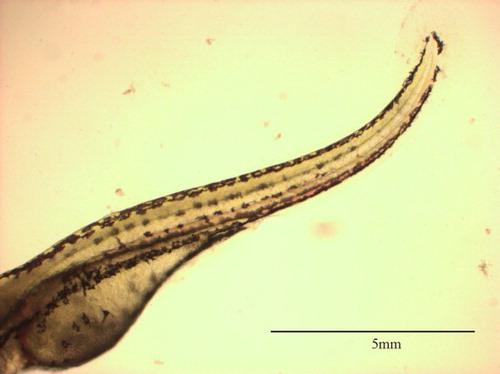
Figure 4. CAWD149-treated (2 mg L–1) Danio rerio embryo 24 h post-fertilisation showing coagulation.
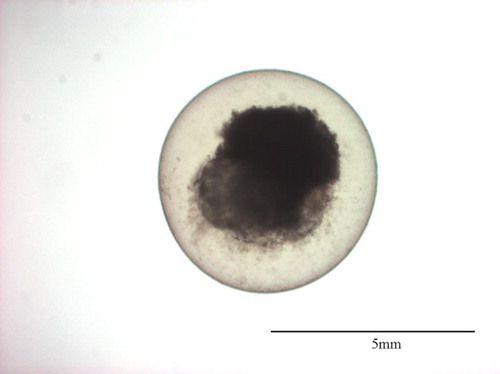
Discussion
The effects of harmful algal bloom (HAB) species on early life stages of marine and aquatic organisms have not been thoroughly investigated. The results of this research show harmful effects of benthic HAB species on the larval development of sea urchins and zebrafish, indicating possible harm to these types of organisms in situ, and bringing to light a need for further research in this area.
The sea urchin developmental assay was the only assay appraised that was sensitive to the presence of all PLTX-containing Ostreopis extracts. The extract from the Noumean Ostreopsis isolate CAWD215 caused abnormal development and also contained the highest concentration of PLTX, indicating that the other extracts tested were likely below the minimal effective concentration for this assay. Previous research has shown O. ovata cell lysate (at 3.7–7.5 mg L–1) to cause sea urchin larval fragmentation and degeneration (Pagliara & Caroppo Citation2012). Exposure to whole Ostreopsis cells has been previously shown to cause juvenile (Privitera et al. Citation2012) and adult (Shears & Ross Citation2009) sea urchin mortality in situ. Further research is needed to determine the sensitivity limit of this assay to PLTX-producing Ostreopsis species.
CTX- and MTX-containing algae extracts were not toxic to the sea urchin embryo development. Previous research has shown fertilised P. lividus eggs expressed a rise in intracellular Na+ and lack of amino acid uptake when exposed to CTX (0.5 nM) directly post-fertilisation (Allemand et al. Citation1986). The only CTX-producing isolate (CAWD212) in this study did not affect urchin fertilisation or development. However, no cellular level processes were monitored in this study so it is unknown if apparently normal larvae had reduced fitness. This is the first study reporting on the effects of maitotoxins on sea urchin development. The two G. pacificus extracts, GAMB10 and CAWD227, from the Cook Islands completely altered sea urchin development at the highest tested concentration. These extracts did not contain CTX or MTX and it is unknown whether the toxicity is due to the putative MTX-3 compound. However, as other Gambierdiscus species tested as part of this work produced this compound without being toxic, it seems unlikely. Further investigation into these strains for potentially novel toxins is warranted.
The FET test has been used in many research and regulatory settings for establishing toxicity of both individual chemicals and overall aquatic environments (Lammer et al. Citation2009). The reference toxicant EC50–96 h was comparable to the assay developer’s result of 20%–80% mortality at 3.7 mg L–1 (Lammer et al. Citation2009), providing strong indication of the validity of the assays. Exposure to toxin produced by the Ostreopsis genus, namely palytoxin, has been shown to cause mortality of embryos in an alternative fish model (medaka) with a 0.01 < LC50 < 0.1 mg L–1 for 48 h exposure (Puiseux-Dao & Edery Citation2006). Embryo mortality was not observed in this study when exposed to Ostreopsis extracts that contained PLTX compounds. This observation may be due to the low sensitivity of the FET test to the concentrations of toxin present in the extracts, which were significantly lower than in the medaka study. It could also indicate that the toxin may not penetrate the chorion.
Two previous studies have demonstrated the toxic effects of CTX on medaka larvae administered by micro-injection (Edmunds et al. Citation1999; Colman et al. Citation2004). Both studies used CTX metabolites purified from ciguatoxic fish, which have been shown to be more toxic than the original algal-derived toxins (Lewis & Holmes Citation1993). Symptoms of toxicity included reduced hatching rates, hyperactivity and spinal curvature (Edmunds et al. Citation1999). The Gambierdiscus extract containing algal CTX was highly toxic in the FET test, with spinal curvature present, as in the medaka studies. Toxicity was also observed for the MTX-containing Gambierdiscus extract, suggesting this bioassay is sensitive to both these toxin classes. MTX-3 containing extracts were also toxic to fish development, but were not as potent. It is known that with many marine biotoxins the mode of exposure greatly influences toxicity (Munday Citation2014). These results indicate that passive exposure through the chorion may affect developmental processes in finfish, which may also have ecological consequences during blooms of these benthic algae species.
Bioassays demonstrate multiple toxic responses and may assist to detect novel undescribed toxins, as is possibly the case in this study. They can be used as a first tier detection method that can be followed up by characterisation by chemical analysis. Advantages of embryonic models include their higher ethical acceptability and lower cost compared to the mouse model. The maintenance of animals is cheap and the methods are easy to conduct, not requiring particular specialist knowledge or equipment. As a standardised protocol, the FET test results may be confidently compared between laboratories (Busquet et al. Citation2014).
Our data indicates the potential for negative in situ effects of epiphytic harmful algae through toxicity to early life stages of animals, which should be considered when assessing the risk of harmful algae on ecology. Embryonic and early life stages of marine animals must be considered vulnerable to dinoflagellate blooms, which may have previously unconsidered consequences for coastal fisheries management and bloom mitigation. Further study is needed to assess the sensitivity limits of these models to Ostreopsis strains, as the sea urchin results indicated sensitivity at higher concentrations and a lack of response in the fish model gave no indication of limit. Further research is also needed to determine if these assays are suitable as algal toxin screening tools to replace the mouse bioassay. The development of screening tools for toxins from Ostreopsis and Gambierdiscus will be important in the future as the global range of these genera increases with climate changes, ballast water transfer and global movement of farmed seafood stocks.
Acknowledgements
The authors would like to thank A/Prof Mary Sewell for advice and technical support during the project. Thanks also to Bhakti Patel, Igor Ruza, Alex Leonard and Matthew Sullivan for help with urchin collection, Mike Hudson for assistance with urchin bioassay methods, and Amy Zhu for assistance with zebrafish husbandry. Thanks to Eric Goodwin for assistance with data analysis. CTX analogues were kindly provided by Dr Mireille Chinain (Institut Louis Malardé, Papeete, French Polynesia) and MTX material was from Prof Takeshi Yasumoto (Japan Food Research Laboratories, Tokyo, Japan).
Associate Editor: Dr Judy Sutherland.
Disclosure statement
No potential conflict of interest was reported by the authors.
ORCiD
O Champeau http://orcid.org/0000-0002-1892-678X
Additional information
Funding
References
- Allemand D, De Renzis G, Payan P, Girard J-P. 1986. Regulatory and energetic role of Na+ in amino acid uptake by fertilized sea urchin eggs. Dev Biol. 118:19–27. doi: 10.1016/0012-1606(86)90069-2
- Argyle P, Harwood DT, Rhodes L, Champeau O, Tremblay L. 2014. Developmental inhibition of zebrafish and sea urchin embryos by toxin producing Ostreopsis and Gambierdiscus isolates. Proceedings of the 16th International Conference on Harmful Algae; 2014 Oct 27–31; Wellington, New Zealand; p. 109–112.
- Berdalet E, Bravo I, Evans J, Fraga S, Kibler S, Kudela M, Larsen J, Litaker W, Penna A, Tester P. 2012. Global ecology and oceanography of harmful algal blooms, GEOHAB core research project: HABs in benthic systems.
- Busquet F, Strecker R, Rawlings JM, Belanger SE, Braunbeck T, Carr GJ, Cenijn P, Fochtman P, Gourmelon A, Hübler N, et al. 2014. OECD validation study to assess intra- and inter-laboratory reproducibility of the zebrafish embryo toxicity test for acute aquatic toxicity testing. Regul Toxicol Pharm. 69:496–511. doi: 10.1016/j.yrtph.2014.05.018
- Carballeira C, Orte M, Viana I, DelValls T, Carballeira A. 2012b. Assessing the toxicity of chemical compounds associated with land-based marine fish farms: the sea urchin embryo bioassay with Paracentrotus lividus and Arbacia lixula. Arch Environ Contam Toxicol. 63:249–261. doi: 10.1007/s00244-012-9769-0
- Carballeira C, Ramos-Gómez J, Martín-Díaz L, DelValls TA. 2012a. Identification of specific malformations of sea urchin larvae for toxicity assessment: application to marine pisciculture effluents. Mar Environ Res. 77:12–22. doi: 10.1016/j.marenvres.2012.01.001
- Ciminiello P, Dell’Aversano C, Iacovo ED, Fattorusso E, Forino M, Tartaglione L. 2011. LC-MS of palytoxin and its analogues: state of the art and future perspectives. Toxicon. 57:376–389. doi: 10.1016/j.toxicon.2010.11.002
- Colman JR, Dechraoui M-YB, Dickey RW, Ramsdell JS. 2004. Characterization of the developmental toxicity of Caribbean ciguatoxins in finfish embryos. Toxicon. 44:59–66. doi: 10.1016/j.toxicon.2004.04.007
- Edmunds JSG, McCarthy RA, Ramsdell JS. 1999. Ciguatoxin reduces larval survivability in finfish. Toxicon. 37:1827–1832. doi: 10.1016/S0041-0101(99)00119-1
- Environment Canada. 2011. Biological test method—fertilisation assay using echinoids (sea urchins and sand dollars). 133 p.
- Fernández N, Beiras R. 2001. Combined toxicity of dissolved mercury with copper, lead and cadmium on embryogenesis and early larval growth of the Paracentrotus lividus sea-urchin. Ecotoxicology. 10:263–271. doi: 10.1023/A:1016703116830
- Fleming LE, Kirkpatrick B, Backer LC, Walsh CJ, Nierenberg K, Clark J, Reich A, Hollenbeck J, Benson J, Cheng YS, and others. 2011. Review of Florida red tide and human health effects. Harmful Algae. 10:224–233. doi: 10.1016/j.hal.2010.08.006
- Franke ES. 2005. Aspects of fertilisation ecology in Evechinus chloroticus and Coscinasterias muricata [Unpublished thesis]. Auckland, New Zealand: University of Auckland.
- Guillard RR. 1975. Culture of phytoplankton for feeding marine invertebrates. In: Culture of marine invertebrate animals. New York: Plenum Press; p. 29–60.
- Holmes MJ, Lewis RJ. 1994. Purification and characterisation of large and small maitotoxins from cultured Gambierdiscus toxicus. Natural Toxins. 2:64–72. doi: 10.1002/nt.2620020204
- Illoul H, Hernández FR, Vila M, Adjas N, Younes AA, Bournissa M, Koroghli A, Marouf N, Rabia S, Ameur FLK. 2012. The genus Ostreopsis along the Algerian coastal waters (SW Mediterranean Sea) associated with a human respiratory intoxication episode. Cryptogamie Algologie. 33:209–216. doi: 10.7872/crya.v33.iss2.2011.209
- Kang NS, Jeong HJ, Lee SY, Lim AS, Lee MJ, Kim HS, Yih W. 2013. Morphology and molecular characterization of the epiphytic benthic dinoflagellate Ostreopsis cf. ovata in the temperate waters off Jeju Island, Korea. Harmful Algae. 27:98–112. doi: 10.1016/j.hal.2013.05.006
- Lammer E, Carr GJ, Wendler K, Rawlings JM, Belanger SE, Braunbeck T. 2009. Is the fish embryo toxicity test (FET) with the zebrafish (Danio rerio) a potential alternative for the fish acute toxicity test? Comp Biochem Phys C: Toxicol Pharma. 149:196–209.
- Lewis RJ, Holmes MJ. 1993. Origin and transfer of toxins involved in ciguatera. Com Biochem Physiol C: Pharm, Toxicol Endocrinol. 106:615–628.
- Munday R.. 2014. Toxicology of seafood toxins: a critical review. In: Botana LM, editor. Seafood and freshwater toxins: pharmacology, physiology, and detection. Boca Raton, FL: CRC Press; p. 197–290.
- Newcombe RG. 1998. Interval estimation for the difference between independent proportions: comparison of eleven methods. Stat Med. 17:873–890. doi: 10.1002/(SICI)1097-0258(19980430)17:8<873::AID-SIM779>3.0.CO;2-I
- Nipper MG, Martin ML, Williams EK. 1997. The optimisation and validation of a marine toxicity test using the New Zealand echinoid, Fellaster zelandiae. Aust J Ecotoxicol. 3:109–115.
- OECD. 1992. Test no. 203: fish, acute toxicity test. Paris: OECD Publishing.
- OECD. 2013. Test no. 236: fish embryo acute toxicity (FET) test. Paris: OECD Publishing.
- Pagliara P, Caroppo C. 2012. Toxicity assessment of Amphidinium carterae, Coolia cfr. monotis and Ostreopsis cfr. ovata (Dinophyta) isolated from the northern Ionian Sea (Mediterranean Sea). Toxicon. 60:1203–1214. doi: 10.1016/j.toxicon.2012.08.005
- Parsons ML, Aligizaki K, Bottein M-YD, Fraga S, Morton SL, Penna A, Rhodes L. 2012. Gambierdiscus and Ostreopsis: reassessment of the state of knowledge of their taxonomy, geography, ecophysiology, and toxicology. Harmful algae. 14:107–129. doi: 10.1016/j.hal.2011.10.017
- Privitera D, Giussani V, Isola G, Faimali M, Piazza V, Garaventa F, Asnaghi V, Cantamessa E, Cattaneo-Vietti R, Chiantore M. 2012. Toxic effects of Ostreopsis ovata on larvae and juveniles of Paracentrotus lividus. Harmful Algae. 18:16–23. doi: 10.1016/j.hal.2012.03.009
- Puiseux-Dao S, Edery M. 2006. The medaka fish: an experimental model in environmental toxicology its use for the survey of microalgal toxins: phycotoxins and cyanotoxins. In: Arapis G, Goncharova N, Baveye P, editors. Ecotoxicology, ecological risk assessment and multiple stressors. Dordrecht, Netherlands: Springer; p. 227–241.
- R Core Team. 2013. R: a language and environment for statistical computing. Vienna: R Foundation of Statistical Computing.
- Rhodes L, Giménez Papiol G, Smith K, Harwood T. 2014a. Gambierdiscus cf. yasumotoi (Dinophyceae) isolated from New Zealand’s sub-tropical northern coastal waters. New Zeal J Mar Fresh Res. 48:303–310. doi: 10.1080/00288330.2013.870220
- Rhodes L, Smith K, Papiol GG, Adamson J, Harwood T, Munday R. 2014b. Epiphytic dinoflagellates in sub-tropical New Zealand, in particular the genus Coolia Meunier. Harmful Algae. 34:36–41. doi: 10.1016/j.hal.2014.02.004
- Rhodes LL, Smith KF, Munday R, Selwood AI, McNabb PS, Holland PT, Bottein M-Y. 2010. Toxic dinoflagellates (Dinophyceae) from Rarotonga, Cook Islands. Toxicon. 56:751–758. doi: 10.1016/j.toxicon.2009.05.017
- Ritz C, Streibig JC. 2005. Bioassay analysis using R. J Stat Softw. 12:1–22. doi: 10.18637/jss.v012.i05
- Selwood AI, van Ginkel R, Harwood DT, McNabb PS, Rhodes LR, Holland PT. 2012. A sensitive assay for palytoxins, ovatoxins and ostreocins using LC-MS/MS analysis of cleavage fragments from micro-scale oxidation. Toxicon. 60:810–820. doi: 10.1016/j.toxicon.2012.05.024
- Selwood A, Rhodes L, Smith K, Harwood DT. 2014. Development of two novel UPLC-MS/MS methods for the analysis of maitotoxin from micro-algal cultures. In: Mackenzie A, editor. International Conference on Harmful Algae; p. 66–69.
- Shears NT, Ross PM. 2009. Blooms of benthic dinoflagellates of the genus Ostreopsis; an increasing and ecologically important phenomenon on temperate reefs in New Zealand and worldwide. Harmful Algae. 8:916–925. doi: 10.1016/j.hal.2009.05.003
- Tubaro A, Durando P, Del Favero G, Ansaldi F, Icardi G, Deeds JR, Sosa S. 2011. Case definitions for human poisonings postulated to palytoxins exposure. Toxicon. 57:478–495. doi: 10.1016/j.toxicon.2011.01.005
- Vasconcelos V, Azevedo J, Silva M, Ramos V. 2010. Effects of marine toxins on the reproduction and early stages development of aquatic organisms. Mar drugs. 8:59–79. doi: 10.3390/md8010059