ABSTRACT
As historically described, the genus Amphidinum has long been recognized to be polyphyletic. Amphidinium sensu lato is the most diverse of all marine benthic dinoflagellate genera and after the redefinition of the sensu stricto genus about 100 species remained of unknown generic affiliation. A species similar to the Australian sensu lato species Amphidinium boggayum was discovered in France. Morphological and molecular phylogenetic data supported the description of a new species and the classification of the two closely related species within a new athecate, photosynthetic, benthic genus, named Bindiferia. Cells were dorsoventrally flattened with smaller asymmetric epicone. The cingulum was descending. The sulcus reached the antapex and had an extension onto the epicone. The apical structure complex formed an anticlockwise loop around the apex. A red stigma was located in the anterior part of the epicone, near the apex. Vegetative division occurred in a non-motile stage within a hyaline sheath. Non-motile cells had dorsal hypocone grooves. The two species differed from each other in epicone size, cingulum displacement, nucleus location and surface groove occurrence. The molecular phylogenetic results showed that Bindiferia is a distinct taxon that is only distantly related to Amphidinium sensu stricto. The nearest sister group to Bindiferia could not be reliably determined, but the apical structure complex suggested a close relationship to the genera of the Gymnodinium sensu stricto clade.
INTRODUCTION
The genus Amphidinium Claparède & J. Lachmann was among the largest and most diverse of all marine benthic dinoflagellate genera, containing about 120 species (e.g. Murray & Patterson Citation2002), but the genus was found to be polyphyletic (Flø Jørgensen et al. Citation2004a). Modern methods have been used to re-investigate the type species of the athecate genera Gymnodinium F. Stein and Gyrodinium Kofoid & Swezy (Daugbjerg et al. Citation2000; Hansen et al. Citation2000; Hansen & Daugbjerg Citation2004; Takano & Horiguchi Citation2004). More precise re-definitions of these genera have caused many of the species formerly assigned to them to be considered ‘sensu lato (s.l.) taxa’, i.e. taxa without generic affiliation. To distinguish Amphidinium from other athecate genera, overly generalized criteria such as epicone dimensions (shorter than ⅓ of the cell length) and cingulum displacement were used in the past. After re-investigations of A. operculatum Claparède & J. Lachmann (the type species) and putative relatives, the genus Amphidinium was also re-defined as dorsoventrally flattened, athecate dinoflagellates with a minute epicone that overlays the anterior ventral part of the hypocone and deflects to the left (Murray et al. Citation2004; Flø Jørgensen et al. Citation2004a). The epicone can be irregular triangular-shaped or crescent-shaped. Cells may or may not be photosynthetic. Of the taxa previously classified within Amphidinium, only about 20 species fell into the Amphidinium Claparède & J. Lachmann emend. M.F. Jørgensen, Sh. Murray & Daugbjerg ‘sensu stricto’ (s.s.) definition, leaving about 100 species of uncertain or unknown generic affiliation (Murray Citation2003). Recently, six new Amphidinium s.s. species were described using a multiple evidence approach (Karafas et al. Citation2017). Six new genera have been characterized already, Togula M.F. Jørgensen, Sh. Murray & Daugbjerg, Prosoaulax Calado & Moestrup, Apicoporus Sparmann, B.S. Leander & Hoppenrath, Ankistrodinium Hoppenrath, Sh. Murray, Sparmann & B.S. Leander, Testudodinium T. Horiguchi, Maiko Tamura, Katsumata & A. Yamaguchi and Nusuttodinium Y. Takano & T. Horiguchi emend. R. Onuma & T. Horiguchi (Flø Jørgensen et al. Citation2004b; Calado & Moestrup Citation2005; Sparmann et al. Citation2008; Hoppenrath et al. Citation2012; Horiguchi et al. Citation2012; Takano et al. Citation2014; Onuma et al. Citation2015), of which four are marine benthic genera and one contains benthic species (including its type). Amphidinium pellucidum Herdman was transferred into the genus Gymnodinium as G. venator M.F. Jørgensen & Sh. Murray (Flø Jørgensen et al. Citation2004a, Citationc). More recently, newly discovered taxa with Amphidinium s.l. morphology were directly described as type species of new genera, like Bispinodinium angelaceum Norico Yamada & T. Horiguchi and Pellucidodinium psammophilum R. Onuma & T. Horiguchi (Yamada et al. Citation2013; Onuma et al. Citation2015).
The genus selection for Amphidinium boggayum Sh. Murray & D.J. Patterson was an arbitrary choice based on the cingulum placement in relation to the cell apex (Murray & Patterson Citation2002). The species would have also met criteria for a classification within Gymnodinium s.s. (Daugbjerg et al. Citation2000). Later, it has been hypothesized by Flø Jørgensen et al. Citation(2004a) that A. boggayum belongs to Gymnodinium but without formal transfer because of missing statistical support in their cladistic analysis of morphological characters and the lack of molecular phylogenetic data.
During biodiversity studies of benthic dinoflagellates in Brittany, France (Hoppenrath et al. Citation2013) and Broome, NW Australia (Murray et al. Citation2006a, Citationb; Kohli et al. Citation2014; Verma et al. Citation2019), cells similar to Amphidinium boggayum were observed and isolated for taxonomical re-investigation of this ‘nameless’ (without generic identity) Amphidinium s.l. taxon and to classify it at genus level. A strain similar to A. boggayum isolated from New Zealand (CAWD164, Rhodes et al. Citation2010) was analysed in this study to determine whether specimens from other regions were similar morphologically and genetically to one another.
MATERIAL AND METHODS
Sampling
For Bindiferia fragilissima sp. nov., intertidal sand was collected 8 September 2010 at Le Letty, ‘Mer Blanche’ (kind of lagoon with small opening to the Atlantic) close to Bénodet, France (47°51.52ʹN, 4°5.04ʹW). The samples were taken from the surface (upper 2 cm) and dinoflagellates were extracted by the seawater ice method (Uhlig Citation1964; Hoppenrath et al. Citation2014).
For Bindifera boggaya comb. nov., intertidal sand samples were collected at Town Beach, Broome (17°58.08ʹS, 122°14.16ʹE), in the tropical north of Western Australia, in September 2003 and in May 2011. The sediment water temperature ranged from 22°C to 29°C and the salinity ranged from 38 to 40 during the sampling period. Samples were also collected from Port Botany, Sydney (33°58.08ʹS, 151°12.57ʹE) in June 2003. The first 2 cm or 0.5 cm of sediment were sampled at low tide using a flat spoon (Murray & Patterson Citation2002).
Culturing
For Bindifera fragilissima sp. nov., single cells were isolated by micro-pipetting, washed in filtered seawater and then transferred into medium. Clonal cultures were maintained at 19°C under a photon flux density of 6.5 μmol m−2 s−1 and 12:12 h (light:dark) cycle in f/2 medium (Guillard & Ryther Citation1962) prepared with sterile filtered seawater, salinity 32, in Germany. A cultured strain identified as A. boggayum, SM20, was established from a sample collected in Port Botany in June 2003 by picking cells under a Leica DMR light microscope. A single cell was washed in a series of filtered seawater. The strain was maintained in f/2 medium, with a 12:12 h (light:dark) cycle at 20°C, with a photon flux density of c. 60 μmol m−2 s−1.
A cultured strain, CAWD164, established from a surface sediment sample collected from Rangaunu Harbour, New Zealand (34°58ʹ S, 173°16ʹ E) in February 2009 was identified as Amphidinium boggayum during this study. CAWD164 strain was cultured in f/2 medium at 25°C, with a 12:12 h (light:dark) cycle under a photo flux density of 40–70 μmol m−2 s−1.
Two cultured strains of Togula jolla M.F. Jørgensen, Sh. Murray & Daugbjerg (CAWD41 and CAWD58) from the Cawthron Collection were additionally sequenced in this study for comparison with Bindifera. These strains were originally isolated from New Zealand sand samples, CAWD41 from Awaroa Inlet (Abel Tasman, South Island) and CAWD58 from Napier (North Island).
Microscopy
Living cells of both species were picked using a Leica DMIL inverted microscope (Leica Microsystems GmbH, Wetzlar, Germany), placed on an object slide and observed with a Leica DMRB equipped with differential interference contrast optics at 400× and 640× magnification with oil immersion objectives in Germany. Digital micrographs were taken using a Leica DFC290 and DFC420C camera. A Leica DMi8 inverted microscope with a Leica DFC7000T camera (Leica Microsystems GmbH, Wetzlar, Germany) was used to document cells in the culture Petri dishes.
Bindifera boggaya comb. nov. cells were observed and photographed using a Leica DMR light microscope (Leica Microsystems GmbH, Wetzlar, Germany), a Zeiss Axiophot microscope (Zeiss, München-Halbergmoos, Germany) with differential interference contrast optics and a Zeiss Axiocam digital camera in Australia. An Olympus IX73 inverted microscope with DP27 5 megapixel digital camera (Olympus Pty Ltd, Melbourne, Australia) was used in New Zealand.
For scanning electron microscopy (SEM) of B. boggaya, cells were pipetted individually from samples, rinsed three times in filtered seawater and placed on polylysine-coated coverslips. They were fixed in 2% osmium tetroxide in seawater for 20 min, rinsed in distilled water and dehydrated in a series of increasing ethanol concentrations (15, 30, 50, 70, 90, 100%). The cells were critical point dried, sputter coated with gold and observed using a Phillips 505 or a JEOL 840 scanning electron microscope at 5–20 kV.
At least seven fixation protocols were tested for SEM of the French isolate but none was successful. In most cases no cells could be observed because they burst during fixation or at the final dehydration steps. In one case, the few potential cells we found were completely covered by mucus. In a transmission electron microscopic (TEM) preparation only a mess of unordered membranes and vesicles were visible.
DNA extraction, PCR amplification and sequencing
For DNA amplification of Bindiferia fragilissima (French culture), and since it was difficult to obtain sequences from extracted DNA, a few cells (10–20) were isolated using a capillary pipette and put directly in a PCR tube. A direct amplification was realized using a nested PCR approach. A first round allowed the amplification of a long amplicon (covering SSU rDNA and partial LSU rDNA) using 18SFW and Dino-ND primers (Hansen & Daugbjerg Citation2004; Chomérat et al. Citation2010). It was then possible to obtain sequences for both genes using the internal primers described in Chomérat et al. (Citation2010). The KOD Xtreme hot start DNA polymerase (Novagen) has been used according to the manufacturer’s recommendations. The thermal cycles were one initial denaturing step at 94°C for 2 min, 35 cycles of 98°C for 10 s, 52°C for 30 s and 68°C for 3 min.
A volume of 10–80 ml of culture of B. boggaya (SM20) was centrifuged for 10 min at 1500–3000 rpm and the pellet transferred to a 1.5-ml Eppendorf tube. DNA was extracted using a modified CTAB method (Flø Jørgensen et al. Citation2004a). Approximately 1450 bp of the LSU rDNA covering the variable domains D1–D6 were amplified using the primers D1R (Scholin et al. Citation1994) and 28–1483 R (Daugbjerg et al. Citation2000); the PCR reaction mixture consisted of 2.5 mM MgCl2, 1 unit of DNA polymerase (Bioline, Astral Scientific, Caringbah, Australia), 10× NH4 buffer, 0.2 mM of mixed dNTPs and 10 pmol of primers. The thermal cycles were one initial denaturing step at 94°C for 3 min, 35 cycles of 94°C for 30–60 s, 50–55°C for 30–60 s and 72°C for 1–3 min and a final cycle of 72°C for 5–6 min.
To prepare for extraction, cultures of B. boggaya (CAWD164) and Togula jolla (CAWD41 and CAWD58) were centrifuged (50 ml, 3000× g, 10 min) and the supernatant decanted, with pelleted cells frozen until further extraction. DNA was extracted using the DNeasy® Powersoil® DNA isolation kit (Qiagen) following the protocol for the automated DNA extraction robot QIAcube® (Qiagen, Carlsbad, USA). The D1–D3 region of the LSU rDNA was amplified using the primer pair D1R and D3B (Nunn et al. Citation1996), a partial region of the SSU rDNA was amplified using EUKA-EUKB primers (Medlin et al. Citation1988) and the internal transcribed spacer (ITS) region was amplified with the ITSA and ITSB primer pair (Adachi et al. Citation1994). Each PCR reaction master mix included 25 µl MyTaq 2x PCR mix (Bioline, Massachusetts, USA), 2 µl of both forward and reverse primers, 2 µl bovine serum albumin (BSA) and 50–100 ng of template DNA for a total reaction volume of 50 µl. For the primer pair for domains D1–D3 the thermo cycle included an initial denaturing step of 95°C for 4 min, followed by 35 cycles of 95°C for 30 s and 60°C for 2 min, with a final extension of 72°C for 10 min. All other primer pairs thermo cycle conditions included an initial denaturing step of 95°C for 2 min, 35 cycles of 95°C for 30 s, 54°C for 30 s and 72°C for 1 min, with final extension of 72°C for 5 min. Resulting PCR products were purified using the AxyPrep PCR clean-up kits (Axygen, California, USA) then sent to Genomic Analysis services (GAS, University of Otago, New Zealand) for direct sequencing in both directions. Purified PCR product for isolate CAWD164 SSU was sent to Macrogen (Geumcheon-gu, Seoul, South Korea) for commercial cloning as direct sequencing of this region was ineffective. Returned forward and reverse sequences were trimmed using Geneious v9.1.8 (Kearse et al. Citation2012), aligned using the ClustalW algorithm (Thompson et al. Citation1994) in Geneious and manually inspected for conflicts.
Alignment and phylogenetic analyses
All new sequences were deposited in GenBank (). Phylogenetic analyses were performed using initial alignments previously developed (Orr et al. Citation2012; Murray et al. Citation2015). Additional dinoflagellate sequences were obtained and added as described above, or downloaded from the GenBank reference database (www.ncbi.nlm.nih.gov). Each rRNA gene (18S, ITS1-5.8S-ITS2 and 28S) was separately aligned using the MUSCLE algorithm (Edgar Citation2004) as implemented in Geneious Prime. Outgroup taxa were established from previous phylogenies (Murray et al. Citation2005; Orr et al. Citation2012). Single gene alignments were checked manually using Geneious Prime, and poorly aligned regions were removed. Alignments were then concatenated, consisting of 114 taxa and 2880 characters, and will be made available under Shauna Murray’s profile on ResearchGate.
Table 1. Accession numbers for newly determined rDNA sequences of Bindiferia boggaya, B. fragilissima and Togula jolla collected at different sites
Phylogenetic trees were calculated with Bayesian Inference (BI) and Maximum Likelihood (ML). The model with the highest Bayesian Information criterion score for the alignment was determined using ModelFinder (Kalyaanamoorthy et al. Citation2017), and was found to be a GTR with a proportion of invariant sites and 4 gamma rate categories, and was used for phylogenetic analyses.
Maximum likelihood (ML) phylogenetic trees were generated using IQtree (Nguyen et al. Citation2015) with 1000 bootstraps. Bayesian Inference (BI) was performed to estimate Posterior Probability (PP) distribution using MrBayes 3.2.6 with Metropolis-Coupled Markov chain Monte Carlo simulations (Ronquist & Huelsenbeck Citation2003). A random starting tree with 4 heated and one cold chain was used with temperature set at 0.2. Trees were sampled every 500 generations for 1,500,000 generations and the first 500,000 generations were discarded as burn-in. Phylogenies were visualized using FigTree (http://tree.bio.ed.ac.uk/software/figtree/) and Adobe Illustrator.
RESULTS
Taxonomy
Bindiferia Borchhardt, Chomérat, Sh. Murray & Hoppenrath gen. nov.
Description
Athecate, dorsoventrally flattened cells with smaller asymmetric epicone in comparison to the hypocone. Cingulum descending. Sulcus reaching the antapex and extending onto the epicone. Red stigma anterior in the epicone, close to the apex. It is likely that an apical structure complex forming an anticlockwise loop around the apex is also a generic feature but this could not be confirmed for the type yet.
Etymology
‘Bindi’ is a traditional decoration in India; a red drop, which is applied in the centre of the forehead and symbolizes an eye; ‘feria’ from Latin verb fero, ferre, to carry, to wear. The genus is feminine in gender.
Registration
Type Species
Bindiferia fragilissima Borchhardt, Chomérat & Hoppenrath.
Bindiferia fragilissima Borchhardt, Chomérat & Hoppenrath sp. nov.,
Figs 1–13. Light micrographs of Bindiferia fragilissima sp. nov. from France. Scale bars = 10 µm.
Figs 1, 2. Same actively motile cell in ventral view in different focal planes showing the sulcal path and cingulum; note the red stigma close to the apex (arrow) and the posterior nucleus (n).
Fig. 3. Actively motile cell in ventral view showing the sulcal path and cingulum; note the red stigma close to the apex (arrow).
Figs 4, 5. Same actively motile cell in ventral view showing the sulcal path including the extension onto the epicone (short arrow); note the red stigma close to the apex (arrow) and the length of the longitudinal flagellum.
Fig. 6. ‘Stationary motile’ cell; note the red stigma (arrow).
Fig. 7. ‘Stationary motile’ cell with two dorsal longitudinal grooves on the hypocone (arrowheads).
Figs 8, 9. Two ‘stationary motile’ cells with three dorsal longitudinal grooves on their hypocone (arrowheads); note the red stigma (arrow).
Fig. 10. Non-motile cell in ventral view covered by a hyaline layer (double arrowhead); note the red stigma (arrow).
Fig. 11. Non-motile division stage covered by a hyaline double-layer (double arrowhead); note the red stigma (arrow).
Fig. 12. Non-motile division stage with daughter cells starting to separate posteriorly, covered by a hyaline double-layer (double arrowhead); note the red stigma (arrow).
Fig. 13. Already motile, nearly divided, but still apically connected daughter cells outside the division cyst
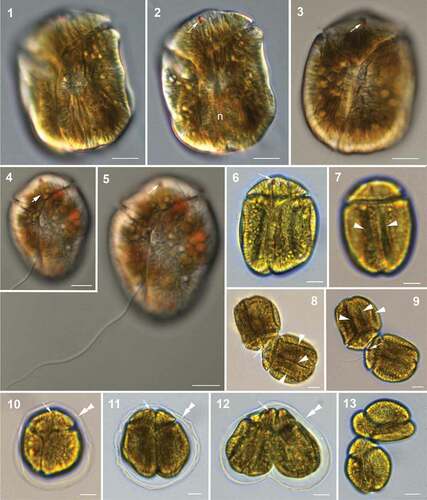
Fig. 14. Hypothetical vegetative life cycle (cell division) of Bindiferia fragilissima sp. nov. Free-swimming cells (a) have a smooth cell surface (lower illustrations) and sometimes many extrusomes can be visible along the cell periphery (upper illustrations). Stationary motile cells develop dorsal grooves (b) and get enclosed by a hyaline sheath (c). Vegetative binary fission takes place in the division cyst, with a hyaline sheath (d, e) and motile nearly divided but still apically connected daughter cells without surface grooves get released from the sheath (f). Smaller free-swimming cells with smooth surface (g) start growing
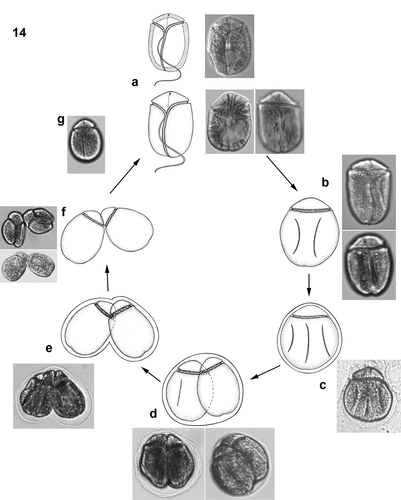
Description
Athecate, dorsoventrally flattened, oval to oblong cells, 30–65 μm (50.9 μm ± 2.6, n = 44) long, 25–50 μm (41.4 μm ± 1.6, n = 44) wide and approximately 30 µm deep. The smaller asymmetric epicone was 0.2 of the total cell length and slightly narrower than the total cell width (). The cingulum descends about two cingular widths (, ). The longitudinal flagellum is about 1.5–2× the cell length (). The narrow sulcus reaches the antapex after curving slightly to the right and it extends onto the epicone in a straight line (, , , ). The apical structure complex could not be observed. Oval to round nucleus located in the lower (sometimes left) hypocone half (). Golden-brown elongated chloroplasts scattered throughout the cell (, S1). The visible elongated rods may be part of a (single?) chloroplast mesh (Fig. S1). A small (3.3–4.3 μm long, n = 10; 2.0–3.8 μm wide, n = 30), oval or rod-shaped, red stigma is located in the anterior-ventral part of the epicone, near the apex (, , , , ). After cells burst it looked like a separate structure not connected to any chloroplast. One or rarely two or several small, orange putative food bodies can be present, often visible above the nucleus. Many extrusomes can be visible along the cell periphery (, , upper image). Free-swimming cells can become stationary and rapidly change into a non-motile stage that is covered by a hyaline layer (under a coverslip). Motile cells are of the described morphology, see above (, ). ‘Stationary motile’ cells are able to swim but do not swim. These cells were wider and usually had two or three longitudinal grooves on the dorsal side of the hypocone (, ). Non-motile cells are sheathed by an irregular hyaline layer, are roundish and usually have three longitudinal grooves on the dorsal side of the hypocone (, ). On average they are larger, 45–63 μm long (55.5 μm ± 4.8, n = 20) and 45–55 μm wide (50.4 μm ± 3.8, n = 20). Vegetative cell division takes place during the non-motile stage (, , , ). In the hyaline sheath (temporary division cyst) also double divisions can happen, resulting in up to four cells per cyst. During ‘normal’ (= not multiple) division daughter cells are in the cyst most of the time and have one or two longitudinal grooves on the hypocone (, ). The cell division starts at the antapex and the last parts to separate are the epicones (, , , ). The final daughter cell separation takes place outside the cyst (, ). The still connected cells are already able to swim. After division smaller cells start growing (). The cyst wall can have a double layer appearance () and can persist for some time (shown on the Petri dish bottom under culture conditions, Fig. S2). The life cycle still needs further investigation.
Holotype
cultured cells preserved in ethanol (Art. 44.2 of the ICN; Turland et al. Citation2018) deposited at the dinoflagellate type collection in the Centre of Excellence for Dinophyte Taxonomy (CEDiT, Wilhelmshaven, Germany), which is part of the Herbarium Senckenbergianum Frankfurt/M. (FR) with the designation CEDiT2021H135.
Representative Figures
, (same cell).
Type Locality
Le Letty, ‘Mer Blanche’ close to Bénodet, France (47°51.847ʹN, 4°5.054ʹW)
Etymology
Latin adjective fragillissimus, -a, -um, the most fragile, very fragile; because of the difficulty to work with vegetative cells‚ exploding all the time.
Registration
Molecular Characterization
MZ233647 (SSU rRNA gene region), MZ233677 (LSU rRNA gene region).
Authentic Strain
Culture deposited at the Culture Collection of Algae and Protozoa (CCAP), strain designation CCAP 1100/1.
Habitat
marine sandy sediments.
Bindiferia boggaya (Sh. Murray & D.J. Patterson) Sh. Murray & Hoppenrath comb. nov.
Basionym
Amphidinium boggayum Sh. Murray & D.J. Patterson in Murray & Patterson Citation2002, European Journal of Phycology 37: 280, figs 2–7.
Registration
Emended Description
Athecate dorsoventrally flattened, oval to oblong cells (, , ), 39–58 µm long, 25–45 µm wide and approximately 22 µm deep. Smaller epicone asymmetrical, apex slightly to the left cell side (, ). It was originally described that the proximal cingulum end originates 0.5–0.6 of the cell length from the apex, rising vertically to 0.2–0.3 of the cell length from the apex, then turning left and continuing at a slightly upward angle on the ventral side (, , ). In the present study (after further observations) it is concluded that the cingulum descends about four cingular widths. The furrow becomes narrow where the ends meet (, ). The longitudinal flagellum arises below the proximal cingulum end. The narrow sulcus curves to the right towards the posterior (, ), reaches and sometimes slightly indents the antapex (), and extends onto the epicone (, ). The apical structure complex is connected to the sulcal extension and forms an anticlockwise loop around the apex (, ). Oval nucleus located in the epicone (, , ). Numerous golden-brown chloroplasts scattered throughout the cell (). A small red stigma in the anterior-ventral part of the epicone, near the apex (, ). Small, reddish, putative food bodies can be present (, , , ). Many extrusomes can be visible along the cell periphery (). Free-swimming cells can become stationary and rapidly change into a non-motile stage that is covered by a hyaline layer (). Non-motile cells are more rounded in shape than swimming cells (, ). Vegetative cell division takes place during the non-motile stage (, , , ). Two or four daughter cells per cyst can develop (, , , ). Surface grooves were not observed.
Figs 15–25. Light (15, 16, 19–25) and scanning electron (17, 18) micrographs of Bindiferia boggaya comb. nov. from Australia. Scale bars = 10 µm.
Figs 15–18. Cells from Sydney, Australia, from the original description (Murray & Patterson Citation2002).
Fig. 15. Cell in ventral view showing the cingulum and sulcus paths, note the sulcal extension (arrow).
Fig. 16. Cell in mid-focus showing the anteriorly located nucleus (n).
Fig. 17. Cell in ventral view showing the cingulum and sulcus paths; note the sulcal extension (arrow). The small arrowheads indicate the beginning and end points of the apical structure complex.
Fig. 18. Apical view of the epicone showing the loop-shaped apical structure complex (arrowheads).
Figs 19–25. Cells from Broome, Australia.
Fig. 19. Motile cell in ventral view; note the red stigma close to the apex (arrow) and possibly a food body (asterisk).
Fig. 20. Motile cell in dorsal view; note the anterior nucleus (n).
Figs 21, 22. Vegetative division stage in a hyaline sheath in different focal planes; note the red stigma (arrow), the nuclei (n) and possible food bodies (asterisk).
Figs 23–25. Non-motile cell in a hyaline sheath in different focal planes (23, ventral; 24 mid-focus; 25, dorsal); note the red stigma (arrow), the anterior nucleus (n) and a possible food body in the posterior area of the hypocone (asterisk). The dorsal hypocone surface is smooth, without grooves
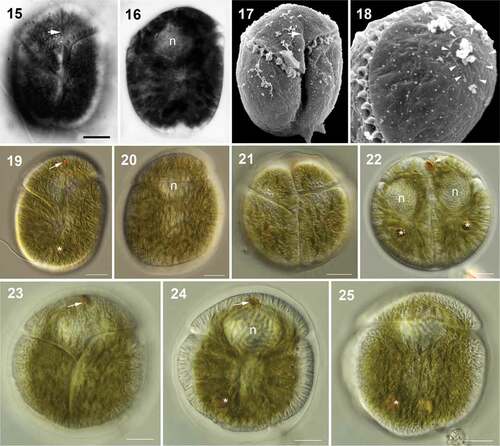
Figs 26–31. Division stages within a hyaline sheath of Bindiferia boggaya comb. nov. from Broome, Australia (26–28) and New Zealand (29–31). Scale bars = 10 µm.
Figs 26, 29. Non-motile, rounded cell; note the stigma (arrow).
Figs 27, 30. Two daughter cells in the division cyst; note a stigma (arrow).
Figs 28, 31. Four daughter cells in the division cyst
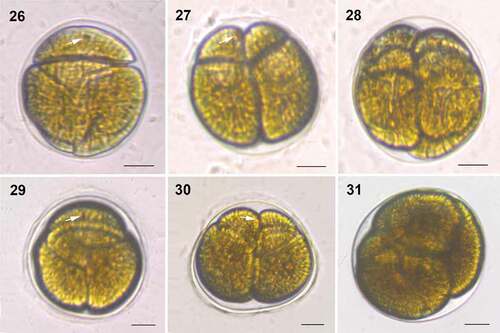
Molecular Characterization
MW720768 (SSU rRNA gene region), MW722979, MW720712 (LSU rRNA gene region).
Strain
Cawthron Institute Culture Collection of Microalgae (CICCM), accession CAWD164.
Habitat
marine sandy sediments.
Distribution
Sydney (Quibray Bay, Port Botany), Durras Lake, Broome (Town Beach), Australia (Murray & Patterson Citation2002; Murray & Hoppenrath, unpublished observations); Rangaunu Harbour, New Zealand.
Phylogenetic inferences
The genus Bindiferia, including the two new species, was found to be monophyletic with very high support (97/0.98 in ML BS/BI PP, respectively; ). The two strains of B. boggaya from Australia and New Zealand clustered together with full support. The species B. boggaya was found to be on a very long branch in the phylogeny, of a similar length to that of species of Gonyaulacales ().
Fig. 32. Molecular phylogeny of the dinoflagellates, including the new sequences of Bindiferia and Togula, based on a concatenated alignment of 18S, ITS1, 5.8S, ITS2 and 28S rRNA gene regions. Most likely tree shown, with support values based on maximum likelihood bootstrap analysis (ML BS) and Bayesian inference posterior probabilities (BI PP)
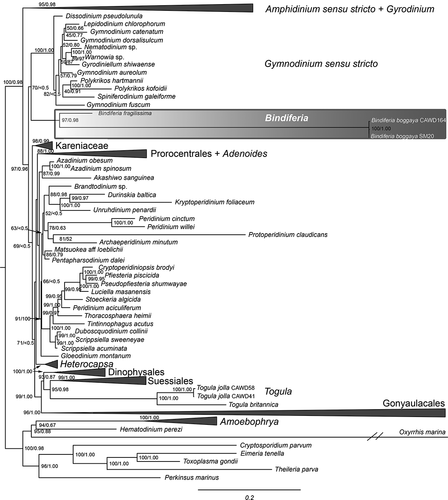
The genus Bindiferia was found to be the sister group to the clade of the genus Gymnodinium s.s., including the type species G. fuscum (Ehrenberg) F. Stein, and closely related genera such as Lepidodinium M.M. Watanabe, S. Suda, I. Inouye, Sawaguchi & Chihara, Gyrodiniellum N.S. Kang, H.J. Jeong & Moestrup, Nematodinium Kofoid & Swezy, Warnowia Er. Lindemann, Polykrikos Buetschli and Spiniferodinium T. Horiguchi & Chihara with moderate/low support in the ML BS analysis (70%). However, this topology was not supported by more than 50% in the BI PP analysis ().
In general, the phylogenetic analysis found that dinoflagellates are strongly supported as monophyletic, and that unarmoured dinoflagellates, particularly the genera Amphidinium s.s. and Gyrodinium, were the most basal of the ‘core’ dinoflagellates. The major orders such as the Prorocentrales, Suessiales, Dinophysales, Gonyaulacales and Peridiniales were all supported.
DISCUSSION
When describing Amphidinium boggayum, Murray & Patterson (Citation2002) discussed that its placement in the genus was an arbitrary choice based on the cingulum placement in relation to the cell apex. Similar Amphidinium species had already been described, e.g. A. asymmetricum Kofoid & Swezy, A. corpulentum Kofoid & Swezy, A. britannicum (Herdman) M. Lebour, or A. ovum Herdman (Kofoid & Swezy Citation1921; Herdman Citation1922, Citation1924). The species would have also met criteria for a classification within the redefined genus Gymnodinium s.s. (Daugbjerg et al. Citation2000), like the type of the apical structure complex.
In the original description A. boggayum (which was based on cells isolated from field samples that were not cultured) the small apical stigma was not recognized (Murray & Patterson Citation2002; , ). Later, in cells from samples taken in Broome, Australia, the stigma was observed and documented (Hoppenrath et al. Citation2014; ) and also specimens from the Sydney area show this feature (S.A. Murray, unpublished observations). Division stages were not described before the present study.
The newly described species B. fragilissima is morphologically very similar to ‘A’. boggayum (Murray & Patterson Citation2002). Both species are athecate, dorsoventrally flattened, with smaller and narrower asymmetric epicone. The cingulum is descending. The sulcus is narrow, not covered by a flap and curving to the right towards the posterior, reaching the antapex. A sulcal extension runs straight onto the epicone towards the apex. A red stigma is visible at an anterior-ventral location in the epicone, close to the apex. Both species divide as non-motile cells in hyaline temporary cyst stages. Bindiferia fragilissima can be distinguished from ‘A’. boggayum by its relatively shorter epicone, its less displaced cingulum and the nucleus located in the hypocone instead of the epicone (). Furthermore, division stages of B. fragilissima showed longitudinal surface grooves on their hypocone that were not recorded for ‘A’. boggaya.
Figs 33–38. Line drawings of Bindiferia fragilissima (33–35) and B. boggaya (36–38). fb, food body; n, nucleus; arrows indicate stigma; arrowheads indicate sulcal extension.
Figs 33, 34, 36, 38. Ventral views.
Figs 35, 37. Dorsal views.
Fig. 38. Drawing from the original description (Murray & Patterson Citation2002)
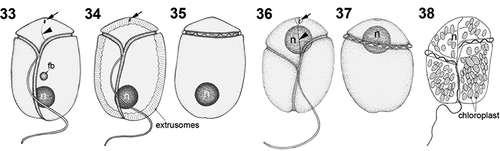
The phylogenetic analysis supported the sister group relationship of the two species and thus ‘A’. boggayum has been transferred to the new genus Bindiferia (). The long branch of the B. boggaya clade suggests either that this might be an ancient group of dinoflagellates, or that it has exhibited much more rapid evolutionary rates than other dinoflagellate genera in ribosomal RNA genes. Strong rate heterogeneity in rRNA genes has been observed in multiple dinoflagellate lineages, particularly the Gonyaulacales (John et al. Citation2003; Murray et al. Citation2005; Kretzschmar et al. Citation2019; Žerdoner Čalasan et al. Citation2019). The order level relationships of the genus Bindiferia could not be resolved with certainty in this analysis, as the sister group relationship with Gymnodinium lacked support (). This suggests that more molecular sequence data, particularly from other genes, would be needed to resolve this topology with certainty.
Both species can be distinguished from similar photosynthetic Amphidinium s.l. species described from the same habitat. ‘Amphidinium’ asymmetricum cells were described with a similar cell shape, similar size range and with a longer left side of the hypocone and of the whole cell (Kofoid & Swezy Citation1921). However, ‘A’. asymmetricum was described with surface striations, no sulcal extension and a sulcal flap overhanging to the cell’s right. Also ‘A’. corpulentum and ‘A’. ovum were described with a similar cell shape, a smaller epicone, no surface striations and a sulcal extension onto the epicone, but with a less displaced cingulum, a sulcal flap overhanging to the cell’s right and a lateral position of the nucleus (Kofoid & Swezy Citation1921; Herdman Citation1924; Larsen Citation1985; Larsen & Patterson Citation1990). The sulcal extension in ‘A’. corpulentum is similar to that of Bindiferia (Kofoid & Swezy Citation1921), but it is shorter in ‘A’. ovum (Larsen Citation1985; Larsen & Patterson Citation1990; Al-Yamani & Saburova Citation2010). All these taxa were described without stigma.
Al-Yamani & Saburova (Citation2010) described a photosynthetic species as Gyrodinium sp. that had some similarities to Bindiferia: a descending cingulum, a narrow sulcus without flap and with a long and straight extension onto the epicone up to the apex. It was shown as non-motile cell within a hyaline sheath and had a coloured spot near the apex. However, the colour of this spot was not as red and its size was larger than in Bindiferia, and it is therefore uncertain whether it represented a stigma. The specimens were smaller than the two Bindiferia species. This species needs further investigation to clarify its affiliation.
The phototrophic genus Togula (also a former Amphidinium s.l. taxon), with species having asymmetric, dorsoventrally flattened cells, differs from Bindiferia by having no sulcal extension, no apical structure complex and no stigma (Flø Jørgensen et al. Citation2004b). Interestingly, the species divide in a non-motile stage inside hyaline mucous sheaths and the division stages can have longitudinal grooves on their hypocones (Flø Jørgensen et al. Citation2004b). Togula britannica (Herdman) M.F. Jørgensen, Sh. Murray & Daugbjerg showed a longitudinal groove on the left ventral hypocone prior to cell division. In Togula jolla cell division was preceded by the formation of six to eight longitudinal grooves on the hypocone (first one left-ventral and two dorsal in close succession, followed by additional dorsal). Cell division of Togula species began at the antapex and the last parts to split were the epicones (Flø Jørgensen et al. Citation2004b), as observed in Bindiferia species. The fact that Bindiferia was not closely related to the genus Togula in the phylogenetic tree, and that Togula appeared as a sister group to the clade Suessiales with high support (95/0.98; ) suggests that these two genera are very distinct from one another. Previously, Togula had been resolved with full support as the sister group to the species Gymnodinium catenatum H.W. Graham, and therefore as a member of the Gymnodiniaceae s.s., in phylogenetic analyses based on large multi-gene datasets (Janouškovec et al. Citation2017; Price & Bhattacharya Citation2017). Recent studies of dinoflagellate phylogeny based on large amounts of sequence data have indicated a monophyletic origin of dinoflagellate thecal plates (Orr et al. Citation2012; Bachvaroff et al. Citation2014; Janouškovec et al. Citation2017; Price & Bhattacharya Citation2017). This suggests that this placement of Togula as separate to the Gymnodiniaceae may be an artefact of the use of rRNA genes, or else it may indicate a previously unrecognized relationship with the Suessiales. Future detailed TEM examination of the amphiesmal alveoli of the species of Togula, with the determination of the presence or absence of thin thecal plates, may help clarify the matter.
Surface grooves on hypocones of athecate species are a feature rarely described (Fig. S3). Klebs (Citation1884) described a species he named A. operculatum with ‘furrows’ or corrugations on the cell surface. This observation has not been verified since the original description but this characteristic was the basis for renaming the taxon as a new species, A. klebsii by Kofoid & Swezy (Citation1921). Dividing motile cells of Amphidinium tomasii Karafas showed grooves on the hypocone, but non-dividing cells had a smooth surface (Karafas et al. Citation2017). The heterotrophic Amphidinium pellucidum sensu Dragesco (Citation1965) was described with longitudinal grooves, a morphological character not originally described for A. pellucidum by Herdman (Citation1922) – now re-classified as Gymnodinium venator (Flø Jørgensen et al. Citation2004a, Citationc). The species Testudodinium corrugatum (J. Larsen & D.J. Patterson) T. Horiguchi, Maiko Tamura & A. Yamaguchi has been described with longitudinal dorsal ribs on the hypocone (Larsen & Patterson Citation1990; Horiguchi et al. Citation2012). This structure can be interpreted as longitudinal grooves when focussing the description not on the elevations but the depressions between them. The dorsal corrugations were only described for one of the three Testudodinium species (Horiguchi et al. Citation2012).
The redefinition of Amphidinium s.s. (species with a minute, left-deflected epicone) by Flø Jørgensen et al. Citation(2004a), as a first step to resolve the morphologically diverse and polyphyletic assemblage of taxa previously assembled within Amphidinium s.l., highlighted the need to reinvestigate the taxa excluded from the genus in the restricted sense. This has already been accomplished for several taxa, like Togula (Flø Jørgensen et al. Citation2004b; see above). Also the genera Apicoporus and Ankistrodinium showed novel epicone characteristics that were different from Amphidinium s.s. or any other athecate genus (Sparmann et al. Citation2008; Hoppenrath et al. Citation2012). Apart from the different epicone size and shape, the sulcal extension onto the epicone and the presence of an apical structure complex, the most striking feature of Bindiferia is the stigma close to the apex. A stigma is not known from Amphidinium species, with one exception: A. cupulatisquama Maiko Tamura & T. Horiguchi has a kidney-shaped stigma, located on the dorsal side of cingulum (Tamura et al. Citation2009). The molecular phylogenetic results are consistent with the morphological interpretation and clearly show that Bindiferia is a distinct genus and only distantly related to the genus Amphidinium.
One morphological feature, the anticlockwise loop-shaped apical structure complex, suggests that the genus may be related to genera of the Gymnodinium s.s. clade, e.g. Gymnodinium, Lepidodinium, Polykrikos, Spiniferodinium and Warnowia (Daugbjerg et al. Citation2000; Hoppenrath & Leander Citation2007a, Citationb; Hoppenrath et al. Citation2009; Horiguchi et al. Citation2011). A sister group relationship of this clade to Bindiferia could not be reliably determined with the present molecular phylogenetic analyses. It has been hypothesized by Flø Jørgensen et al. Citation(2004a) that A. boggayum belongs to Gymnodinium. Their cladistic analysis of morphological and ultrastructural characters grouped Bindiferia boggaya (as A. boggayum) within the Gymnodinium clade with no support for this grouping (Flø Jørgensen et al. Citation2004a). Thus, the closest relative of Bindiferia remains unknown.
Moestrup & Daugbjerg (Citation2007) distinguished five stigma types, all of them situated in the sulcal area close to the flagellar roots. A stigma similar to type A (pigment globules inside the peridinin dino-chloroplast) but located on the dorsal side of the cingulum was described for an Amphidinium species (Tamura et al. Citation2009). Another dorsal stigma can be observed in Margalefidinium polykrikoides (Margalef) F. Gómez, Richlen & D.M. Anderson (Iwataki et al. Citation2010, as Cochlodinium). Currently eight different stigma types are known, three of them outside of the chloroplasts (Hoppenrath Citation2017). To the best of our knowledge this is the first report of a stigma located ventrally close to the apex. There are no ultrastructural data available yet (because of technical problems), only the hint from broken cells that the pigments of the stigma stay together as one unit that seems not to be associated with a chloroplast.
Dinoflagellates whose principle life cycle stage is non-motile, with their cell shapes different from the typical motile stages, were collectively called ‘coccoid dinoflagellates’ by Hoppenrath et al. (Citation2014). Most of the marine benthic coccoid dinoflagellates possess a similar type of life cycle, i.e. non-motile vegetative cells divide to form motile cells within a cell wall (thecate taxa) or sheath (athecate taxa) and the (usually motile) daughter cells are released, leaving behind the parental cell wall or sheath. The duration of the motile phase is often much shorter than that of the non-motile phase. After swimming, the motile cells become quiescent and return to the non-motile cell stage by developing the characteristic morphology (summarized in Hoppenrath et al. Citation2014). Some athecate taxa were described: e.g. Dinothrix rugata (Maiko Tamura & T. Horiguchi) Norico Yamada & T. Horiguchi (as Galeidinium Maiko Tamura & T. Horiguchi), Pyramidodinium T. Horiguchi & Sukigara and Spiniferodinium produce dome-shaped or pyramid-shaped cell coverings (Horiguchi & Chihara Citation1987; Horiguchi & Sukigara Citation2005; Tamura et al. Citation2005). Bindiferia species have a simple hyaline sheath around their dividing cells and the duration of the non-motile life cycle stage is not known. In culture, only very few of the cells are actively motile during the light phase.
Supplemental Material
Download PDF (1.4 MB)ACKNOWLEDGEMENTS
We thank David J. (Paddy) Patterson for the light micrographs of B. boggaya from Broome, Western Australia. DNA sequencing of Cawthron Institute Culture Collection of Micro-algae (CICCM) isolates of Togula jolla were prepared by Jacqui Stuart, Coastal and Freshwater Group, Cawthron Institute.
Disclosure statement
No potential conflict of interest was reported by the authors.
Supplementary material
Supplemental data for this article can be accessed on the publisher’s website.
Additional information
Funding
References
- Adachi M., Sako Y. & Ishida Y. 1994. Restriction fragment length polymorphism of ribosomal DNA internal transcribed spacer and 5.8s regions in Japanese Alexandrium species (Dinophyceae). Journal of Phycology 30: 857–863. DOI:https://doi.org/10.1111/j.0022-3646.1994.00857.x.
- Al-Yamani F.Y. & Saburova M.A. 2010. Illustrated guide on the flagellates of Kuwait’s intertidal soft sediments. Kuwait Institute for Scientific Research, Kuwait. 197 pp.
- Bachvaroff T.R., Gornik S.G., Concepcion G.T., Waller R.F., Mendez G.S., Lippmeier J.C. & Delwiche C.F. 2014. Dinoflagellate phylogeny revisited: using ribosomal proteins to resolve deep branching dinoflagellate clades. Molecular Phylogenetics and Evolution 70: 314–322. DOI:https://doi.org/10.1016/j.ympev.2013.10.007.
- Calado A.J. & Moestrup Ø. 2005. On the freshwater dinoflagellates presently included in the genus Amphidinium, with a description of Prosoaulax gen. nov. Phycologia 44: 112–119. DOI:https://doi.org/10.2216/0031-8884(2005)44[112:OTFDPI]2.0.CO;2.
- Chomérat N., Sellos D.Y., Zentz F. & Nézan E. 2010. Morphology and molecular phylogeny of Prorocentrum consutum sp. nov. (Dinophyceae), a new benthic dinoflagellate from south Brittany (northwestern France). Journal of Phycology 46: 183–194. DOI:https://doi.org/10.1111/j.1529-8817.2009.00774.x.
- Daugbjerg N., Hansen G., Larsen J. & Moestrup Ø. 2000. Phylogeny of some of the major genera of dinoflagellates based on ultrastructure and partial LSU rDNA sequence data, including the erection of three new genera of unarmoured dinoflagellates. Phycologia 39: 302–317. DOI:https://doi.org/10.2216/i0031-8884-39-4-302.1.
- Dragesco J. 1965. Étude cytologique de quelques flagellés mésopsammiques. Cahiers de Biologie Marine 6: 83–115.
- Edgar R.C. 2004. MUSCLE: multiple sequence alignment with high accuracy and high throughput. Nucleic Acids Research 32: 1792–1797. DOI:https://doi.org/10.1093/nar/gkh340.
- Flø Jørgensen M., Murray S. & Daugbjerg N. 2004a. Amphidinium revisited. I. Redefinition of Amphidinium (Dinophyceae) based on cladistic and molecular phylogenetic analyses. Journal of Phycology 40: 351–365. DOI:https://doi.org/10.1111/j.1529-8817.2004.03131.x.
- Flø Jørgensen M., Murray S. & Daugbjerg N. 2004b. A new genus of athecate interstitial dinoflagellates, Togula gen. nov., previously encompassed within Amphidinium sensu lato: inferred from light and electron microscopy and phylogenetic analyses of partial large subunit ribosomal DNA sequences. Phycological Research 52: 284–299. DOI:https://doi.org/10.1111/j.1440-1835.2004.tb00338.x.
- Flø Jørgensen M., Murray S. & Daugbjerg N. 2004c. Corrigendum to: Amphidinium revisited. I. Redefinition of Amphidinium (Dinophyceae) based on cladistic and molecular phylogenetic analyses. Journal of Phycology 40: 1181.
- Guillard R.R.L. & Ryther J.H. 1962. Studies on marine phytoplankton diatoms. I. Cyclotella nana Hustedt and Detonula confervacea (Cleve) Gran. Canadian Journal of Microbiology 8: 229–239. DOI:https://doi.org/10.1139/m62-029.
- Hansen G. & Daugbjerg N. 2004. Ultrastructure of Gyrodinium spirale, the type species of Gyrodinium (Dinophyceae), including a phylogeny of G. dominans, G. rubrum and G. spirale deduced from partial LSU rDNA sequences. Protist 155: 271–294. DOI:https://doi.org/10.1078/1434461041844231.
- Hansen G., Moestrup Ø. & Roberts K.R. 2000. Light and electron microscopical observations on the type species of Gymnodinium, G. fuscum (Dinophyceae). Phycologia 39: 365–376. DOI:https://doi.org/10.2216/i0031-8884-39-5-365.1.
- Herdman E.C. 1922. Notes on dinoflagellates and other organisms causing discolouration of the sand at Port Erin. II. Proceedings and Transactions of the Liverpool Biological Society 36: 15–30.
- Herdman E.C. 1924. Notes on dinoflagellates and other organisms causing discolouration of the sand at Port Erin. IV. Proceedings and Transactions of the Liverpool Biological Society 38: 75–84.
- Hoppenrath M. 2017. Dinoflagellate taxonomy – a review and proposal of a revised classification. Marine Biodiversity 47: 381–403. DOI:https://doi.org/10.1007/s12526-016-0471-8.
- Hoppenrath M. & Leander B.S. 2007a. Character evolution in polykrikoid dinoflagellates. Journal of Phycology 43: 366–377. DOI:https://doi.org/10.1111/j.1529-8817.2007.00319.x.
- Hoppenrath M. & Leander B.S. 2007b. Morphology and phylogeny of the pseudocolonial dinoflagellates Polykrikos lebourae and Polykrikos herdmanae n. sp. Protist 158: 209–227. DOI:https://doi.org/10.1016/j.protis.2006.12.001.
- Hoppenrath M., Bachvaroff T.R., Handy S.M., Delwiche C.F. & Leander B.S. 2009. Molecular phylogeny of ocelloid-bearing dinoflagellates (Warnowiaceae) as inferred from SSU and LSU rDNA sequences. BMC Evolutionary Biology 9: Article 116. DOI:https://doi.org/10.1186/1471-2148-9-116.
- Hoppenrath M., Murray S.A., Sparmann S.F. & Leander B.S. 2012. Morphology and molecular phylogeny of Ankistrodinium gen. nov. (Dinophyceae), a new genus of marine sand-dwelling dinoflagellates formerly classified within. Amphidinium. Journal of Phycology 48: 1143–1152. DOI:https://doi.org/10.1111/j.1529-8817.2012.01198.x.
- Hoppenrath M., Chomérat N. & Leander B.S. 2013. Molecular phylogeny of Sinophysis: evaluating the possible early evolutionary history of dinophysoid dinoflagellates. In: Biological and geological perspectives of dinoflagellates (Ed. by J.M. Lewis, F. Marret & L. Bradley), pp 207–214. Micropalaeontological Society special publications. Geological Society, London, UK.
- Hoppenrath M., Murray S.A., Chomérat N. & Horiguchi T. 2014. Marine benthic dinoflagellates – unveiling their worldwide biodiversity. Kleine Senckenberg-Reihe 54, Schweizerbart Science, Stuttgart, Germany. 276 pp.
- Horiguchi T. & Chihara M. 1987. Spiniferodinium galeiforme, a new genus and species of benthic dinoflagellates (Phytodiniales, Pyrrhophyta) from Japan. Phycologia 26: 478–487. DOI:https://doi.org/10.2216/i0031-8884-26-4-478.1.
- Horiguchi T. & Sukigara C. 2005. Pyramidodinium atrofuscum gen. et sp. nov. (Dinophyceae), a new marine sand-dwelling coccoid dinoflagellate from tropical waters. Phycological Research 53: 247–254. DOI:https://doi.org/10.1111/j.1440-183.2005.00392.x.
- Horiguchi T., Hayashi Y., Kudo H. & Hara Y. 2011. A new benthic dinoflagellate Spiniferodinium palauense sp. nov. (Dinophyceae) from Palau. Phycologia 50: 616–623. DOI:https://doi.org/10.2216/11-15.1.
- Horiguchi T., Tamura M., Katsumata K. & Yamaguchi A. 2012. Testudodinium gen. nov. (Dinophyceae), a new genus of sand-dwelling dinoflagellates formerly classified in the genus Amphidinium. Phycological Research 60: 137–149. DOI:https://doi.org/10.1111/j.1440-1835.2012.00645.x.
- Iwataki M., Hansen G., Moestrup Ø. & Matsuoka K. 2010. Ultrastructure of the harmful unarmored dinoflagellate Cochlodinium polykrikoides (Dinophyceae) with reference to the apical groove and flagellar apparatus. Journal of Eukaryotic Microbiology 57: 308–321. DOI:https://doi.org/10.1111/j.1550-7408.2010.00491.x.
- Janouškovec J., Gavelis G.S., Burki F., Dinh D., Bachvaroff T.R., Gornik S.G., Bright K.J., Imanian B., Strom S.L., Delwiche C.F. et al. 2017. Major transitions in dinoflagellate evolution unveiled by phylotranscriptomics. Proceedings of the National Academy of Sciences of the USA 114: E171–E180.
- John U., Fensome R.A. & Medlin L.K. 2003. The application of a molecular clock based on molecular sequences and the fossil record to explain biogeographic distributions within the Alexandrium tamarense “species complex” (Dinophyceae). Molecular Biology and Evolution 20: 1015–1027. DOI:https://doi.org/10.1093/molbev/msg105.
- Kalyaanamoorthy S., Minh B.Q., Wong T.K.F., von Haeseler A. & Jermiin L.S. 2017. ModelFinder: fast model selection for accurate phylogenetic estimates. Nature Methods 14: 587–589. DOI:https://doi.org/10.1038/nmeth.4285.
- Karafas S., Teng S.T., Leaw C.P. & Alves-de-Souza C. 2017. An evaluation of the genus Amphidinium (Dinophyceae) combining evidence from morphology, phylogenetics and toxin production, with the introduction of six novel species. Harmful Algae 68: 128–151. DOI:https://doi.org/10.1016/j.hal.2017.08.001.
- Kearse M., Moir R., Wilson A., Stones-Havas S., Cheung M., Sturrock S., Buxton S., Cooper A., Markowitz S., Duran C. et al. 2012. Geneious basic: an integrated and extendable desktop software platform for the organization and analysis of sequence data. Bioinformatics 28: 1647–1649. DOI:https://doi.org/10.1093/bioinformatics/bts199.
- Klebs G. 1884. Ein kleiner Beitrag zur Kenntniss der Peridineen. Botanische Zeitung 42: 737–745, pl. X.
- Kofoid C.A. & Swezy O. 1921. The free-living unarmored Dinoflagellata. Memoirs of the University of California 5: 562pp.
- Kohli G.S., Neilan B.A., Brown M.V., Hoppenrath M. & Murray S.A. 2014. Cob gene pyrosequencing enables characterization of benthic dinoflagellate diversity and biogeography. Environmental Microbiology 16: 467–485. DOI:https://doi.org/10.1111/1462-2920.12275.
- Kretzschmar A.L., Verma A., Murray S., Kahlke T., Fourment M. & Darling A. 2019. Trial by phylogenetics – evaluating the multi-species coalescent for phylogenetic inference on taxa with high levels of paralogy (Gonyaulacales, Dinophyceae). BioRXiv. DOI: https://doi.org/10.1101/683383.
- Larsen J. 1985. Algal studies of the Danish Wadden Sea II. A taxonomic study of psammobious dinoflagellates. Opera Botanica 79: 14–37.
- Larsen J. & Patterson D.J. 1990. Some flagellates (Protista) from tropical marine sediments. Journal of Natural History 24: 801–937. DOI:https://doi.org/10.1080/00222939000770571.
- Medlin L., Elwood H.J., Stickel S. & Sogin M.L. 1988. The characterization of enzymatically amplified eukaryotic 16S-like rRNA-coding regions. Gene 71: 491–499. DOI:https://doi.org/10.1016/0378-1119(88)90066-2.
- Moestrup Ø. & Daugbjerg N. 2007. On dinoflagellate phylogeny and classification. In: Unravelling the algae – the past, present, and future of algal systematics (Ed. by J. Brodie & J. Lewis), pp 215–230. Systematics Association special volume series 75. CRC Press, Boca Raton, Florida, USA.
- Murray S.A. 2003. Diversity and phylogenetics of sand-dwelling dinoflagellates from Southern Australia. PhD Thesis. University of Sydney, Sydney, Australia. 202 pp.
- Murray S.A. & Patterson D.J. 2002. The benthic dinoflagellate genus Amphidinium in south-eastern Australian waters, including three new species. European Journal of Phycology 37: 279–298. DOI:https://doi.org/10.1017/S0967026202003591.
- Murray S.A., Flø Jørgensen M., Daugbjerg N. & Rhodes L. 2004. Amphidinium revisited. II. Resolving species boundaries in the Amphidinium operculatum species complex (Dinophyceae), including the description of Amphidinium trulla sp. nov. and Amphidinium gibbosum comb. nov. Journal of Phycology 40: 366–382. DOI:https://doi.org/10.1046/j.1529-8817.2004.03132.x.
- Murray S.A., Flø Jørgensen M., Ho S.Y., Patterson D.J. & Jermiin L.S. 2005. Improving the analysis of dinoflagellate phylogeny based on rDNA. Protist 156: 269–286. DOI:https://doi.org/10.1016/j.protis.2005.05.003.
- Murray S., Hoppenrath M., Larsen J. & Patterson D.J. 2006a. Bysmatrum teres sp. nov., a new sand-dwelling dinoflagellate from north-western Australia. Phycologia 45: 161–167. DOI:https://doi.org/10.2216/05-05.1.
- Murray S., Hoppenrath M., Preisfeld A., Larsen J., Yoshimatsu S., Toriumi S. & Patterson D.J. 2006b. Phylogenetics of Rhinodinium broomeense gen. et sp. nov., a peridinioid, sand-dwelling dinoflagellate (Dinophyceae). Journal of Phycology 42: 934–942. DOI:https://doi.org/10.1111/j.1529-8817.2006.00241.x.
- Murray S.A., Diwan R., Orr R.J., Kohli G.S. & John U. 2015. Gene duplication, loss and selection in the evolution of saxitoxin biosynthesis in alveolates. Molecular Phylogenetics and Evolution 92: 165–180. DOI:https://doi.org/10.1016/j.ympev.2015.06.017.
- Nguyen L.-T., Schmidt H.O., von Haeseler A. & Minh B.Q. 2015. IQ-TREE: a fast and effective stochastic algorithm for estimating maximum likelihood phylogenies. Molecular Biology and Evolution 32: 268–274. DOI:https://doi.org/10.1093/molbev/msu300.
- Nunn G.B., Theisen B.F., Christensen B. & Arctander P. 1996. Simplicity-correlated size growth of the nuclear 28S ribosomal RNA D3 expansion segment in the crustacean order Isopoda. Journal of Molecular Evolution 42: 211–223. DOI:https://doi.org/10.1007/BF02198847.
- Onuma R., Watanabe K. & Horiguchi T. 2015. Pellucidodinium psammophilum gen. & sp. nov. and Nusuttodinium desymbiontum sp. nov. (Dinophyceae), two novel heterotrophs closely related to kleptochloroplastidic dinoflagellates. Phycologia 54: 192–209. DOI:https://doi.org/10.2216/14-103.1.
- Orr R.J.S., Murray S.A., Stüken A., Rhodes L. & Jakobsen K.S. 2012. When naked became armored: an eight-gene phylogeny reveals monophyletic origin of theca in dinoflagellates. PLOS One 7: Article e50004. DOI:https://doi.org/10.1371/journal.pone.0050004.
- Price D.C. & Bhattacharya D. 2017. Robust dinoflagellate phylogeny inferred from public transcriptome databases. Journal of Phycology 53: 725–729. DOI:https://doi.org/10.1111/jpy.12529.
- Rhodes L., Smith K., Selwood A., McNabb P., Van Ginkel R., Holland P. & Munday R. 2010. Production of the cyclic imine, pinnatoxin, by a peridinoid dinoflagellate isolated from Northland, New Zealand. Harmful Algae 9: 384–389. DOI:https://doi.org/10.1016/j.hal.2010.01.008.
- Ronquist F. & Huelsenbeck J.P. 2003. MrBayes 3: bayesian phylogenetic inference under mixed models. Bioinformatics 19: 1572–1574. DOI:https://doi.org/10.1093/bioinformatics/btg180.
- Scholin C.A., Herzog M., Sogin M. & Anderson D.M. 1994. Identification of group-and strain-specific genetic markers for globally distributed Alexandrium (Dinophyceae). II. Sequence analysis of a fragment of the LSU rRNA gene. Journal of Phycology 30: 999–1011. DOI:https://doi.org/10.1111/j.0022-3646.1994.00999.x.
- Sparmann S.F., Leander B.S. & Hoppenrath M. 2008. Comparative morphology and molecular phylogeny of taxa of the new marine benthic dinoflagellate genus Apicoporus, classified formerly within Amphidinium sensu lato. Protist 159: 383–399. DOI:https://doi.org/10.1016/j.protis.2007.12.002.
- Takano Y. & Horiguchi T. 2004. Surface ultrastructure and molecular phylogenetics of four unarmored heterotrophic dinoflagellates, including the type species of the genus Gyrodinium (Dinophyceae). Phycological Research 52: 107–116. DOI:https://doi.org/10.1111/j.1440-1835.2004.tb00319.x.
- Takano Y., Yamaguchi H., Inouye I., Moestrup Ø. & Horiguchi T. 2014. Phylogeny of five species of Nusuttodinium gen. nov. (Dinophyceae), a genus of unarmoured kleptoplastidic dinoflagellates. Protist 165: 759–778. DOI:https://doi.org/10.1016/j.protis.2014.09.001.
- Tamura M., Shimada S. & Horiguchi T. 2005. Galeidinium rugatum gen. et sp. nov. (Dinophyceae), a new coccoid dinoflagellate with a diatom endosymbiont. Journal of Phycology 41: 658–671. DOI:https://doi.org/10.1111/j.1529-8817.2005.00085.x.
- Tamura M., Takano Y. & Horiguchi T. 2009. Discovery of a novel type of body scale in the marine dinoflagellate, Amphidinium cupulatisquama sp. nov. (Dinophyceae). Phycological Research 57: 304–312. DOI:https://doi.org/10.1111/j.1440-1835.2009.00550.x.
- Thompson J.D., Higgins D.G. & Gibson T.J. 1994. CLUSTAL W: improving the sensitivity of progressive multiple sequence alignment through sequence weighting, position-specific gap penalties and weight matrix choice. Nucleic Acids Research 22: 4673–4680. DOI:https://doi.org/10.1093/nar/22.22.4673.
- Turland N.J., Wiersema J.H., Barrie F.R., Greuter W., Hawksworth D.L., Herendeen P.S., Knapp S., Kusber W.-H., Li D.-Z., Marhold K. et al. [Eds]. 2018. International code of nomenclature for algae, fungi, and plants (Shenzhen Code) adopted by the nineteenth international botanical congress Shenzhen, China, July 2017. Koeltz Botanical Books, Glashütten, Germany. xxxviii + 254 pp. Regnum Vegetabile 159. DOI:https://doi.org/10.12705/Code.2018.
- Uhlig G. 1964. Eine einfache Methode zur Extraktion der vagilen, mesopsammalen Microfauna. Helgoländer wissenschaftliche Meeresuntersuchungen 11: 178–185. DOI:https://doi.org/10.1007/BF01612370.
- Verma A., Kazandjian A., Sarowar C., Harwood D.T., Murray J.S., Pargmann I., Hoppenrath M. & Murray S.A. 2019. Morphology and phylogenetics of benthic Prorocentrum species (Dinophyceae) from tropical north-western Australia. Toxins 11: Article 571. DOI:https://doi.org/10.3390/toxins11100571.
- Yamada N., Terada R., Tanaka A. & Horiguchi T. 2013. Bispinodinium angelaceum gen. et sp. nov. (Dinophyceae), a new sand-dwelling dinoflagellate from the seafloor off Mageshima Island, Japan. Journal of Phycology 49: 555–569. DOI:https://doi.org/10.1111/jpy.12064.
- Žerdoner Čalasan A., Kretschmann J. & Gottschling M. 2019. They are young, and they are many: dating freshwater lineages in unicellular dinophytes. Environmental Microbiology 21: 4125–4135. DOI:https://doi.org/10.1111/1462-2920.14766.