Abstract
Testosterone deficiency in males is associated with serious comorbidities such as cardiovascular disease, diabetes type two, and also an increased risk of premature death. The pathogenetic mechanism behind this association, however, has not yet been clarified and is potentially bidirectional. The aim of this clinical trial was to gain insight into the short-term effect of changes in testosterone on blood analytes in healthy young men. Thirty healthy young male volunteers were recruited and monitored in our designed human model. Blood sampling was performed prior to and 3 weeks after pharmacological castration with a gonadotropin-releasing hormone antagonist. Subsequently, testosterone replacement with 1000 mg testosterone undecanoate was given and additional blood samples were collected 2 weeks later. The alterations in the levels of 37 routine biomarkers were statistically analysed. Eight biomarkers changed significantly in a similar manner as testosterone between the time points (e.g. prostate specific antigen, creatinine and magnesium), whereas seven other markers changed in the inverse manner as testosterone, including sexual hormone-binding globulin, urea, aspartate aminotransferase and alanine aminotransferase. Most of our results were supported by data from other studies. The designed controlled human model yielded changes in known biomarkers suggesting that low testosterone has a negative effect on health in young healthy men.
Introduction
The association between testosterone levels and male health has been the subject of several recent studies. Serum testosterone levels gradually decline as men age and when this decline is accelerated it is referred to as late-onset hypogonadism, i.e. a condition characterized by low testosterone and clinical symptoms such as loss of muscle mass, loss of libido, azoospermia and a reduced ability to concentrate [Citation1]. Hypogonadism is now recognized as a common condition with an estimated prevalence of up to 30% in males from 46 to 89 years old [Citation2]. It is difficult, however, to determine the prevalence, and this is partly due to the lack of a definition of the lowest normal concentration [Citation3]. Concurrent with an age-related decline in testosterone, there is an increase in sexual hormone-binding globulin (SHBG). Subsequently, increasing SHBG contributes to further lower levels of free testosterone. Additionally, the concentration of testosterone has been reported to be the subject of negative secular trend [Citation1,Citation3–5].
It is important to diagnose hypogonadism due to the association with an increased risk of serious diseases, e.g. cardiovascular disease (CVD) [Citation6], metabolic syndrome and type 2 diabetes, that can result in premature mortality [Citation1,Citation3,Citation7,Citation8]. However, the causality of association between low testosterone and these comorbidities is not yet clarified and may indeed be bidirectional [Citation9,Citation10]. Testosterone also plays an important role in preventing osteoporosis, which is probably due to its conversion to estradiol [Citation11].
The reports on a potential association between low testosterone and decreased male health have stimulated an interest in testosterone replacement therapy (TRT). Sales of synthetic testosterone have greatly increased; although opinions differ regarding the efficacy and safety of TRT [Citation6,Citation10,Citation12–14]. Knowledge on both the short-term and long-term effects of pathological testosterone levels is limited.
The aim of the present study was to gain insight into the effects of changes in testosterone by analyzing routine blood biomarkers in healthy young men undergoing pharmaceutical castration and subsequent testosterone supplementation. Blood samples were analyzed at three different states in each individual: normal testosterone, low testosterone and after testosterone replacement.
Materials and methods
Subjects
The subjects were recruited through advertisements in the local media and Lund University Medical School and all received reimbursement for participation. The study was approved and registered by the Regional Ethical Review Board Lund (http://epn.se/en, Approval number: DNR 2014/311, date of approval 8 May 2014). Additionally, the participants provided signed informed consent. Inclusion criteria were the following: 19–32 years of age, healthy, body mass index (BMI) 20–25 kg/m2, non-smoker or occasional smoker. Exclusion criteria included: regular medication, exposure to anabolic steroids, drugs of abuse used within the last year or ever presenting with a stroke, heart and liver disease, cancer or any other chronic disease. This information was obtained through a questionnaire. Thirty healthy males were included in the study performed at the Centre for Reproductive Medicine at Skåne University Hospital in Malmö, Sweden.
Study outline
At each time point, the subjects were interviewed about their current health status. Any symptoms that may be related to the medication given were noted. There were 29 non-smokers and one occasional smoker (n = 30). The subject characteristics (mean) were as follows: age = 23.9 years, height = 181.9 cm, weight = 75.8 kg, BMI = 23.0 kg/m2 and number of alcohol units per week = 1.2. The ranges of these parameters are provided in .
Table 1. Questionnaire responses from the subjects in the study detailing age, height, weight, BMI and alcohol habits (n = 30).
A flow diagram of the subjects can be viewed in . Blood samples were collected at three time points. At baseline, after the first blood collection, each subject received a subcutaneous abdominal injection of gonadotropin-releasing hormone antagonist (GnRHa) (Degeralix®, Ferring Pharmaceuticals, 240 mg). After 3 weeks, following the second blood collection, an intramuscular injection of testosterone undecanoate (Nebido®, Bayer Pharmaceuticals, 1000 mg) was administered; and after a further 2 weeks, the participants returned for the final blood collection. Thus, blood was collected from the same 30 healthy men at the following time points: (A) normal testosterone and gonadotropins; (B) low testosterone and low gonadotropins and (C) supplemented testosterone and persistent low gonadotropins.
Figure 1. Subject flow of the designed human model. Thirty blood samples were collected at (A) baseline, (B) 3 weeks after the GnRH antagonist (Degeralix) had full effect and (C) 2 weeks after receiving testosterone undecanoate (Nebido®). The results in biomarkers followed were then subjected to paired statistical analysis, and the results were biologically interpreted.
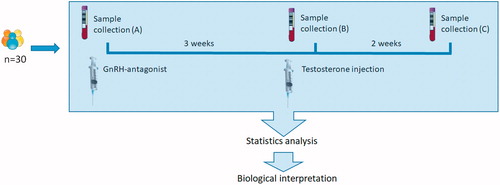
Sample handling and measurements
At each time point, four tubes of blood (EDTA–blood, sodium heparin plasma, fluoride citrate plasma and sodium citrate plasma) were sent to the Clinical Chemistry Laboratory at Skåne University Hospital in Malmö for hematological analysis and assessment of clinical chemistry biomarkers. The blood samples were collected under fasting conditions between 8 a.m. and 10 a.m. The following biomarkers were analyzed: follicular stimulating hormone (FSH), luteinizing hormone (LH), estradiol, testosterone, SHBG, testosterone/SHBG, thyroid stimulating hormone (TSH), insulin, albumin, aspartate aminotransferase (ASAT), alanine aminotransferase (ALAT), glutamyltransferase, alkaline phosphatase, C-reactive protein (CRP), creatine kinase, prostate specific antigen (PSA), troponin T, sodium, potassium, calcium, urea, magnesium, glucose, creatinine, apolipoprotein A1 (apoA1), apolipoprotein B (apoB), apoB/apoA1, cholesterol, triglycerides, hemoglobin, leukocytes, thrombocytes, neutrophils, eosinophils, basophils, lymphocytes and monocytes. The biomarkers were measured by automated analytical devices (hematology: Sysmex XN-2000, Norderstedt, Germany; clinical chemistry: Cobas 6000, Roche Diagnostics, Mannheim, Germany). Range of measurements, lower limit of detection, coefficients of variation and units are provided in Table S1. The data from study can be viewed in Table S2.
Statistics
The statistical analyses were performed using SPSS Statistics 21.0 (IBM, Somers, IL, USA) and R programs [Citation15]. A descriptive analysis was performed in order to optimize further data handling. The presence of outliers was checked by performing principal component analysis and boxplots. The normal distribution of the variables was checked at each time point by the Shapiro–Wilk test [Citation16]. The data was handled differently depending on the distribution within the time points. The changes between time points for each biomarker were analyzed separately.
The biomarkers that followed a normal distribution were analyzed by the paired Student’s t-test (2-tailed). Other parameters were accordingly analyzed by Wilcoxon and McNemar tests. The significance level was adjusted as 0.05/3 (number of comparisons) to control Type I errors from multiple comparisons (Bonferroni correction) [Citation17]. Biomarkers with p-value <.0167 from were considered statistically significant. As a complementary analysis, the 95% confidence intervals (CIs) [Citation18] of the mean of the differences between time points were analyzed to corroborate previous analyses. If the CI did not include zero, the change was considered to be statistically significant.
Results
Outcome of the human model
Our study design provided a unique set of samples for the following conditions: normal testosterone, low testosterone and supplemented testosterone. The effect of the temporary chemical castration was clearly apparent after a radical reduction in the concentration of the reproductive hormones in condition B. Meanwhile, following testosterone supplementation, the concentrations of testosterone and the ratio of testosterone and SHBG generally increased to higher levels compared to baseline ().
Figure 2. Changes in reproductive hormones due to pharmacological interventions in the individual subject prior to normalization. Subject 1–29 at the three time points: (A) baseline, (B) GnRH antagonist and (C) GnRH antagonist with synthetic testosterone. The subjects are represented by different colors. At time point (C), LH and FSH remain low due to the inhibitory effect of testosterone on the hormonal axis. Due to the administration of synthetic testosterone, testosterone is high in time point (C).
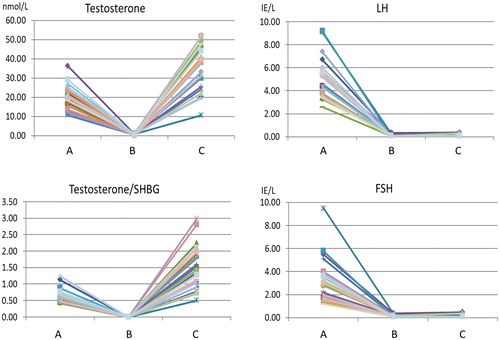
The majority of the subjects (28/30) recovered the level of testosterone or had a higher level of the hormone compared to baseline. Two of the subjects still had sub-baseline testosterone levels at the last time point. In addition, the variation in testosterone and gonadotropins was greatest in the last time point and at baseline, respectively (). One subject was removed from further analysis because he was a statistical outlier at baseline in several biomarkers, however, he is included in the reporting of side effects (Figure S1).
Reported side effects
Twenty-two subjects reported side effects after injection of GnRHa. The most common complaint was decreased libido (n = 15). Other common complaints included: tiredness (n = 7), depressive symptoms (n = 6) and irritation around the injection site (n = 5). In total, eight subjects experienced hot flashes after the testosterone injection. Six subjects did not have any side effects during the study, and seven of the participants experienced side effects after both treatments.
No unexpected side effects were reported. The side effects reported by the subjects are common and were not harmful. The study group was relatively homogeneous, and all subjects responded similarly to the GnRHa treatment. There were, however, individual differences in response to the testosterone undecanoate injection.
Biomarkers with significant difference between time points
In , biomarkers that exhibited significant change between all three time point comparisons include the following: testosterone, testosterone/SHBG, estradiol and PSA. Markers that were significant between the comparisons A–B and B–C were ALAT, apolipoprotein A1 and calcium. LH and FSH had a significant change in the comparisons A–B and A–C, due to the means being close to zero at both time point B and C.
Table 2. Results from statistical tests.Table Footnote*
Additionally, the markers that either exhibited a positive or negative relation to testosterone and had at least one significant change in the time point comparisons are included in , where the average concentration in each time point can be viewed. The biomarkers in green are following the same pattern as testosterone, the red biomarkers behave in the opposite manner.
Figure 3. Association patterns of the biomarkers exhibiting statistically significant variations. Biomarkers with a similar pattern to testosterone (two left columns). Biomarkers with an inverse pattern to testosterone (two right columns). On the x axes are the three time points: (A) baseline, (B) GnRH antagonist and (C) GnRH antagonist with synthetic testosterone. Horizontal lines with an asterisk (*) represent significant changes (p-value < .0167) between time points.
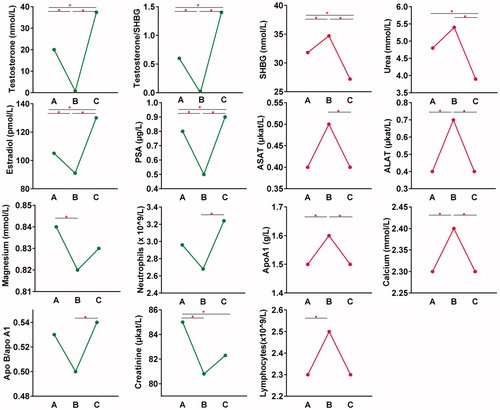
Discussion
Influence of testosterone on the biomarkers
We have designed a human model for the study of the biochemical effects of hypogonadism in males. The model yielded significant changes in several routine blood biomarkers due to changes in testosterone levels. As the subjects were healthy and the short-term treatment did not jeopardize overall health, the changes in the disease biomarkers were not expected to be large. The fold change between the means is therefore not very high, although it was enough to produce significant changes for the majority of the biomarkers monitored.
Additionally, most of the biomarkers that had significant changes in the time point comparison A to B, recovered during comparison of B to C. Such biomarkers seem related to the change in testosterone levels because of the subsequent restoration following reintroduction of testosterone. Creatinine, magnesium and lymphocytes did not achieve a significant recovery, but these showed a tendency to recover at the last time point.
The changes observed in magnesium and creatinine are supported by other studies, where the results demonstrated that these biomarkers are regulated by testosterone [Citation19]. The observation that PSA increased with testosterone is in accordance with another study investigating the relation between testosterone and PSA in healthy men [Citation20]. The increase, however, is extremely low and does not suggest a pathological development in the subjects in the present study.
Under hypogonadism, the apoB/apoA1 ratio decreased but returned to baseline levels after testosterone replacement. An increase in apoB/apoA1 is known to be related to an increased risk of coronary heart disease [Citation21]. These results appear to conflict with the theory that hypogonadism is associated with an increased risk of CVD. This could possibly be due to the fact that the subjects were healthy young males; and as such, could physiologically compensate for the lipid metabolism despite exhibiting a low testosterone level.
The changes observed in urea and calcium levels were seen in a previous study that also demonstrated that these were related to changes in testosterone [Citation19]. The alteration in urea and creatinine suggested muscle degradation in the hypogonadal state. Catabolism of amino acids can be used for gluconeogenesis or are cleared from the body via the kidney in the urea cycle. Additionally, decreased muscle mass is one of the symptoms of hypogonadism [Citation1].
The change in ALAT and ASAT in relation to testosterone is in agreement with other studies. Testosterone is important for liver function and low testosterone has been associated with non-alcoholic fatty liver disease, probably due to the effects on lipid metabolism [Citation22]. A recent investigation in young adults revealed that ALAT and ASAT/ALAT levels were significantly correlated with insulin resistance, where ALAT was found to be significantly elevated in subjects with insulin resistance [Citation23].
General discussion and concluding remarks
Due to a lack of data on the short-term response to GnRHa treatment and subsequent restoration of testosterone levels by hormone replacement, it is difficult to directly compare our results with other studies. In the present study, the effect of testosterone could be delineated and most of the biomarkers changed significantly between the time points. The fact that all biomarkers do not change according to comorbidities may be because the subjects were healthy and thus could physiologically compensate for low testosterone levels. Another explanation could be that some of the comorbid changes are due to the disease inducing low testosterone.
A study from 2001 compared biomarker levels in young healthy men following different doses of testosterone with weekly injections of testosterone over a 20-week period [Citation24], as well as monthly injections of GnRH agonists. The subjects that were given the highest dose of synthetic testosterone (600 mg/week) had a significant increase in PSA. In our investigation, PSA also increased after one dose of 1000 mg testosterone undecanoate. ALAT and ALP did not significantly differ across the time points; however, in the present study a change was apparent. The power of our model is thus illustrated, as a larger difference in testosterone was created.
Three studies investigating the short-term effects on insulin sensitivity due to testosterone fluctuations in healthy males [Citation25–27]. Neither study found significant effects in total glucose disposal or insulin sensitivity. One of the studies found that adipose mass was inversely correlated testosterone, estradiol and dihydrotestosterone. Another of the studies revealed that total glucose disposal occurred in early induced hypogonadism and suggested that this could be due to a compensating mechanism by adiponectin. This could explain why the glucose levels did not significantly change in the present study. It is well known that testosterone has a positive effect on insulin. Men suffering from low testosterone have a greater risk of developing diabetes type two and TRT has been shown to increase insulin sensitivity [Citation28].
Based on three different studies on the long term effects of TRT, a review by Traish [Citation29] found that CRP decreased with TRT in men suffering from testosterone deficiency. A significant decrease in cholesterol and triglycerides after synthetic testosterone was apparent. The first measurement after baseline was at 12 months; while in our study there was only 5 weeks from baseline to the last time point. The subjects in the studies that Traish evaluated had a mean age of 57–61 years. In our study, significant differences in cholesterol and triglycerides may have become apparent if the time line had been extended, however, as our subjects were healthy young males it is difficult to say with certainty.
In conclusion, short-term hypogonadism induced by pharmaceutical castration with GnRHa in healthy young men and subsequent testosterone replacement produced changes in blood biomarkers that change positively and negatively with testosterone. In order to observe biomarkers indicative negative health effects, a controlled human model was designed that avoided changes of high magnitude. Many of the biomarkers related to conditions such as CVD, diabetes type two and liver disease displayed expected patterns to indicate that low testosterone does have a negative effect on health. Patients with age-related hypogonadism commonly suffer from these conditions. The effects described in our study suggested that short-term changes in plasma biomarkers can occur in patients that undergo pharmaceutical castration. This could be beneficial information for caregivers treating patients undergoing such therapy.
Disclosure statement
No potential conflict of interest was reported by the authors.
Additional information
Funding
References
- Traish AM, Miner MM, Morgentaler A, et al. Testosterone deficiency. Am J Med. 2011;124(7):578–587.
- Allan CA, McLachlan RI. Age-related changes in testosterone and the role of replacement therapy in older men. Clin Endocrinol (Oxf). 2004;60(6):653–670.
- Miner M, Barkin J, Rosenberg MT. Testosterone deficiency: myth, facts, and controversy. Can J Urol. 2014;21:39–54.
- Araujo AB, Wittert GA. Endocrinology of the aging male. Best Pract Res Clin Endocrinol Metab. 2011;25(2):303–319.
- Travison TG, Araujo AB, O’Donnell AB, et al. A population-level decline in serum testosterone levels in American men. J Clin Endocrinol Metab. 2007;92(1):196–202.
- Kloner RA, Carson C, Dobs A, et al. Testosterone and cardiovascular disease. J Am Coll Cardiol. 2016;67(5):545–557.
- Ebrahimi F, Christ-Crain M. Metabolic syndrome and hypogonadism-two peas in a pod. Swiss Med Wkly. 2016;146:w14283.
- Cheung KKT, Luk AOY, So WY, et al. Testosterone level in men with type 2 diabetes mellitus and related metabolic effects: a review of current evidence. J Diabetes Invest. 2015;6(2):112–123.
- Zarotsky V, Huang MY, Carman W, et al. Systematic literature review of the risk factors, comorbidities, and consequences of hypogonadism in men. Andrology. 2014;2(6):819–834.
- Bhasin S. A perspective on the evolving landscape in male reproductive medicine. J Clin Endocrinol Metab. 2016;101(3):827–836.
- Mohamad NV, Soelaiman IN, Chin KY. A concise review of testosterone and bone health. Clin Interv Aging. 2016;11:1317–1324.
- Gencer B, Mach F. Testosterone: a hormone preventing cardiovascular disease or a therapy increasing cardiovascular events? Eur Heart J. 2016;37(48):3569–3575.
- Morgentaler A. Controversies and advances with testosterone therapy: a 40-year perspective. Urology. 2016;89:27–32.
- Naderi S. Testosterone replacement therapy and the cardiovascular system. Curr Atheroscler Rep. 2016;18:1–6.
- R Core Team. R: A language and environment for statistical computing. R Foundation for Statistical Computing [Internet]; 2016. Available from: https://www.r-project.org/
- Shapiro SS, Wilk A. An analysis of variance test for normality (complete samples). Biometrika. 1965;52(3-4):591.
- Bonferroni CE. Teoria statistica delle classi e calcolo delle probabilità. 8th ed. Pubblicazioni Del R Istituto Superiore di Scienze Economiche e Commerciali di Firenze. Libreria internazionale Seeber; 1936. p. 3–62.
- Greenfield ML, Kuhn JE, Wojtys EM. A statistics primer. Confidence intervals. Am J Sports Med. 1998;26(1):145–149.
- Lam T, Poljak A, McLean M, et al. Testosterone prevents protein loss via the hepatic urea cycle in human. Eur J Endocrinol. 2017;176(4):489–496.
- Peskoe SB, Joshu CE, Rohrmann S, et al. Circulating total testosterone and PSA concentrations in a nationally representative sample of men without a diagnosis of prostate cancer. Prostate. 2015;75(11):1167.
- Lu M, Lu Q, Zhang Y, et al. ApoB/apoA1 is an effective predictor of coronary heart disease risk in overweight and obesity. J Biomed Res. 2011;25(4):266–273.
- Mody A, White D, Kanwal F, et al. Relevance of low testosterone to nonalcoholic fatty liver disease. Cardiovasc Endocrinol. 2015;4(3):83–89.
- Simental-Mendía LE, Rodríguez-Morán M, Gómez-Díaz R, et al. Insulin resistance is associated with elevated transaminases and low aspartate aminotransferase/alanine aminotransferase ratio in young adults with normal weight. Eur J Gastroenterol Hepatol. 2017;29(4):435–440.
- Bhasin S, Woodhouse L, Casaburi R, et al. Testosterone dose-response relationships in healthy young men. Am J Physiol Metab. 2001;281:E1172–E1181.
- Rabiee A, Dwyer AA, Caronia LM, et al. Impact of acute biochemical castration on insulin sensitivity in healthy adult men. Endocr Res. 2010;35(2):71–84.
- Host C, Gormsen LC, Hougaard DM, et al. Acute and short-term chronic testosterone fluctuation effects on glucose homeostasis, insulin sensitivity, and adiponectin: a randomized, double-blind, placebo-controlled, crossover study. J Clin Endocrinol Metab. 2014;99:1088–1096.
- Thirumalai A, Rubinow KB, Cooper LA, et al. Dose-response effects of sex hormone concentrations on body composition and adipokines in medically castrated healthy men administered graded doses of testosterone gel. Clin Endocrinol. 2017;87(1):59–67.
- Kelly DM, Jones TH. Testosterone: a metabolic hormone in health and disease. J Endocrinol. 2013;217(3):R25–R45.
- Traish AM. Outcomes of testosterone therapy in men with testosterone deficiency (TD): part II. Steroids. 2014;88:117–126.