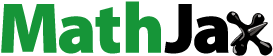
Abstract
Glucose measurement plays a central role in the diagnosis of gestational diabetes mellitus (GDM). Because of earlier reports of overestimation of glucose in the widely used tubes containing granulated glycolysis inhibitor, the study assessed the performance of fast-clotting serum tubes as an alternative sample for the measurement of glucose. Glucose concentration in fast-clotting serum was compared to lithium-heparin plasma placed in an ice-water slurry after sample collection and glucose stability at room-temperature was studied. Blood samples from 30 volunteers were drawn in four different types of tubes (serum separator tubes, fast-clotting serum tubes, lithium-heparin tubes and sodium fluoride, EDTA and a citrate buffer (NaF–EDTA–citrate) tubes, all from Greiner Bio-One). Lithium-heparin tubes were placed in an ice-water slurry until centrifugation in accordance with international recommendations and centrifuged within 10 min. After centrifugation, glucose was measured in all tubes (timepoint T0) and after 24, 48, 72, 96 and 120 h of storage at 20–22 °C. NaF–EDTA–citrate plasma showed significant overestimation of glucose concentration by 4.7% compared to lithium-heparin plasma; fast-clotting serum showed glucose concentrations clinically equivalent to lithium-heparin plasma. In fast-clotting serum tubes, mean bias between glucose concentration after 24, 48, 72, 96 and 120 h and T0 was less than 2.4%. All individual differences compared to T0 were less than 6.5%. The results fulfill the acceptance criteria for sample stability based on biological variation. Fast-clotting serum tubes can be an alternative for the measurement of glucose in diagnosis and management of GDM and diabetes mellitus, especially when prolonged transportation is necessary.
Introduction
Measurement of fasting plasma glucose and glucose after a oral glucose tolerance test (oGTT) plays a central role in the diagnosis of gestational diabetes mellitus (GDM) and diabetes mellitus (DM). While diagnosis of DM primary is based on measuring hemoglobin A1c, the diagnostic criteria for the diagnosis of GDM are based solely on plasma glucose concentration [Citation1]. Therefore, glucose should be measured in venous plasma when used for diagnosis according to the recommendations of the National Academy of Clinical Biochemistry (NACB) [Citation2]. For reliable identification of diabetes patients, exact measurement of plasma glucose is essential.
The preanalytical phase for glucose measurement is critical because of ongoing metabolism of glucose after sample collection. Without a stabilizer, glucose decreases by 5–7% per hour [Citation3]. To stop glycolysis, plasma must be separated from blood cells by centrifugation as soon as possible. Since delay of sample transport and centrifugation are regularly encountered, alternative procedures to minimize glycolysis are recommended. The NACB therefore recommends to use tubes containing a rapidly effective glycolysis inhibitor [Citation2]. The former widely used tubes containing sodium fluoride alone as a glycolysis inhibitor are considered insufficient by the NACB and World Health Organization (WHO) [Citation2,Citation4]. Instead, tubes containing a mixture of sodium fluoride, EDTA and a citrate buffer (NaF–EDTA–citrate tubes) should be used [Citation2]. Since, several national societies have implemented the use of NaF–EDTA–citrate tubes in their national guidelines for the diagnosis of GDM.
NaF–EDTA–citrate tubes are provided with liquid additives (e.g. GlucoExact, Sarstedt, Nümbrecht, Germany), or with granulated additives (e.g. Vacuette FC-mix, Greiner Bio-One GmbH, Frickenhausen, Germany). The use of glycolysis inhibitor in granular form has a pre-analytical advantage by eliminating dilution effects, which could occur due to insufficient tube filling when using liquid additives. NaF–EDTA–citrate tubes have been reported to overestimate glucose concentration in plasma [Citation5,Citation6]. Systematic higher plasma glucose measurement can lead to misclassification of diabetes patients and erroneous patient management when using the current established decision limits for diagnosis of diabetes [Citation7,Citation8]. Some authors therefore discourage use of NaF–EDTA–citrate tubes for routine use until the decision limits for diagnosis of diabetes have been re-evaluated [Citation7]. However, recent outcome-studies demonstrated lower frequencies of preterm and primary cesarean delivery when using citrate-buffered tubes for the measurement of glucose in diagnosis of GDM compared to NaF-tubes without glycolysis inhibitor [Citation9].
If tubes containing a glycolysis inhibitor cannot be used, current guidelines recommend to place sample tubes immediately in an ice-water slurry and to separate the plasma from the cells within 15–30 min [Citation2]. In daily practice, this is a challenge and extremely rarely used for obvious reasons. Further, some authors stated that placing the tube in an ice-water slurry does not stop glycolysis promptly and completely [Citation10,Citation11].
In Norway, most samples for diagnosis of GDM and DM are taken in primary health care. Shipping of the samples to the central laboratory can take up to several days and glucose stability is crucial in these cases. When using tubes containing dry citrate and fluoride additives, complete dissolving of granula is crucial to achieve glucose stability. Difficulties in dissolving fluoride and citrate granula in these tubes have been reported, even if the recommended number of tube inversions was followed [Citation12]. Not fully dissolving of granula results in reduced stability of glucose [Citation12]. Manufactures confirm glucose stability up to 24 h at room-temperature without centrifugation and up to 48 h after centrifugation. In countries where long transportation times are expected, this may not be sufficient. Two studies confirmed long-term stability of glucose in tubes containing granulated NaF–EDTA–citrate for up to five days at room-temperature, but one of the investigated tubes (Venosafe Glycaemia, Leuven, Belgium) was discontinued from the European market in the meantime [Citation12,Citation13].
To circumvent these obstacles, use of fast-clotting serum tubes can be an alternative for glucose measurement in diagnosis of diabetes. Fast-clotting serum tubes contain thrombin as a procoagulant reducing the clotting time to less than 5 min. This enables rapid separation of serum from cells to prevent glycolysis. Rapid centrifugation can be a challenge for samples taken in hospital because of the necessity of immediate sample transport to the central laboratory. However, in primary healthcare where most of the samples for diagnosis of diabetes were taken, centrifuges are usually located directly at the point of sample collection. Fast-clotting serum tubes have been evaluated extensively since market launch. Equivalent results compared to standard serum and lithium-heparin plasma have been demonstrated for most clinical chemistry analytes, including glucose [Citation14,Citation15]. However, plasma samples in these studies were not collected in accordance with recommendations for diagnosis of diabetes by the NACB. Therefore, studies evaluating the comparability of glucose concentrations in fast-clotting serum tubes and plasma collected in accordance with the recommendations for diagnosis of diabetes used when current cut-offs were established, are lacking. Further, glucose stability at ambient temperature is important for shipping of samples. To our knowledge, no studies have documented glucose stability in fast-clotting serum tubes at ambient temperature beyond two days so far.
The aim of the study was to evaluate the suitability of fast-clotting serum tubes for glucose measurement as an alternative to the widely used NaF–EDTA–citrate tubes. In the first part, glucose concentration in fast-clotting serum tubes was compared with glucose concentration in lithium-heparin plasma as a reference as well as NaF–EDTA–citrate plasma and standard serum. In the second part, the study evaluated the stability of glucose up to five days at ambient temperature in fast-clotting serum and alternative tubes taken under recommended conditions. The stability study was conducted largely in accordance with the CRESS checklist for reporting stability studies [Citation16].
Materials and methods
Study design, samples and analyzer
The study was performed in April 2021 at the Ålesund Hospital, Aalesund, Norway. Thirty volunteers (14 men and 16 women), aged 18–62 years (median 33) were recruited to phlebotomy. The volunteers comprised 10 outpatients, 10 inpatients from the medical department and 10 volunteers from the laboratory personnel. All individuals participating in the study signed an informed consent agreement. The study was conducted in accordance with the guidelines of the Regional Committees for Medical and Health Research Ethics.
Venous blood was collected in four types of tubes, all supplied from Greiner Bio-One (Kremsmünster, Austria), following the Joint EFLM-COLABIOCLI recommendation for venous blood sampling [Citation17]: one serum separator tube with clot activator (standard serum, Vacuette, no. 4540067R, 3.5 mL), one CAT serum fast separator tube (Vacuette, no. 454591, 3.5 mL), one lithium-heparin tube (Vacuette, no. 454029, 4 mL) and one NaF–EDTA–citrate tube (FC-mix glycaemia, no. 454513, 3 mL).
After venipuncture, lithium-heparin tubes were immediately placed in an ice-water slurry according to the NACB recommendations, transported manually to the laboratory and centrifuged within 10 min after sampling at 1800 × g for 10 min in a pre-cooled refrigerated centrifuge at 4 °C.
Fast-clotting serum tubes containing gel-separator and serum separator tubes containing gel-separator were centrifuged after 10 min and 30 min, respectively, according to manufacturer’s instructions. NaF–EDTA–citrate tubes were centrifuged after 20 min because of practical reasons concerning the centrifugation capacity. In contrast to manufacturer’s recommendation, the clotting time of fast-clotting serum tubes was extended from 5 min to 10 min because of incomplete clotting after 5 min in some tubes observed earlier. All samples were centrifuged at room-temperature (20–22 °C) at 1800 × g for 10 min. After centrifugation, plasma and serum were separated from the cells and glucose was measured immediately after separation in lithium-heparin plasma, fast-clotting serum, NaF–EDTA–citrate plasma and standard serum. This timepoint was defined as timepoint 0 (T0). The relative bias between baseline lithium-heparin and fast-clotting serum, NaF–EDTA–citrate plasma and serum glucose was calculated using the following formula:
For the evaluation of glucose stability, the samples were stored in the dark at 20–22 °C and reanalyzed after 24, 48, 72, 96 and 120 h, respectively. Prior to analysis, samples were inverted five times.
Glucose measurement was performed on a Roche Cobas c702 analyzer (Roche Diagnostics, Oslo, Norway) by single measurement using the hexokinase method (reagent lot 506161, calibrator lot 410091, Roche Diagnostics, Oslo, Norway) traceable to the glucose ID-GCMS method. All concentrations were compared to the initial glucose concentrations measured at T0 (100%). The relative bias between glucose concentration at T0 and different timepoints (Tx) was calculated using the formula:
In the study period, glucose internal quality control (IQC) was run two times a day at two levels, at 5.7 mmol/L and 13.4 mmol/L (Precicontrol ClinChem Multi 1, lot 324213 and Precicontrol ClinChem Multi 2, lot 410032, Roche Diagnostics, Oslo, Norway). The inter-assay coefficient of variation (CVA) in this period was 1.2% at 5.7 mmol/L and 0.8% at 13.4 mmol/L. The laboratory participates in an external quality control program (EQA) four times a year using commutable control material with target values based on reference method. All EQA-measurements in the last two years fulfilled the national quality requirements for glucose measurement (total allowable error <7.4%).
Statistical analysis
Statistical analysis was performed using IBM SPSS Statistics software (version 27, IBM Corp., Armonk, NY) and Sigmaplot software (version 14.5, Sigmaplot Software Inc., San Jose, CA).
Sample data were tested for normality using the Shapiro–Wilk test. Because of non-normally distributed glucose concentrations, results were presented as median and interquartile range. Pairwise differences between the different tube types and lithium-heparin plasma at T0 were compared using the Wilcoxon signed rank test.
Significance of glucose-loss over all timepoints was tested using Friedman’s test. Significance values were adjusted by post hoc Bonferroni’s correction for multiple tests. A p value of <.05 was considered statistically significant.
The clinical acceptance limits for glucose stability were defined as ±2.4% for bias and ±6.5% for total allowable error based on biological variation in line with the Milan hierarchy [Citation18]. Data for biological variation were taken from the EFLM Biological Variation Database [Citation19].
Results
Glucose concentration in fast-clotting serum, NaF–EDTA–citrate plasma and serum was compared to glucose concentration in optimal handled lithium-heparin plasma according to the recommendation of the NACB. Thirty samples covering a glucose concentration range of 4.32–7.60 mmol/L were used for comparison. Median glucose concentration measured in all four tube types and the mean biases from reference sample are presented in . The median glucose concentration (IQR) at T0 varied between different tubes with 5.09 (4.76–5.68) mmol/L the lowest and 5.47 (5.06–6.07) mmol/L the highest concentration. Glucose concentration in NaF–EDTA–citrate plasma was significantly higher than in lithium-heparin plasma, while median concentration in serum was significantly lower compared to lithium-heparin plasma. In contrast, the median glucose concentration in fast-clotting serum was shown to be almost equal to lithium-heparin plasma.
Table 1. Median glucose concentration (1st-3rd quartile) at T0 according to tube type.
In part two of the study, stability of glucose at 20–22 °C after centrifugation was studied in fast-clotting serum, NaF–EDTA–citrate plasma and standard serum over five days. The median glucose concentrations at T0 and after 24, 48, 72, 96 and 120 h of storage are shown in . The maximum decrease of median glucose concentration over five days was observed in fast-clotting serum tubes after 96 h (–0.08 mmol/L). The relative differences between both the mean glucose concentration at different time points and T0 (100%) and individual glucose concentrations at different time points and T0 (100%) were used for evaluating glucose stability (). For all tubes, the relative bias including the 90% confidence interval was below ±2.4% compared to T0 at all time points. Further, individual glucose concentrations do not differ by more than 6.5% from the concentration at T0.
Figure 1. Relative difference of glucose concentration (%) vs. T0 after storage of centrifuged samples at 20–22 °C according to tube type. Mean difference between baseline glucose and glucose after storage is shown with red data points (90% confidence interval). Individual differences are shown as grey data points. The red dashed lines present the maximal allowable bias, the grey dashed lines present the maximal allowable total error. (A) Fast-clotting serum, (B) NaF–EDTA–citrate plasma and (C) serum.
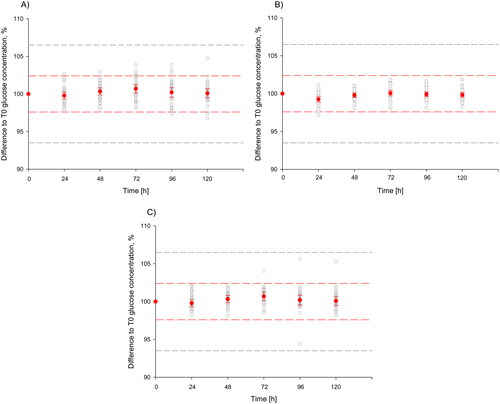
Table 2. Median glucose concentration (1st–3rd quartile) at 20–22 °C according to tube type and storage time.
The observed differences fulfill the acceptance limits for stability based on biological variation.
Discussion
Here, we report the evaluation of fast-clotting serum tubes for the measurement of glucose for diagnosis of GDM and DM. Widely used tubes containing a fluoride–citrate mixture stabilize glucose immediately after venipuncture and have been shown to be superior to standard serum separator tubes with clot activator, due to delayed processing of serum and ongoing glycolysis until separation from cells [Citation20,Citation21]. Bonetti et al. demonstrated that even a delay of 1 h before centrifugation can result in 12% lower glucose concentration compared to lithium-heparin tubes placed immediately in an ice-water slurry after venipuncture [Citation21].
However, NaF–EDTA–citrate tubes have been shown to give significantly higher glucose measurements compared to optimally processed plasma according to NACB recommendations.
Further, challenges have been reported in dissolving fluoride–citrate granula. Incomplete dissolving of granula or powder additives leads to reduced glucose stability in uncentrifuged samples. Winter et al. reported problems to dissolve the stabilizer despite of correct mixing [Citation12]. In their study, they observed that thoroughly mixing by invasion according to the manufacturer’s recommendation was not sufficient in many cases because non-dissolved granules were still visible in the tubes. The manufacturer of the tubes confirmed that mixing is critical for stabilization. Because the manufacturer could not guarantee that the recommendation for inversion of the tubes is strictly followed and hence to ensure the integrity of correct glucose stabilization, the stability of glucose in these tubes was reduced to 24 h at room temperature without centrifugation.
To circumvent these issues, fast-clotting serum tubes can be an alternative, as they allow for rapid separation of serum from cells. Since currently used cut-off values for DM and GDM were established using the tubes placed in an ice-water slurry, we chose this procedure as a reference in our study [Citation2]. In the first part of our study, we compared glucose concentration in centrifuged fast-clotting serum tubes, NaF–EDTA–citrate tubes and serum tubes with lithium-heparin tubes. We found a systematic positive bias of 4.7% for glucose measurement in NaF–EDTA–citrate plasma compared to lithium-heparin plasma placed in an ice-water slurry. This is in agreement with findings by Carey et al. [Citation5] and Saracevic et al. [Citation6]. The bias is clinically relevant as it increases the rate of GDM and DM diagnosis. However, other studies showed no significant bias in glucose measurement between tubes containing granulated NaF–EDTA–citrate and optimally handled NaF–oxalate plasma [Citation22].
In contrast, glucose measurement in fast-clotting serum tubes centrifuged 10 min after sampling and in lithium-heparin plasma tubes showed a statistically, but not clinically significant bias between the tubes. It is crucial to centrifuge the tubes 10 min after blood collection in order to timely separate serum from cells. This can be a challenge in hospital laboratories because distractions, e.g. phone calls or difficult sampling of incoming patients could delay loading samples in a timely manner. However, most of the oGTT for diagnosis of GDM were conducted in primary healthcare where centrifugation at defined timepoints is far less complicated.
The manufacturer of the fast-clotting serum tubes recommends centrifugation after 5 min. However, we decided to centrifuge the tubes after 10 min, because of incomplete clotting after 5 min observed in some tubes earlier.
Serum centrifuged 30 min after sampling showed 2.6% lower glucose concentration due to delayed processing. This is in agreement with earlier studies [Citation23].
In the second part of our study, we confirmed glucose stability up to 120 h at 20–22 °C after centrifugation in fast-clotting serum, NaF–EDTA–citrate plasma and serum. The results for serum and NaF–EDTA–citrate plasma are in agreement with findings by Winter et al. who confirmed glucose stability in these tubes up to 96 h [Citation12]. It should be noted that Winter et al. used different acceptance limits for stability. Winter et al. based the acceptance limits on the minimal difference (MD) which describes the smallest statistically significant difference between two adjacent measurements. The MD depends on the imprecision of the analytical method resulting in wider acceptance limits (±0.26 mmol/L) for the mean glucose concentration and the corresponding confidence interval. In contrast, the acceptance limits in our study are based solely on biological variation and are in principle independent from analytical imprecision. This results in much narrower acceptance criteria for glucose stability in our study. One has to keep in mind that data on biological variation depend on analytical imprecision [Citation24]. Although individual glucose concentrations do not differ by more than 6.5% from the concentration at T0 and fulfill the acceptance criteria for stability, it should be noted that the dispersion of the individual differences is lowest in NaF–EDTA–citrate plasma at timepoints 48, 72, 96 and 120 h. This is likely due to the prompt inhibition of glycolysis after sampling in citrate-containing tubes and the interindividual variability of ongoing glycolysis in serum until centrifugation.
The study has some limitations. The stability study was conducted in accordance with the CRESS checklist for reporting stability studies. However, we have not calculated an instability equation since the general stability of glucose in uncentrifuged samples is known in the literature and the aim of our study was to study the performance of CAT serum fast separator tubes as an alternative to NaF–EDTA–citrate tubes in the diagnosis of GDM and DM. In this study, 30 samples were used. The number could limit the confidence in the statistical estimates. However, our study was conducted as a combined stability study and comparison study. A number of 30 samples were considered as sufficient in line with the recommendations by the Norwegian Organization for Quality Improvement of Laboratory Examinations (NOKLUS) for the conduction of stability studies which recommends at least 20 samples. Further, the number of samples is in agreement with most of the stability studies published in the last 20 years dealing with this topic [Citation25]. Another possible limitation of the study is that glucose measurement was carried out in single measurement and not in replicate measurement. The analytical variation of glucose measurement is low and for reasons of practicability, costs and resources, single glucose measurement was considered as appropriate for both parts of the study.
Our results confirm clinically equivalent glucose concentrations in fast-clotting serum and lithium-heparin plasma immediately placed in an ice-water slurry. In addition, we confirmed glucose stability up to five days at 20–22 °C in fast-clotting serum after centrifugation. Meanwhile, the NOKLUS recommends the use of fast-clotting serum tubes in their national guidelines for diagnosis of GDM and DM.
Conclusions
The present study demonstrates that glucose concentration in fast-clotting serum generated by centrifugation of CAT serum fast separator tubes is not clinically significantly different from glucose concentration in lithium-heparin plasma placed immediately in an ice-water slurry after sampling. This assures correct diagnosis of GDM and DM when using established diagnostic cut-off values. Use of fast-clotting serum tubes can avoid significant systematic higher glucose concentrations which have been reported and confirmed for the widely used NaF–EDTA–citrate tubes.
After centrifugation, glucose is stable in fast-clotting serum up to five days at 20–22 °C without clinically significant changes. This enables shipping of samples to the central laboratory over long distances without loss of glucose due to glycolysis. CAT serum fast separator tubes can be a practical alternative for use in the diagnosis of GDM and DM.
Disclosure statement
No potential conflict of interest was reported by the author(s).
References
- Diagnostic criteria and classification of hyperglycemia first detected in pregnancy: a World Health Organization Guideline. Diabetes Res Clin Pract. 2014;103(3):341–363.
- Sacks DB, Arnold M, Bakris GL, et al. Guidelines and recommendations for laboratory analysis in the diagnosis and management of diabetes mellitus. Clin Chem. 2023;69(8):808–868. doi: 10.1093/clinchem/hvad080.
- Chan AY, Swaminathan R, Cockram CS. Effectiveness of sodium fluoride as a preservative of glucose in blood. Clin Chem. 1989;35(2):315–317. doi: 10.1093/clinchem/35.2.315.
- World Health Organization. Definition and diagnosis of diabetes mellitus and intermediate hyperglycemia. Geneva, Switzerland: WHO; 2006.
- Carey R, Lunt H, Heenan HF, et al. Collection tubes containing citrate stabiliser over-estimate plasma glucose, when compared to other samples undergoing immediate plasma separation. Clin Biochem. 2016;49(18):1406–1411. doi: 10.1016/j.clinbiochem.2016.05.017.
- Saracevic A, Dukic L, Juricic G, et al. Various glycolysis inhibitor-containing tubes for glucose measurement cannot be used interchangeably due to clinically unacceptable biases between them. Clin Chem Lab Med. 2018;56(2):236–241. doi: 10.1515/cclm-2017-0279.
- Orth M, Hawran H, Ulloor J, et al. Effects of different tube types on patient classification using current diabetes decision limits. Pract Lab Med. 2019;17:e00134. doi: 10.1016/j.plabm.2019.e00134.
- Song D, Lia M, Hurley JC. Recommended pre-analytical plasma glucose sampling methodology may distort gestational diabetes mellitus prevalence: implications for diagnostic thresholds. Diabet Med. 2019;36(10):1226–1233. doi: 10.1111/dme.14073.
- Szoke D, Borille S, Cardellicchio M, et al. Impact of optimizing pre-analytical phase on the diagnosis of gestational diabetes and related outcomes. Clin Chem Lab Med. 2021;59(12):1981–1987. doi: 10.1515/cclm-2021-0706.
- Juricic G, Kopcinovic LM, Saracevic A, et al. Liquid citrate acidification introduces significant glucose bias and leads to misclassification of patients with diabetes. Clin Chem Lab Med. 2016;54(2):363–371.
- van den Berg SAA, Thelen MHM, Salden LPW, et al. It takes acid, rather than ice, to freeze glucose. Sci Rep. 2015;5(1):8875. doi: 10.1038/srep08875.
- Winter T, Hannemann A, Suchsland J, et al. Long-terms stability of glucose: glycolysis inhibitor vs. gel barrier tubes. Clin Chem Lab Med. 2018;56(8):1251–1258. doi: 10.1515/cclm-2017-0860.
- Winter T, Greise A, Nauck M, et al. Long-terms stability of glucose: 96-h study using terumo glycaemia tubes. Clin Chem Lab Med. 2016;54(3):407–410.
- Yan R, Lou A, Watts G, et al. Comparison of Becton Dickinson vacutainer rapid serum tube with serum separator tube for routine chemistry and immunoassay tests. J Clin Pathol. 2014;67(7):599–604. doi: 10.1136/jclinpath-2013-202130.
- Dimeski G, Johnston J, Masci PP, et al. Evaluation of the Greiner Bio-One serum separator BCA fast clot tube. Clin Chem Lab Med. 2017;55(8):1135–1141. doi: 10.1515/cclm-2016-0806.
- Cornes M, Simundic AM, Cadamuro J, et al. The CRESS checklist for reporting stability studies: on behalf of the European Federation of Clinical Chemistry and Laboratory Medicine (EFLM) Working Group for the Preanalytical Phase (WG-Pre). Clin Chem Lab Med. 2021;59(1):59–69. doi: 10.1515/cclm-2020-0061.
- Simundic AM, Bölenius K, Cadamuro J, et al. Joint EFLM-COLABIOCLI recommendation for venous blood sampling. Clin Chem Lab Med. 2018;56(12):2015–2038. doi: 10.1515/cclm-2018-0602.
- Sandberg S, Fraser CG, Horvath AR, et al. Defining analytical performance specifications: consensus statement from the 1st strategic conference of the European Federation of Clinical Chemistry and Laboratory Medicine. Clin Chem Lab Med. 2015;53(6):833–835.
- Aarsand AK, Fernandez-Calle P, Webster C, et al. The EFLM Biological Variation Database; 2021 [cited 2021 Mar 2]. Available from: http://biologicalvariation.eu/
- Del Pino IG, Constanso I, Mourìn LV, et al. Citric/citrate buffer: an effective antiglycolytic agent. Clin Chem Lab Med. 2013;51(10):1943–1949. doi: 10.1515/cclm-2012-0735.
- Bonetti G, Cancelli V, Coccoli G, et al. Which sample tube should be used for routine glucose determination? Prim Care Diabetes. 2016;10(3):227–232. doi: 10.1016/j.pcd.2015.11.003.
- van der Hagen EAE, Fokkert MJ, Kleefman AMD, et al. Technical and clinical validation of the Greiner FC-Mix glycaemia tube. Clin Chem Lab Med. 2017;55(10):1530–1536.
- Stahl M, Joergensen LGM, Petersen PH, et al. Optimization of preanalytical conditions and analysis of plasma glucose. 1. Impact of the new WHO and ADA recommendations on diagnosis of diabetes mellitus. Scand J Clin Lab Invest. 2001;61(3):169–180.
- Sandberg S, Carobene A, Bartlett B, et al. Biological variation: recent development and future challenges. Clin Chem Lab Med. 2023;61(5):741–750. doi: 10.1515/cclm-2022-1255.
- Gómez Rioja R, Martínez Espartosa D, Segovia M, et al. Laboratory sample stability. Is it possible to define a consensus stability function? An example of five blood magnitudes. Clin Chem Lab Med. 2018;56(11):1806–1818. doi: 10.1515/cclm-2017-1189.