Abstract
Natural and semi-synthetic cannabinoid analogs are getting increasing media attention for their recreative use as an alternative to traditional cannabis, in Sweden as well as internationally. To investigate an increasing number of urine samples incoming to our clinical laboratory that were screening positive, using a CEDIA THC-COOH immunoassay from ThermoFisher Scientific, but then testing negative using GC-MS based verification analysis, we developed an LC-MS/MS-method for verification of hexahydrocannabinol (HHC) and Δ8-tetrahydrocannabinol. Assessment of HHC intake was based on identification of the following four metabolites: 11-nor-9(R)-carboxy-hexahydrocannabinol (R-HHC-COOH), 11-nor-9(S)-carboxy-hexahydrocannabinol (S-HHC-COOH), 11-hydroxy-9(R)-hexahydrocannabinol (R-HHC-OH) and 11-hydroxy-9(S)-hexahydrocannabinol (S-HHC-OH). Out of 46 urine samples analysed in this study, 44 showed presence of HHC-metabolites, which indicate HHC as the main explanation for an increased number of negative verifications for THC-COOH. In these samples, the HHC-OH metabolites occurred at a higher concentration than R-HHC-COOH while S-HHC-COOH was only detected in few samples at low concentrations. R-HHC-COOH and S-HHC-COOH can easily be added to a pre-existing verification method for THC-COOH, and still show acceptable results, while HHC-OH requires an enzyme capable of hydrolysing the ether glucuronide bond.
Background
Recreational use of Cannabis Sativa products is common, with analytical results consistent with cannabis use being a frequent finding at the clinical laboratory. Local culture and legislation are believed to influence trends in marijuana distribution and consumption, with recent, global tendencies towards decriminalization and legislation contributing to more wide-spread consumption patterns [Citation1]. In Sweden, albeit illegal, cannabis is the most commonly used illegal psychoactive product [Citation2].
Traditional marijuana use entails an intake of Δ9-tetrahydrocannabinol (Δ9-THC) – the main psychoactive compound in C. Sativa. Retailer and consumer efforts to circumvent legislation on controlled substances have led to a rise in the availability of cannabinoid analogs – natural, semi-synthetic and synthetic compounds acting on cannabinoid receptors, on the commercial market [Citation3]. Two such analogs are the Δ8-THC, a naturally occurring, structural isomer that differs from Δ9-THC on the placement of a double bond, and hexahydrocannabinol (HHC) that lacks said double bond. With respect to Swedish legislation, Δ8-THC was subject to a long-standing ban on C. Sativa family compounds. Nonetheless, in 2020, a transient increase was reported in the number of detected Δ8-THC in a clinical laboratory in Stockholm [Citation2]. HHC is semi-synthetic, predominantly synthesized from cannabidiol [Citation4] and not subjected to an antecedent family ban. First detected by Swedish Customs in October 2022, it was criminalized in July 2023 [Citation5].
At Örebro University hospital, we adhere to common laboratory protocol on drugs of abuse measurement in urine based on an initial immunoassay-based screening, followed by verification of positive findings by mass-spectrometry based analysis. In 2023, samples testing positive on screening analysis but negative for Δ9-THC-COOH on verification was a phenomenon that was increasingly observed at our laboratory. Immunoassays, based on antibody detection with varying specificity, often cross-react with structurally similar compounds [Citation6], and based on the suspicion that the positive screening results could be attributable to Δ8-THC-COOH or HHC metabolites, we devised a liquid chromatography-tandem mass spectrometry assay (LC-MS/MS) capable of detecting these compounds. Patient samples from clinical routine, referred to our laboratory and featuring this pattern of positive screening and negative, or suspiciously low concentrations, on verification, were included in the study.
Whether 11-nor-9-carboxy-hexahydrocannabinol (HHC-COOH) is a metabolite to HHC in humans was unknown for a long time [Citation7]. In a recent study, however, it was concluded that HHC-COOH, and its glucuronide are indeed metabolites to HHC, but they were determined not to be the main metabolites [Citation8]. It was however very recently reported that 8(R)OH-9(R)-HHC is the main metabolite found in urine [Citation9]. To the best of our knowledge, none of the glucuronides are available as reference compounds. Since HHC-COOH and HHC-OH are available as reference compounds it was of interest to investigate the possibility to verify suspected HHC intake with these two metabolites using LC-MS/MS.
Materials and methods
Method validation, chemicals
(9R)-11-nor-9-carboxy-hexahydrocannabinol (R-HHC-COOH), (9S)-11-nor-9-carboxy-hexahydrocannabinol (S-HHC-COOH), 11-OH-9(R)-Hexahydrocannabinol (R-HHC-OH) and 11-OH-9(S)-Hexahydrocannabinol (S-HHC-COOH) certified reference materials were obtained from Cayman Chemicals (Cayman Chemical Company, Ann Arbor, USA), and were dissolved in methanol, yielding 1.0 mg/ml. (−)-11-nor-9-Carboxy-Δ9-THC solution (Δ9-THC-COOH) T-019, (-)-11-nor-9-Carboxy-Δ8-THC (Δ8-THC-COOH) solution T-170, and (+)-11-Nor-Δ9-THC-9-carboxylic acid glucuronide (Δ9-THC-COOH-glucuronide) solution T-038 were obtained from Cerilliant (Cerilliant Corporation, Round Rock, USA). THC Internal standard (±)-11-nor-9-Carboxy-Δ9-THC-D9 solution T-009 was obtained from Cerilliant and was diluted to 2000 µg/L with methanol. The water used was 18.2 MΩ ultra-pure water produced in-house using an Elga (Elga LabWater, High Wycombe, UK) Purelab Chorus 1 system. Methanol from Merck (Merck KGaA, Darmstadt, Germany), LiChrosolv for Liquid Chromatography. Acetonitrile from Honeywell (Honeywell Research Chemicals, Charlotte, USA), for LC-MS. Formic acid from VWR (VWR International, Radnor, USA), AnalaR Normapur. B-One beta-glucuronidase from Kura Biotech (Kura Biotech, Los Lagos, Chile).
LC-MS
The instrumentation consisted of a Waters Acquity UPLC I-Class system with binary pump and FTN-injector fitted with a sample organizer. A Waters Xevo TQ-XS triple quadrupole mass spectrometer with electrospray was used for detection. A Waters Acquity HSS T3 (1,8 µm 100*2,1 mm) column was employed for analysis. Injection volume was 0.5 µl. Mobile phase A was water with 0.1% formic acid and mobile phase B neat acetonitrile. Column flow rate was 0.6 ml/min with the following gradient: time 0 min, 40% B, 1.7 min, 80% B, 1.71 min, 100% B, 2.0 min, 100% B, 2.05 min, 40% B, 2.7 min, 40% B. Total run time was 2.7 min. Column oven was set at 50 °C and sample compartment was set at 6 °C. This method separates R-HHC-COOH from S-HHC-COOH, but R-HHC-OH and S-HHC-OH coelute and were calibrated using R-HHC-OH, see . Δ8-THC-COOH shows peak overlap with Δ9-THC-COOH. The retention time difference allows for identification, but not an accurate quantification in presence of Δ9-THC-COOH, see .
Figure 1. MS-chromatograms for co-eluting R/S-HHC-OH (top), R-HHC-COOH and S-HHC-COOH (mid), and THC-COOH-D9 internal standard (bottom).
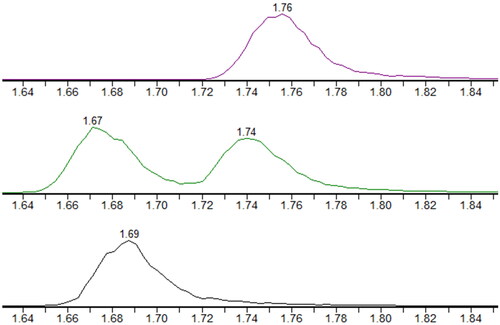
Detection was carried out in positive mode. The electrospray interface was operated with a capillary voltage of 3 kV, source temperature of 150 °C, desolvation gas temperature of 600 °C, desolvation gas flow of 900 L/hr and a cone gas flow of 150 L/hr. MS/MS transitions with the following parameters were used with a cone voltage of 2 V; for R/S-HHC-COOH (347 > 193 collision energy 26 and 347 > 121 collision energy 22), for R/S-HHC-OH (333 > 193 collision energy 24 and 333 > 123 collision energy 40), for HHC-COOH-glucuronide (523 > 347 collision energy 20, and 523 > 193 collision energy 40) and for HHC-OH-glucuronide (509 > 333 collision energy 17, and 509 > 193 collision energy 40). Data was acquired and processed using Waters Mass Lynx 4.2 software.
Sample preparation
Calibrator stock solution was prepared at 50 000, 5 000, 5000 and 500 µg/L in methanol with R-HHC-COOH, S-HHC-COOH, R-HHC-OH and Δ8-THC-COOH, which were spiked manually into blank urine in the 96-well plate, resulting in 10 000, 1000, 100 and 10 µg/L of each analyte. Sample preparation was carried out using a Tecan Evo with fixed tips. Pipetting of calibrators and samples (50 µL) was followed by 10 µL methanol and 100 µL B-One beta-glucuronidase. The plate was shaken and incubated for 60 min at 37 °C. Further addition of 20 µL internal standard, 300 µL acetonitrile, shaking, adding 50 µL water and a final shaking, completed sample preparation. Non-hydrolyzed samples were prepared identically, but using water instead of enzyme and omitting incubation at 37 °C. Alkaline hydrolysis was performed by addition of 10 µL of 5 M NaOH to 100 µL sample. Incubation for 30 min at room temperature was followed by neutralization using 30 µL of 50% acetic acid. Further addition of 20 µL internal standard, 600 µL acetonitrile, 300 µL water and shaking completed sample preparation.
Clinical samples
Clinical samples, from routine healthcare, collected at the Clinic of Dependency Disorders and the Psychiatric Clinic at Örebro University Hospital, were used in this study. Drugs of abuse testing is performed to aid in the diagnosis, treatment and follow-up of patients who are admitted on a voluntary basis because of substance-use dependence, or a psychiatric condition where dependency is a risk- or co-factor of the diagnosis. The samples were referred for analysis at the Department of Clinical Chemistry between 30th august and 6th november 2023. Urine samples were collected in plastic tubes. The protocol in effect at our laboratory has samples from clinical routine normally discarded after analysis. These samples were instead salvaged for inclusion in this study, subsequently anonymized, keeping only information on the age and sex of the patient. Legislation in effect in Sweden stipulates that approval from the national Swedish Ethical Review Authority is not required when conducting research involving anonymized samples that cannot be traced back to the individual patient. Initial screening analysis was performed using a Siemens Advia 1800 platform (Siemens Healthineers, Erlangen, Germany) with Thermo Scientific Cedia THC reagents (Thermo Fisher Scientific, Waltham, MA), using 25 µg/L as cut-off. Samples testing positive were further processed for verification using a traditional GC-MS (Agilent 5975 C single quadrupole MS, 7890S GC and 7693 auto-sampler, Agilent Technologies, Santa Clara, CA) method involving alkaline hydrolysis, SPE work-up and derivatisation using MSTFA. A cut off of 10 µg/L was used to determine that a sample was positive for THC.
Results
Between 30th august −23 and 6th november −23, a total of 4512 urine samples, on which THC measurement was requested, were sent to our laboratory, and 512 were screened positive. Out of these, 26, or 5%, tested negative using GC-MS based verification analysis for Δ9-THC-COOH. A further 20 samples with lower than expected levels on verification compared to the screening concentration were included in this study. The inclusion criterion was that screen to verification ratio was >4. This amounted to 46 samples in total. Because some patients were repeatedly tested, the number of unique patients whose samples were included were 24; 17 men and 7 women, with ages ranging from 16-50 y. See for details on the baseline characteristics of the samples used in the study.
Table 1. Baseline characteristics of the studied samples.
Method validation
Linearity and LOQ
Linearity was assessed for R-HHC-OH and R-HHC-COOH from 10 000 to 1 µg/L with 13 concentrations. Linearity was acceptable down to 10 µg/L, but below that, the response was declining particularly for R-HHC-OH. Matrix effects were assessed at 500 and 10 µg/L using 8 different blank urine matrices. Matrix effects were below 10% in all cases and internal standard compensated for this further, such that average matrix effects were below 3% and CV’s over 8 matrices were all below 5%.
Recovery
Recovery of analytes is strongly affected by adsorption to plastic surfaces, and adsorption is typically dependent on lipophilicity, pH, volume-to-surface ratio, and type of surface, which makes cannabinoid analysis difficult [Citation10]. No systematic investigation mas made, however, it was confirmed experimentally that adsorption of R-HHC-OH and R-HHC-COOH to the plastic container is significant in urine (up to 80% adsorption), but that a final concentration of 60% acetonitrile in the 96 well plate was enough to preclude adsorption while still not resulting in injection effects in LC-MS/MS.
Hydrolysis
Initial attempts to hydrolyze patient samples using alkali, afforded R/S-HHC-COOH as expected, but only small amounts of R/S-HHC-OH. In order to monitor hydrolysis of conjugates, a parent search MRM-experiment was carried out, identifying conjugates of HHC-OH and HHC-COOH. These conjugate peaks also produced identical peaks in the MRM for corresponding unconjugated analytes, through in-source-fragmentation. MS conditions for conjugate transitions were optimized by injecting a sample under varying MS-conditions. The HHC-COOH-glucuronide was found to co-elute with Δ9-THC-COOH-glucuronide (certified reference material), which thus supported identity. HHC-OH-glucuronide was found to exist as two different peaks, consistent with finding the two different R/S-HHC-OH-isomers in patient samples using a slower gradient LC-run than what was used for the actual quantification. HHC-OH-glucuronides were also found to elute near the HHC-COOH-glucuronide, which supported identity. When trying out enzymatic hydrolysis conditions, it was observed that presumed conjugate peaks disappeared, and HHC-OH and HHC-COOH was formed. It was noted that HHC-COOH hydrolyzed quickly already at room temperature, while HHC-OH-glucuronide required longer reaction time and elevated temperature. After trials with different reaction times and temperatures, the final enzymatic hydrolysis protocol was proposed.
All samples were analyzed with and without enzymatic hydrolysis. It was observed that peak areas of conjugates without hydrolysis correlated reasonably well with assessed levels of corresponding analytes, which further supported correct identification of conjugates. The degree of conversion on hydrolysis was estimated as peak area of conjugate with/without hydrolysis. Conversion degrees were thus estimated to 96% on average for HHC-COOH, with all samples but four showing a conversion degree >95%. Conversion degrees for HHC-OH were estimated similarly to >98% for all samples.
Alkaline hydrolysis resulted in slightly higher levels of HHC-COOH compared to enzymatic cleavage. In three cases where enzymatic conversion degrees were notably low, alkaline hydrolysis turned out to produce HHC-COOH-levels significantly higher than the enzymatic method.
The degree of conjugation could also be estimated from the non-hydrolysis/hydrolysis ratio. The degree of conjugation of HHC-OH thus was more than 98% in all samples except one. This figure is however likely affected by the fact that HHC-OH is fairly hydrophobic, and will stick to the plastic test tube to a significant degree if present in patient samples. HHC-COOH similarly showed a degree of conjugation of 81% on average, however with large individual variation.
Δ8-THC-COOH was identified in only five samples, which were all positive for both HHC-OH and HHC-COOH. Δ8-THC-COOH was not quantified, but identified by comparing retention time and ion-ratio to that of certified reference material. The ion ratio for Δ8-THC-COOH was ca 1.2 compared to ca 2.2 for Δ9-THC-COOH, and retention time relative to the internal standard was 0.97 for Δ8-THC-COOH compared to 1.02 for Δ9-THC-COOH. Thus, this method could unambiguously differentiate these isobars as long as they were not both present in the same sample, see .
Measurement on clinical samples
Out of 46 samples in the study, 32 were found positive for R-HHC-COOH and 38 for R/S-HHC-OH using a cut-off of 10 µg/L. S-HHC-COOH was only found positive in 6 samples, all with concentrations below 50 µg/L and all showing higher levels of R-HHC-COOH. If the positive identification of a metabolite is used as the only criterion (correct retention time and ion-ratio), then 44 of 46 samples could be judged as consistent with HHC intake. If samples positive for Δ9-THC-COOH on verification were excluded, a pool of 26 samples would be obtained, of which 22 were positive for R-HHC-COOH and 25 were positive for R/S-HHC-OH. Measured concentrations of R/S-HHC-OH were higher than R-HHC-COOH in most samples. Excluding samples with no identifiable HHC-OH or HHC-COOH, 36 of 41 samples showed HHC-OH levels higher than HHC-COOH. The ratio R/S-HHC-OH over R-HHC-COOH was 2.0 on average for positive samples.
Discussion
In this study, we devised an LC-MS/MS assay capable of identification of Δ8-THC-COOH and quantification of R-HHC-COOH, S-HHC-COOH and R/S-HHC-OH in urine, with the purpose of examining an increasingly frequently occurring phenomenon of clinical samples testing positive on screening using an enzymatic immunoassay, but testing negative for Δ9-THC-COOH on follow up verification analysis by GC-MS. In 2021, reports of increasing seizures by Swedish Customs of Δ8-THC, followed by clinical accounts on increasing frequencies of laboratory detection of cannabinoid analogs [Citation11], as well as HHC seizures by Customs in 2022, made cannabinoid analogs a likely explanation. Using the present LC-MS/MS assay, we observed that a majority of studied samples showed presence of Δ8-THC or HHC (), which we consider to reflect a recent surge in consumption. While the low number of samples positive for HHC in the study makes inferences of prevalence uncertain, the broad age range and somewhat high percentage of women, 7 out of 24, may suggest that HHC consumption is not restricted to a single demographic, unlike traditional cannabis use in Sweden, which is more common among the young, and more common among men than women [Citation12].
In retrospect, this emergence was predictable based on the reports from Swedish Customs, and our findings concord with previous experience and the notion that laboratories offering drugs of abuse testing should be sensitive to trends in patterns of consumption [Citation13,Citation14]. This is particularly important when it comes to new or non-traditional psychoactive compounds, where having strategies or protocols established on how to deal with changing trends in drug consumption that may be reflected in unusual screening/verification results.
Experientially, cannabinoid analog use in many countries is related to regulatory status [Citation15], with trends of surging consumption of not yet illegalized compounds that vanes after they are legally controlled. Nonetheless, Δ8-THC, that was subject to a Cannabinoid family ban in Sweden, still saw an increase in 2021, likely following increased availability on international markets. At our laboratory, 39 of the 46 studied samples (arrived 30th august −23 and 6th november −23 in the laboratory), tested positive for HHC, while only 5 tested positive for Δ8-THC, suggesting that HHC constituted most of the increased cannabinoid analog consumption. The impact from the ban, effected in July 2023, on HHC prevalence remains to be seen. Concordant with the findings of Wolf et al. who examined various enzymatic immunoassays and their cross-reactivity with cannabinoid analogs [Citation16], we observed high cross-reactivity in the immunoassay in use at our laboratory with both Δ8-THC and HHC (data not shown).
There were limitations to this study, most importantly the inability to obtain commercial, certified reference material of conjugates for use in method validation. Thus, the assessment of enzyme efficacy must be seen as preliminary. The samples examined in this study were urine collected in a clinical healthcare context, and only those testing positive in the initial enzyme immunoassay were examined. This precluded any examination of possible interferences affecting screening, and any examination of possible substances testing negative on screening but possibly having similar chromatographic properties. Because of the assay design, we were also unable to identify any other cause than HHC or Δ8-THC for samples testing positive on screening, but negative for THC-COOH on verification. Further studies employing assays capable of detection of a larger selection of esoteric compounds are warranted to examine the nature of such samples.
It has been reported that HHC-COOH-glucuronide is not a main metabolite to HHC [Citation8], and very recently that 8-OH-HHC-glucuronide is the main metabolite [Citation9]. In this study, we found that R/S-HHC-OH appears to exist in higher concentrations, roughly 2 times the R-HHC-COOH concentration, which will allow for a longer detection window. Based on the 26 samples positive in screen and negative on verification for THC-COOH, 25 samples were found positive for R/S-HHC-OH (96%), 22 for R-HHC-COOH (85%), and 6 for S-HHC-COOH (23%). Considering that 8-OH-HHC-glucuronide is reported to be the main metabolite, it is possible that an even better detection window could be achieved by using this metabolite.
Alkaline hydrolysis of HHC-OH glucuronide was shown to be ineffective, which is a result that is similar to what is found with other THC metabolites [Citation17]. Previous work on THC metabolites found that it is difficult to enzymatically, simultaneously hydrolyze both THC-COOH glucuronide and THC-OH glucuronide [Citation18,Citation19]. The present work indicate that the particular enzyme used in this study work well with both HHC ester- and ether-glucuronides, but it is also acknowledged that this enzymatic protocol is more complicated and expensive than alkaline hydrolysis. Considering that more work has to be done before a definitive analyte for verification of HHC is determined, we opine that incorporation of R-HHC-COOH in a pre-existing analysis of THC-COOH based on hydrolysis is a cost efficient solution, with acceptable results.
It is our recommendation that clinical laboratories employing enzymatic immunoassays for screening take into account the cross-reactivity of similar compounds with respect to concurrent trends in drugs of abuse consumption. With access to LC-MS/MS equipment, it is possible to obtain identification of these increasingly common cross-reacting compounds, with a practical and reasonable analysis protocol, when faced with conflicting results in screening and Δ9-THC verification analysis.
Disclosure statement
No potential conflict of interest was reported by the author(s).
References
- Ladegard K, Bhatia D. Impact of cannabis legalization on adolescent cannabis use. Psychiatr Clin North Am. 2023;46(4):635–646. doi: 10.1016/j.psc.2023.03.008.
- Helander A, Villen T. [Drug use and drug trends in Sweden 2010-2020 - results from urine drug testing in the workplace]. Lakartidningen. 2021;118:21056
- Grotenhermen F. Pharmacokinetics and pharmacodynamics of cannabinoids. Clin Pharmacokinet. 2003;42(4):327–360. doi: 10.2165/00003088-200342040-00003.
- Ujvary I. Hexahydrocannabinol and closely related semi-synthetic cannabinoids: a comprehensive review. Drug Test Anal. 2024;16(2):127–161.
- Socialdepartementet. Nio nya substanser blir narkotikaklassade 2023 [2023-11-17]. Available from: https://www.regeringen.se/pressmeddelanden/2023/06/nio-nya-substanser-blir-narkotikaklassade/.
- Saitman A, Park HD, Fitzgerald RL. False-positive interferences of common urine drug screen immunoassays: a review. J Anal Toxicol. 2014;38(7):387–396. doi: 10.1093/jat/bku075.
- The European Monitoring Centre for Drugs and Drug Addiction (EMCDDA). Hexahydrocannabinol (HHC) and related substances. Accessed on 12 dec 2023. Available from: https://www.emcdda.europa.eu/publications/technical-reports/hhc-and-related-substances_en.
- Schirmer W, Auwärter V, Kaudewitz J, et al. Identification of human hexahydrocannabinol metabolites in urine. Eur J Mass Spectrom (Chichester). 2023;29(5-6):326–337. doi: 10.1177/14690667231200139.
- Di Trana A, Di Giorgi A, Sprega G, et al. Disposition of hexahydrocannabinol epimers and their metabolites in biological matrices following a single administration of smoked hexahydrocannabinol: a preliminary study. Pharmaceuticals (Basel). 2024;17(2):249. doi: 10.3390/ph17020249.
- Roth KD, Siegel NA, Johnson RW, Jr., et al. Investigation of the effects of solution composition and container material type on the loss of 11-nor-Delta 9-THC-9-carboxylic acid. J Anal Toxicol. 1996;20(5):291–300. doi: 10.1093/jat/20.5.291.
- Helander A, Johansson M, Andersson A, et al. Analytical and medico-legal problems linked to the presence of Delta-8-tetrahydrocannabinol (Delta-8-THC): results from urine drug testing in Sweden. Drug Test Anal. 2022;14(2):371–376. doi: 10.1002/dta.3190.
- Drugs ESSPoAaO. ESPAD report 2019. 2019.
- Albertson TE, Chenoweth JA, Colby DK, et al. The changing drug culture: emerging drugs of abuse and legal highs. FP Essent. 2016;441:18–24.
- Manthey J. Cannabis use in Europe: current trends and public health concerns. Int J Drug Policy. 2019;68:93–96. doi: 10.1016/j.drugpo.2019.03.006.
- Ferretti ML, Gournay LR, Bingaman MG, et al. A survey study of individuals using hexahydrocannabinol cannabis products: use patterns and perceived effects. Cannabis Cannabinoid Res. 2023 [cited 2023-11-17] [
- Wolf CE, Pokhai AA, Poklis JL, et al. The cross-reactivity of cannabinoid analogs (Delta-8-THC, Delta-10-THC and CBD), their metabolites and chiral carboxy HHC metabolites in urine of six commercially available homogeneous immunoassays. J Anal Toxicol. 2023;47(8):732–736. doi: 10.1093/jat/bkad059.
- Kemp PM, Abukhalaf IK, Manno JE, et al. Cannabinoids in humans. II. The influence of three methods of hydrolysis on the concentration of THC and two metabolites in urine. J Anal Toxicol. 1995;19(5):292–298. doi: 10.1093/jat/19.5.292.
- Aizpurua-Olaizola O, Zarandona I, Ortiz L, et al. Simultaneous quantification of major cannabinoids and metabolites in human urine and plasma by HPLC-MS/MS and enzyme-alkaline hydrolysis. Drug Test Anal. 2017;9(4):626–633. doi: 10.1002/dta.1998.
- Sempio C, Scheidweiler KB, Barnes AJ, et al. Optimization of recombinant beta-glucuronidase hydrolysis and quantification of eight urinary cannabinoids and metabolites by liquid chromatography tandem mass spectrometry. Drug Test Anal. 2018;10(3):518–529. doi: 10.1002/dta.2230.