Abstract
Objectives
The presence of intestinal flora in the gut has been linked to migraines in recent studies, but whether the association is causal or due to bias remains to be clarified. We aimed to explore whether there is a potential causal relationship between gut microbiota and migraine risk with this study.
Materials and methods
We conducted a two-sample Mendelian randomized analysis study to explore whether gut microbiota has a causal relationship with migraine using publicly available data from large-scale genome-wide association studies. The inverse variance weighting was used as the main method, and weighted median and MR-Egger were used as supplementary methods for causal inference. Sensitivity analyses, including leave-one-out analysis, Cochran Q test, and MR-Egger intercept test, were used to verify the robustness of the results.
Results
After rigorous quality control of the results, we identified that genetic predisposition towards a higher abundance of genus.Lactobacillus was causally associated with higher of migraine (IVW OR = 1.10, 95% CI = 1.03 − 1.18, p = .004), whereas the higher abundance of family.Prvotellaceae predicted a decreased risk of migraine (IVW OR = 0.89, 95% CI = 0.80 − 0.98, p = .02). Sensitivity analyses indicated the results were not biased by pleiotropy.
Conclusion
According to our research, there is evidence showing that gut microbiota may be involved in migraine development, which suggested that a stool examination might be helpful to recognize those with a higher risk of migraine. Further mechanisms remained to be elucidated in future studies.
Introduction
Migraine is a neurological condition typically characterized by recurrent unilateral or bilateral throbbing headaches accompanied by nausea, vomiting, photophobia, and phonophobia that can persist from hours to days. It is a primary brain dysfunction disease caused by genetic and environmental factors and the World Health Organization has listed it as one of the sixth most disabling diseases in the world [Citation1, Citation2]. It is well known from current epidemiological studies that the global risk of migraine is rapidly increasing and will increase by nearly 50% in the next 20 years [Citation3]. As reported, migraine still leads to a heavy mental burden for both men and women, which could consequently be harmful to the physical and mental health of humans. Besides, human society still faces a heavy economic burden from migraine [Citation2]. Therefore, detecting and preventing diseases early of migraine is urgent and important.
Migraine patients frequently experience obvious gastrointestinal issues. The most common presentations are nausea and vomiting, with a minority proportion of individuals also experiencing diarrhea, constipation, and indigestion [Citation4]. The associations between migraine and gastrointestinal symptoms suggest that gut microbiota, an important gastrointestinal factor, may contribute to migraine development.
Indeed, previous studies have reported several “axes” linking the gut with other systems. Recently, the gut-liver-brain axis has received increasing attention due to the complex interactions among the three [Citation5]. However, the mechanisms remain unclear. The gut-brain axis has been widely studied. As early as 1980, Banks first confirmed the existence of the gut-brain axis, in which gut microbiota play a critical role in regulating brain function from the bottom up [Citation6]. For instance, several central nervous system (CNS)-related health conditions, like Alzheimer’s disease, Parkinson’s disease, and Huntington’s disease, have been linked with the disturbance of gut microbiota, in which immune and endocrine regulations played a key role [Citation7–11]. For Huntington’s disease, specifically, Kong et al. provided the first specific evidence of gut dysbiosis in a Huntington’s disease model, and Wasser et al. observed the first evidence of gut dysbiosis in people diagnosed with Huntington’s disease [Citation12, Citation13]. For ALS, Zhang et al. first provided evidence of gut dysfunction and microbiome abnormalities as important components in ALS disease [Citation14]. Also, For Parkinson’s disease, Scheperjans et al. provided the first evidence of shifts in gut microbiota in patients with Parkinson’s disease [Citation15]. Except for the gut-brain axis, in recent years, the interaction between the gut and the liver has also been recognized. Studies have reported that portal vein, biliary tract, and systemic circulation might mediate the interaction between the gut and liver [Citation16]. Therefore, the role of the liver in the gut-brain axis cannot be neglected, and there might be a typical microbiota-gut-liver-brain axis disease model though the mechanisms require further investigation.
In terms of migraine, one of the CNS diseases, studies have sought to evaluate its relationship with gut microbiota but yielded inconsistent results. A recent study found that probiotics can reduce the risk of migraine attacks and protect individuals from migraine disease [Citation17]. However, another study reported that gut microbiota may not be associated with migraine disease and have no effect on the disease. The discrepant results might be ascribed to the inherent defects of observational studies, including residual reverse causality and confounding. It is necessary to investigate the causal impact of gut microbiota on migraine risk using a novel approach, given the large burden migraine brings.
Mendelian randomization (MR) is a typical epidemiological research method developed recently for causal inference [Citation18]. The method is a common and well-established method of investigating the causal relationship between an exposure and an outcome by pooling statistical data from genome-wide association studies (GWAS) while controlling for uncertain confounding effects and minimizing confounding factors (e.g. environment) [Citation19]. Instrumental variables (IVs) were selected based on genetic variants significantly associated with exposure for inferring causality [Citation20].
Conceptually, MR analysis is similar to a randomized controlled trial (RCT). Single nucleotide polymorphisms (SNPs) tightly associated with exposure were utilized as instrumental variables (IVs) for inferring causality [Citation20]. Since genetic variants are assigned randomly in fertilization, the MR estimates are less likely to be affected by confounding factors, and thereby the results will be largely unconfounded [Citation21]. The bias of reverse causation is also largely reduced because genetic variants are typically not affected by the development of the resulting trait [Citation21]. Therefore, based on the advantages mentioned above, MR is often referred to as "RCT created by nature", and is widely applied to explore causalities. As an example, Cai et al. identified several blood metabolites that could be causally related to epilepsy in a recent MR study [Citation22]. In a recent two-sample Mendelian randomization study, Bifidobacterium was causally associated with preeclampsia and eclampsia [Citation23].
In this study, we conducted a two-sample MR study to explore whether there is a causal association of gut microbiota with migraine risk. We aimed to identify influential gut microbiota, enhance the quality of the existing evidence, and may provide new insights into the prevention and treatment of migraine.
Materials and methods
Study design
We assessed gut microbiota’s causal effect on migraine using single nucleotide polymorphisms (SNPs) as instrumental variables (IVs) in a two-sample Mendelian randomization framework. To comprehensively explore whether gut microbiota was involved in migraine pathogenesis, we analyzed the causalities at five different signature levels, including phylum, class, order, family, and genus. MR research needs to meet three basic assumptions: (i) There is a strong correlation between IVs and exposure factors; (ii) There are no confounding factors associated with IVs, either observed or unobserved; (iii) It is only through exposure factor that IVs directly influence the outcome [Citation24]. The overview of the research design was shown in . All analyses in this study were performed using the “TwoSampleMR” package (version 0.5.6) based on the R program (version 4.2.2).
GWAS data for gut microbiota
The summary-level statistics for gut microbiota were obtained from a large-scale GWAS consisting of 18,340 participants from 24 cohorts (nearly 78% of European descent) conducted by the MiBioGen consortium [Citation25]. In this GWAS, Age, sex, technical covariates, and genetic principal components were adjusted. Specifically, the microbial composition was determined by targeting three distinct regions within the 16S rRNA. According to the original GWAS, the distinct regions of the 16S rRNA gene included V4, V3-V4, and V1-V2. Finally, respectively 9 phyla, 16 classes, 20 orders, 36 families, and 131 genera were tested. In detail, summary GWAS data for the abundance of bacterial taxa were retrieved from https://mibiogen.gcc.rug.nl/.
GWAS data for migraine
The genetic information for migraine came from a large-scale GWAS conducted by Gormley et al. [Citation26], comprising 37,500 participants of European ancestry. The GWAS included participants diagnosed with migraine by a doctor or those who self-reported migraine through questionnaires, which improved the sample size to improve the statistical power of the study at the expense of some precision of the phenotype determine. Quality control, principal components analysis, and imputation were conducted in the GWAS. The full summary statistics were publicly available in the GWAS Catalog (https://www.ebi.ac.uk/gwas/downloads/summary-statistics).
Quality control of IVs
In this two-sample MR study, the IVs were SNPs that were strongly associated with each gut microbiota taxon. Given that the number of IVs was insufficient in the case of limiting the threshold at p < 5 × 10−8, we used a more relaxed threshold of p < 1 × 10−5 to obtain more IVs to improve the statistical power. This strategy was generally used in previous studies [Citation27]. Furthermore, to ensure the independence of each instrumental variable, we clipped the SNPs at a threshold of linkage disequilibrium (LD) r2 < 0.1 within a 500 kb distance. Afterward, we extracted the SNPs from the outcome data and discarded those that were absent. Next, to ensure that the effect alleles were concordant between exposure and outcome SNPs, we synchronized the exposure and outcome IVs information. During this procedure, palindromic SNPs or those with incompatible alleles were eliminated. Lastly, in order to assess the degree of weak IV bias, we calculated the F-statistic for the IVs. If the F-statistic was less than 10, the IV was considered to be weak and would introduce bias to the MR estimations [Citation28].
Mendelian randomization
The inverse variance weighted (IVW) method, weighted median, and MR-Egger regression were used to infer the causality between gut microbiota and the risk of migraine. Among them, the IVW method was used as the primary method as it is the most powerful method [Citation29]. However, it is also vulnerable to bias even when there is only one invalid IV. The weighted median method is relatively robust as it allows no more than half of the IVs to be invalid [Citation30]. To exploratorily reveal the potential causalities, we determine a significant causality at p < .05 of an IVW estimate.
Sensitivity analysis
Sensitivity analyses were performed to assess the reliability of our MR results. We first conducted the “leave-one-out” analysis to appraise whether the overall IVW estimates were strongly driven by some high-influence IVs [Citation31]. Secondly, we used Cochran’s Q test to appraise the existence of heterogeneity. In detail, Cochran’s Q value with p < .05 indicated the existence of heterogeneity [Citation32]. Thirdly, the intercept derived from the MR-Egger regression was applied to evaluate the horizontal pleiotropy [Citation33]. Specifically, an intercept with p < .05 indicated the existence of pleiotropy.
Results evaluation
To screen robust causal associations between gut microbiota and migraine more rigorously, several criteria were set for the quality control of the MR results: (i) the IVW estimate was significant at p < .05; (ii) According to the MR-Egger intercept assessment, there is no horizontal pleiotropy; (iii) the “leave-one-out” analysis identified no high-influence IVs to bias the overall estimate.
Results
IVs overview
After IVs quality control, a totally 2651 SNPs were eligible to proxy gut microbiota. In detail, each bacterial taxon’s number of SNPs (9 Phyla, 16 classes, 20 orders, 32 families, and 119 genera). The F-statistics for each of the SNPs were all over 10, suggesting all the IVs were statistically sufficient for MR analysis.
Causal effect of gut microbiota on migraine
Using the IVW method, we primarily identified 12 bacterial taxa significantly associated with the risk of migraine, of which four bacterial taxa exerted a harmful effect, including genus.Lactobacillus, genus.Parabacteroides, genus.Subdoligranulum, and genus.Peptococcus, whereas the other eight taxa play a beneficial role, including genus.RuminococcaceaeUCG010, genus.RuminococcaceaeUCG011, genus.Anaerofilum, genus.LachnospiraceaeND3007group, genus.Eubacterium nodatum group, genus.Prevotella7, family.Prevotellaceae, and genus.Streptococcus (, ).
Figure 2. Potential candidate gut microbiota in the risk of migraine identified by the inverse variance weighted (IVW) method.
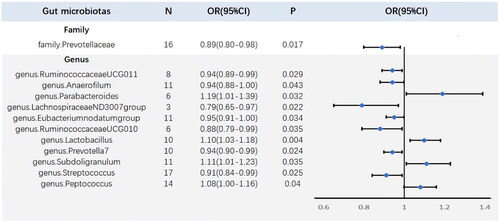
Table 1. Mendelian randomization (MR) results for the potential candidate gut microbiota in the risk of migraine.
By conducting sensitivity analysis, only two of the recognized gut microbiota passed quality control, including genus.Lactobacillus and family.Prevotellacea (). In detail, genetically predicted a higher abundance of genus.Lactobacillus causally related to a higher migraine risk (IVW OR = 1.10, 95% CI = 1.03–1.18, p = .004), whereas the higher abundance of family.Prvotellaceae predicted a lower risk of migraine (IVW OR = 0.89, 95% CI = 0.80–0.98, p = .02). The weighted median method and the MR-Egger regression yielded similar estimates (). The “leave-one-out” analysis showed that the overall IVW estimates were not strongly influenced by any single SNP, indicating the MR estimates were relatively robust (). Besides, Cochran’s Q test shows that no heterogeneity was detected (). Furthermore, the non-significant intercept derived from the MR-Egger regression suggested no horizontal pleiotropy was detected ().
Figure 3. Scatterplots for the Mendelian randomization (MR) estimation of the association between gut microbiota and the risk of migraine.
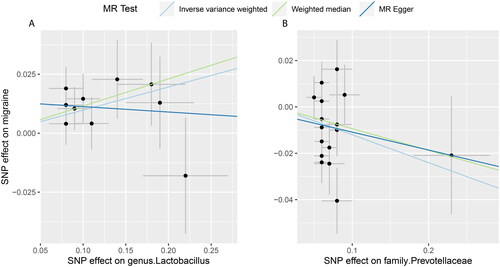
Figure 4. Leave-one-out plots for the Mendelian randomization (MR) estimation of the association between gut microbiota and the risk of migraine.
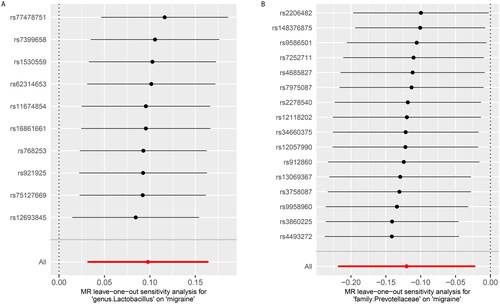
Table 2. Pleiotropy and heterogeneity test for the significant Mendelian randomization estimates.
Discussion
The main findings of the current study
In this two-sample MR study, it was found that two gut microbiotas were causally related to migraine risk, including genus.Lactobacillus and family.Prevotellacea. Specifically, we found a greater abundance of genus.Lactobacillus was associated with a higher risk of migraine, whereas a higher abundance of family.Prevotellacea was related to a decreased risk of migraine. To our knowledge, in this MR study, gut microbiota is explored in relation to migraine risk for the first time, which would extend the existing literature on the topic of the gut-brain axis.
Previous findings
There has been an exponential increase in research on the role of gut microbiota in human health in recent years [Citation34]. Disrupted gut microbiota balance has been linked to various diseases, such as psychiatric, metabolic, and autoimmune diseases [Citation35]. Many literatures have also reported that intestinal flora can regulate the host’s immunity and metabolism, and have a potential impact on human health [Citation36, Citation37]. To date, accumulating research evidence points to a potential link between gut microbiota and migraine risk. However, the results were discrepant across distinct studies. In one study, changes in gut composition of the migraine patients were observed by A. Ghavami, et al. [Citation38] In another study, researchers found that gut microbiota had no significant effect on migraine [Citation39]. Specifically, some studies have reported that Lactobacillus could alleviate migraine, but Roos and colleagues found no evidence of the association between Lactobacillus and migraine [Citation39]. In observational studies, there is always the possibility of residual confounding and reverse causality, which can create equivocal results. Therefore, a specific spectrum of gut microbiota that contributes to the development of migraine disease has not been thoroughly identified and deserves discussion.
Potential mechanisms
The GWAS for gut microbiota identified that host genetics influence the abundance of gut microbiota. Studies have reported that metabolic disturbance and obesity could influence gut microbiota [Citation40]. Meanwhile, variation in host genes might also underlie susceptibility to metabolic diseases. Therefore, the mechanisms linking host genetics with gut microbiota might be metabolic diseases [Citation41]. For migraine, The GWAS also detected genetic variables, suggesting a certain degree of heritability. Using the detected genetic information of genetic variables as IVs derived from both the GWAS, we can conduct regression analysis to evaluate whether they were significantly correlated. In this work, we observed a causal effect of the genus.Lactobacillus and family.Prevotellacea on the risk of migraine. However, the mechanisms underlying the associations are extremely limited based on the current literature and warrant further experimental investigations.
Based on the current results of our MR study, we confirmed the involvement of gut microbiota in the occurrence of migraine, suggesting gut microbiota might be a promising biomarker for migraine pathology. Studies have reported gut microbiota participate in the regulation of inflammation and immunity. Also, inflammatory factors, like TNF-α and IL-1β, were reported to be involved in the release of pain neuro-mediators during the onset of migraine [Citation42, Citation43]. Thereby, Mechanisms linking dysbacteriosis with such CNS-related conditions might include inflammatory or neuroimmune regulation, supporting the gut-brain axis in which inflammatory or immune regulation of the gastrointestinal tract could impose a critical impact on the pathological pathway of migraine [Citation44].
Strengths and limitations
A key strength of this MR analysis is its ability to measure exposure through the use of genetic IVs, thereby overcoming problems such as environmental confounding typically encountered in traditional observational studies [Citation21]. Indeed, there has been a link between the gut microbiota and other health problems, including gastrointestinal, metabolic, psychiatric, and autoimmune disorders, all of which may influence the observed relationship with migraine disease [Citation45, Citation46]. Using an MR design, our research largely avoided unmeasured confounding. Second, benefitting from the publicly available large-scale GWAS data, we systematically evaluated the association between gut microbiota and migraine, trying to identify the candidates involved in the development of migraine from nearly 200 taxa.
This study should also pay attention to some limitations. Firstly, in this study, European descent makes up the majority of the participants in the data, which has the limitation of ethnic data, although this will largely avoid the phenomenon caused by population heterogeneity. However, whether the MR analysis results are generally applicable to other races is worthy of further discussion in future research. Secondly, due to the limited number of IVs satisfying the strict threshold (p < 5 × 10−8), we relaxed the p-value (p < 1 × 10−5) between IVs and exposures to obtain more SNPs, which was widely used but it may increase the likelihood that the first assumption of MR is violated [Citation47]. To ensure sufficient strength of the IVs, we excluded SNPs with F < 10. Thirdly, when using multiple IVs to proxy the exposures, we were not able to directly fully mitigate confounding as the specific biological function of the IVs is typically unknown. Reassuringly, through utilizing complementary sensitivity analyses, our results were rigorously quality-controlled and bias was less likely.
Interpretation for the results of the MR analysis
While the current study identifies some causal roles of gut microbiota associated with the risk of migraine, the MR results should be interpreted with caution. One reason is that the two-sample MR only revealed the overall effect of exposure on the outcome, not the direct effect [Citation48]. There is a complex pathway from exposure to outcome and further studies are warranted to explore the possible mechanisms linking gut microbiota to migraine. Therefore, by identifying potential candidate bacterial taxa, our work would inspire researchers to conduct further in-depth investigations so as to decipher the underlying pathways. Another reason is that as mentioned in previous studies, the MR causal estimates should not be regarded as clinical practice outcomes for interventions on risk factors, according to methodological researchers [Citation49]. Instead, researchers are encouraged to derive indicators of disease risk rather than apply MR results directly to provide expected effects for clinical interventions. Accordingly, we conclude that stool testing may be a viable strategy for identifying specific populations with a higher migraine risk based on the results of our study.
Conclusions
In conclusion, it is clear from this MR study that gut microbiota has a potential causal relationship with migraine symptoms and that there is supporting evidence of a causal connection between gut microbiota and increased or decreased risk of migraine. This will have implications for clinicians that early stool testing may provide a viable alternative for migraine disease screening to identify those at high risk for migraine. In addition, modulating gut microbiota might also be a potentially important strategy to prevent migraine disease. Potential mechanisms warrant further investigations.
Authors contributions
XM designed the study, analyzed the data, and wrote the manuscript. JL assisted with the analysis and revision of the manuscript. HY critically read and edited the manuscript. All authors contributed to the article and approved the submitted version.
Ethics statement
We used publicly available summary level data. Therefore, no additional ethical approval is required. Informed consent was provided by all participants in the original GWAS study.
Supplemental Material
Download MS Word (1.4 MB)Acknowledgments
It is with great gratitude that we acknowledge MiBioGen for providing gut microbiota-related GWAS data as well as the participants and investigators of the GWAS Catalog study.
Disclosure statement
Neither the authors nor the researchers have any commercial or financial relations that could be construed as potential conflicts of interest.
Data availability statement
There was no restriction on the use of data in this study because all the data were publicly available.
Additional information
Funding
References
- International Headache Society. Headache classification committee of the international headache society (IHS) the international classification of headache disorders. Cephalalgia. 2018;38(1):1–211.
- GBD 2016 Disease and Injury Incidence and Prevalence Collaborators. Global, regional, and national incidence, prevalence, and years lived with disability for 328 diseases and injuries for 195 countries, 1990–2016: a systematic analysis for the global burden of disease study 2016. Lancet. 2017;390(10100):1211–1259. doi: 10.1016/S0140-6736(17)32154-2.
- Safiri S, Pourfathi H, Eagan A, et al. Global, regional, and national burden of migraine in 204 countries and territories, 1990–2019. Pain. 2022;163(2):e293–e309. doi: 10.1097/j.pain.0000000000002275.
- Selby G, Lance JW. Observations on 500 cases of migraine and allied vascular headache. J Neurol Neurosurg Psychiatry. 1960;23(1):23–32. doi: 10.1136/jnnp.23.1.23.
- Ding JH, Jin Z, Yang XX, et al. Role of gut microbiota via the gut-liver-brain axis in digestive diseases. World J Gastroenterol. 2020;26(40):6141–6162. doi: 10.3748/wjg.v26.i40.6141.
- Banks WA. Evidence for a cholecystokinin gut-brain axis with modulation by bombesin. Peptides. 1980;1(4):347–351. doi: 10.1016/0196-9781(80)90013-3.
- Faraco G, Brea D, Garcia-Bonilla L, et al. Dietary salt promotes neurovascular and cognitive dysfunction through a gut-initiated TH17 response. Nat Neurosci. 2018;21(2):240–249. doi: 10.1038/s41593-017-0059-z.
- Sampson TR, Debelius JW, Thron T, et al. Gut microbiota regulate motor deficits and neuroinflammation in a model of Parkinson’s disease. Cell. 2016;167(6):1469–1480. doi: 10.1016/j.cell.2016.11.018.
- Bhattacharjee S, Lukiw WJ. Alzheimer’s disease and the microbiome. Front Cell Neurosci. 2013;7:153. doi: 10.3389/fncel.2013.00153.
- Borre YE, Moloney RD, Clarke G, et al. The impact of microbiota on brain and behavior: mechanisms & therapeutic potential. Adv Exp Med Biol. 2014;817:373–403. doi: 10.1007/978-1-4939-0897-4_17.
- Konjevod M, Nikolac Perkovic M, Sáiz J, et al. Metabolomics analysis of microbiota-gut-brain axis in neurodegenerative and psychiatric diseases. J Pharm Biomed Anal. 2021;194:113681. doi: 10.1016/j.jpba.2020.113681.
- Kong G, Cao KL, Judd LM, et al. Microbiome profiling reveals gut dysbiosis in a transgenic mouse model of Huntington’s disease. Neurobiol Dis. 2020;135:104268. doi: 10.1016/j.nbd.2018.09.001.
- Wasser CI, Mercieca EC, Kong G, et al. Gut dysbiosis in Huntington’s disease: associations among gut microbiota, cognitive performance and clinical outcomes. Brain Commun. 2020;2(2):fcaa110. doi: 10.1093/braincomms/fcaa110.
- Zhang YG, Wu S, Yi J, et al. Target intestinal microbiota to alleviate disease progression in amyotrophic lateral sclerosis. Clin Ther. 2017;39(2):322–336. doi: 10.1016/j.clinthera.2016.12.014.
- Scheperjans F, Aho V, Pereira PA, et al. Gut microbiota are related to Parkinson’s disease and clinical phenotype. Mov Disord. 2015;30(3):350–358. doi: 10.1002/mds.26069.
- Milosevic I, Vujovic A, Barac A, et al. Gut-Liver axis, gut microbiota, and its modulation in the management of liver diseases: a review of the literature. Int J Mol Sci. 2019;20(2):395. doi: 10.3390/ijms20020395.
- Dai YJ, Wang HY, Wang XJ, et al. Potential beneficial effects of probiotics on human migraine headache: a literature review. Pain Phys. 2017;2(20;2):E251–e5. doi: 10.36076/ppj.2017.E255.
- Sekula P, Del Greco MF, Pattaro C, et al. Mendelian randomization as an approach to assess causality using observational data. J Am Soc Nephrol. 2016;27(11):3253–3265. doi: 10.1681/ASN.2016010098.
- Smith GD, Ebrahim S. ‘Mendelian randomization’: can genetic epidemiology contribute to understanding environmental determinants of disease? Int J Epidemiol. 2003;32(1):1–22. doi: 10.1093/ije/dyg070.
- Davey Smith G, Hemani G. Mendelian randomization: genetic anchors for causal inference in epidemiological studies. Hum Mol Genet. 2014;23(R1):R89–R98. doi: 10.1093/hmg/ddu328.
- Sanderson E, Smith GD, Windmeijer F, et al. Corrigendum to: an examination of multivariable mendelian randomization in the single-sample and two-sample summary data settings. Int J Epidemiol. 2020;49(3):1057–1057. doi: 10.1093/ije/dyaa101.
- Cai J, Li X, Wu S, et al. Assessing the causal association between human blood metabolites and the risk of epilepsy. J Transl Med. 2022;20(1):437. doi: 10.1186/s12967-022-03648-5.
- Li P, Wang H, Guo L, et al. Association between gut microbiota and preeclampsia-eclampsia: a two-sample mendelian randomization study. BMC Med. 2022;20(1):443. doi: 10.1186/s12916-022-02657-x.
- Burgess S, Small DS, Thompson SG. A review of instrumental variable estimators for mendelian randomization. Stat Methods Med Res. 2017;26(5):2333–2355. doi: 10.1177/0962280215597579.
- Kurilshikov A, Medina-Gomez C, Bacigalupe R, et al. Large-scale association analyses identify host factors influencing human gut microbiome composition. Nat Genet. 2021;53(2):156–165. doi: 10.1038/s41588-020-00763-1.
- Gormley P, Anttila V, Winsvold BS, et al. Meta-analysis of 375,000 individuals identifies 38 susceptibility loci for migraine. Nat Genet. 2016;48(8):856–866. doi: 10.1038/ng.3598.
- Wei Z, Yang B, Tang T, et al. Gut microbiota and risk of five common cancers: a univariable and multivariable mendelian randomization study. Cancer Med. 2023;12(9):10393–10405. doi: 10.1002/cam4.5772.
- Pierce BL, Ahsan H, Vanderweele TJ. Power and instrument strength requirements for mendelian randomization studies using multiple genetic variants. Int J Epidemiol. 2011;40(3):740–752. doi: 10.1093/ije/dyq151.
- Pierce BL, Burgess S. Efficient design for mendelian randomization studies: subsample and 2-sample instrumental variable estimators. Am J Epidemiol. 2013;178(7):1177–1184. doi: 10.1093/aje/kwt084.
- Burgess S, Foley CN, Allara E, et al. A robust and efficient method for mendelian randomization with hundreds of genetic variants. Nat Commun. 2020;11(1):376. doi: 10.1038/s41467-019-14156-4.
- Burgess S, Bowden J, Fall T, et al. Sensitivity analyses for robust causal inference from mendelian randomization analyses with multiple genetic variants. Epidemiology. 2017;28(1):30–42. doi: 10.1097/EDE.0000000000000559.
- Greco MF, Minelli C, Sheehan NA, et al. Detecting pleiotropy in mendelian randomisation studies with summary data and a continuous outcome. Stat Med. 2015;34(21):2926–2940. doi: 10.1002/sim.6522.
- Bowden J, Davey Smith G, Burgess S. Mendelian randomization with invalid instruments: effect estimation and bias detection through egger regression. Int J Epidemiol. 2015;44(2):512–525. doi: 10.1093/ije/dyv080.
- Chen Y, Zhou J, Wang L. Role and mechanism of gut microbiota in human disease. Front Cell Infect Microbiol. 2021;11:625913. doi: 10.3389/fcimb.2021.625913.
- Morais LH, Schreiber H, Mazmanian SK. The gut microbiota-brain axis in behaviour and brain disorders. Nat Rev Microbiol. 2021;19(4):241–255. doi: 10.1038/s41579-020-00460-0.
- Thaiss CA, Zmora N, Levy M, et al. The microbiome and innate immunity. Nature. 2016;535(7610):65–74. doi: 10.1038/nature18847.
- Gao J, Xu K, Liu H, et al. Impact of the gut microbiota on intestinal immunity mediated by tryptophan metabolism. Front Cell Infect Microbiol. 2018;8:13. doi: 10.3389/fcimb.2018.00013.
- Ghavami A, Khorvash F, Heidari Z, et al. Effect of synbiotic supplementation on migraine characteristics and inflammatory biomarkers in women with migraine: results of a randomized controlled trial. Pharmacol Res. 2021;169:105668. doi: 10.1016/j.phrs.2021.105668.
- de Roos NM, van Hemert S, Rovers JMP, et al. The effects of a multispecies probiotic on migraine and markers of intestinal permeability-results of a randomized placebo-controlled study. Eur J Clin Nutr. 2017;71(12):1455–1462. doi: 10.1038/ejcn.2017.57.
- Karlsson FH, Tremaroli V, Nookaew I, et al. Gut metagenome in European women with normal, impaired and diabetic glucose control. Nature. 2013;498(7452):99–103. doi: 10.1038/nature12198.
- Goodrich JK, Waters JL, Poole AC, et al. Human genetics shape the gut microbiome. Cell. 2014;159(4):789–799. doi: 10.1016/j.cell.2014.09.053.
- Yücel M, Kotan D, Gurol Çiftçi G, et al. Serum levels of endocan, claudin-5 and cytokines in migraine. Eur Rev Med Pharmacol Sci. 2016;20(5):930–936.
- Martami F, Razeghi Jahromi S, Togha M, et al. The serum level of inflammatory markers in chronic and episodic migraine: a case-control study. Neurol Sci. 2018;39(10):1741–1749. doi: 10.1007/s10072-018-3493-0.
- Cámara-Lemarroy CR, Rodriguez-Gutierrez R, Monreal-Robles R, et al. Gastrointestinal disorders associated with migraine: a comprehensive review. World J Gastroenterol. 2016;22(36):8149–8160. doi: 10.3748/wjg.v22.i36.8149.
- McLean MH, Dieguez D, Jr., Miller LM, et al. Does the microbiota play a role in the pathogenesis of autoimmune diseases? Gut. 2015;64(2):332–341. doi: 10.1136/gutjnl-2014-308514.
- de Vos WM, de Vos EA. Role of the intestinal microbiome in health and disease: from correlation to causation. Nutr Rev. 2012;70(Suppl 1):S45–S56. doi: 10.1111/j.1753-4887.2012.00505.x.
- Cai J, He L, Wang H, et al. Genetic liability for prescription opioid use and risk of cardiovascular diseases: a multivariable mendelian randomization study. Addiction. 2022;117(5):1382–1391. doi: 10.1111/add.15767.
- Sanderson E. Multivariable mendelian randomization and mediation. Cold Spring Harb Perspect Med. 2021;11(2):a038984. doi: 10.1101/cshperspect.a038984.
- VanderWeele TJ, Tchetgen Tchetgen EJ, Cornelis M, et al. Methodological challenges in mendelian randomization. Epidemiology. 2014;25(3):427–435. doi: 10.1097/EDE.0000000000000081.