Abstract
The interactions of zinc (Zn) and cadmium (Cd) in uptake and translocation are common but not consistent. We hypothesized that Cd2+ and Zn2+ activity in the apoplasmic solution bathing root-cells could affect Zn accumulation in plants dependent on the wheat genotype. This hypothesis was tested using seedlings of two bread wheat genotypes (Triticum aestivum L. cvs. Rushan and Cross) and one durum wheat genotype (Triticum durum L. cv. Arya) with different Zn efficiencies grown in chelate-buffered nutrient solutions with three Zn2+ (10−11.11, 10−9.11, and 10−8.81 µM) and two Cd2+ (10−11.21 and 10−10.2 µM) activity levels. Increasing Zn2+ activity in the nutrient solution significantly increased Zn concentration in root and shoots of all three wheat genotypes, although the magnitude of this increase was dependent on the genotype. Cadmium decreased Zn concentration in roots of “Cross” while it had no significant effect on root Zn concentration in “Rushan.” At Zn2+ = 10−11.11 µM, Cd decreased shoot Zn concentration in “Arya” whereas it increased shoot Zn concentration at Zn2+ = 10−8.81 µM. Cadmium increased shoot Zn concentration of “Rushan” and “Cross” at Zn2+ = 10−8.81 µM but it had no significant effect on shoot Zn concentration of these genotypes at Zn2+ = 10−11.11 µM. The zinc-inefficient genotype “Arya” accumulated significantly more Cd in its root in comparison with “Cross” and “Rushan.” Cadmium concentration in roots of “Arya” was decreased significantly with increasing Zn activity. The effect of Zn on accumulation of Cd in roots of “Cross” and “Rushan” was dependent on the dose provided, and therefore, both synergistic (at Zn2+ = 10−9.11 µM) and antagonistic (at Zn2+ = 10−8.81 µM) interactive effects were found in these genotypes. Zinc supply increased the Zn concentration of xylem sap in “Cross” and “Rushan” whereas Zn content in xylem sap of “Arya” was decreased at Zn2+ = 10−9.11 µM and thereafter increased at Zn2+ = 10−8.81 µM. Cadmium treatment reduced Zn concentration in xylem sap of “Arya,” while it tended to increase Zn content in xylem sap of “Cross.” At Zn-deficient conditions, greater retention of Zn in root cell walls of Zn-inefficient “Arya” resulted in lower root-to-shoot transport of Zn in this genotype. Results revealed that the effect of Cd on the root-to-shoot translocation of Zn via the xylem is dependent on wheat genotype and Zn activity in the nutrient solution.
Introduction
Zinc (Zn) is an essential micronutrient that plays numerous roles in the physiology and biochemistry of higher plants (Marschner Citation1995). Zn deficiency is common in wheat-growing areas of the world, particularly in calcareous soils (Welch et al. Citation1991; Takkar and Walker Citation1993; White and Zasoski Citation1999).
On the other hand, cadmium (Cd) is one of the most toxic heavy metals in the environment due to its high mobility and severe toxicity to plants (Norvell et al. Citation2000). Although plants do not require Cd2+ for growth and reproduction, the bioaccumulation index of Cd2+ in plants is high and may exceed that of many essential elements (Brown et al. Citation1990). Anthropogenic inputs of Cd to soils occur via mining, atmospheric depositions, and use of fertilizers, manures, municipal sewage-wastes, compost and industrial sludge (Alloway and Steinnes Citation1999).
Cadmium and Zn are elements having similar geochemical and environmental properties (Hart et al. Citation2002). Therefore, significant interactions occur between Cd and Zn in their accumulation by roots and translocation from roots to the arial parts of plants.
The effects of interactions between Zn and Cd on uptake and distribution in crops are relevant for plant nutrition as well as for the quality of plant foods. Many studies have reported on the effect of Zn2+ on the uptake and translocation of Cd2+ in some crops, such as wheat (Triticum aestivum L.) (Choudhary et al. Citation1994), bean (Phaseolus vulgaris L.) (Chaoui et al. Citation1997), and lettuce (Lactuca sativa L.) (McKenna et al. Citation1993). Increasing the supply of Zn in the growth media may reduce the accumulation of Cd in plants, particularly in Zn deficient conditions (Choudhary et al. Citation1994; Oliver et al. Citation1994). Insufficient solution Zn2+ activity may be an important factor in enhanced Cd accumulation by plants even when Zn is applied at sufficient levels to prevent Zn deficiency. Under insufficient Zn2+ activity the ability to adequately regulate ion transport processes of plasma membranes is reduced (Norvell and Welch Citation1993; Welch and Norvell Citation1993). Impaired integrity of root plasma membrane may be accompanied by an increase in Cd uptake.
On the other hand, an increase in the plant Zn uptake in the presence of Cd has been reported (Hinsley et al. Citation1984; Sharma and Agrawal Citation2006). These contradictory results may be attributed to differences among the crop species or cultivars, interactions between cultivars and metals, and differences of plant tissues in their tendency to accumulate the metals (Brown et al. Citation1972). Thus, further studies are needed to investigate the interactions between Zn and Cd in order to encourage selection for enhanced uptake efficiency of the desirable element Zn, and reduced uptake of the undesirable metal Cd.
Wheat genotypes differ widely in their ability to absorb, accumulate, and distribute Zn and Cd (Cakmak et al. Citation1996, Citation1997; Kalayci et al. Citation1999). These differences among wheat genotypes in the tissue accumulation and partitioning of Zn and Cd may be associated with their Zn efficiency.
We hypothesized that inadequate Zn2+ activity (estimated to be 10−10 to 10−11 M Zn2+) in the apoplasmic solution bathing root-cells could affect Cd accumulation in plants dependent on the wheat genotype. We tested this hypothesis using seedlings of three wheat genotypes with different Zn efficiencies grown in the chelate-buffered nutrient solutions and supplied Cd2+ at a level typically found in agricultural high-Cd soils in central Iran.
Materials and Methods
Solution Composition and Plant Growth Conditions
The experiment was arranged as a completely randomized factorial design with three levels of Zn in the nutrient solution and two levels of Cd. Zinc was added as zinc sulphate (ZnSO4) at concentrations of 0, 10, and 20 µM and corresponding activities (Zn2+) 10−11.11, 10−9.11 and 10−8.81. Cadmium sulphate (CdSO4) was added to achieve concentrations of 0 and 1 µM of total Cd in the nutrient solution with the corresponding free metal activities (Cd2+) 10−11.21 and 10−10.2, respectively. Each Cd-Zn treatment had four replicates. Free metal activities and solution speciation were calculated using Visual MINTEQ08 (Gustafsson Citation2007). The nutrient solution contained, in mM, KNO3, 2.5; Ca(NO3)2, 2.5; MgSO4, 1; KH2PO4, 0.1; in µM, KCl, 75; H3BO3, 10; Na2MoO4, 0.2; FeEDTA, 20; MnSO4, 2; CuSO4, 5; NiCl2, 1 and 2 mM MES [2-(N-morpholino) ethanesulfonic acid] buffer (pH 6.0). The chelating agent, EDTA, was added to supply 50 µM in excess of the sum of manganese (Mn), copper (Cu), nickel (Ni), Cd, and Zn concentrations to buffer microelement cation activities (Chaney Citation1988).
Seeds of two bread wheat genotypes (Triticum aestivum L. cvs. Rushan and Cross) and a durum wheat genotype (Triticum durum L. cv. Arya) were germinated in quartz sand moistened with distilled water. After 7 days, sixteen seedlings per replicate were transferred to 8-L polyethylene beakers; they were continuously aerated and solution pH was monitored on a daily basis and adjusted to 5.6 with KOH or HCl. Cadmium and Zn treatments were imposed on the eighth day following seed imbibition, one day after transferring the seedlings to the solution culture. Nutrient solutions were replaced twice a week. The plants were kept under controlled conditions with an 8-h light period at a light intensity of 390 µmol m2−s−1, 25/20 °C day/night temperature, and 65–75% relative humidity. Plants were harvested after 60 days and washed with deionized water. The roots and shoots were separated for analysis. Plant samples were dried for 48 h at 70°C, weighed, and digested by microwave (USEPA Citation1995). About 0.500 g of plant samples were digested in APCU-40 75 mL TFM Teflon microwave vessels (Milestone Srl, START D, Sorisole, Italy) using 5 mL of HNO3 and 3 mL of H2O2, then were filtered through Whatman no. 42 filters, transferred to 50-mL volumetric flasks, and diluted with deionized water.
For quality control, reagent blanks and NIST Standard Reference Materials (NIST No. 1515 apple leaves) were included. Concentrations of Zn and Cd in the digest solutions were determined by atomic absorption spectrometry (AAS) (PerkinElmer 800, PerkinElmer, Wellesley, MA) (Chapman and Pratt Citation1961). All plant tissue concentrations are expressed on a dry weight basis.
Xylem Sap Analysis
Two weeks after transferring the seedlings to the nutrient solution, one set of plants was decapitated with a razor blade just below the first leaf node. A silicon tube was inserted over the decapitated stem and sealed, and xylem sap exuded over a 24-h period was collected and analyzed via AAS for metal concentrations.
Zn concentration in the root cell wall
Roots (0.6 g) were homogenized with 1 mm MES (pH 6.0) and centrifuged for 10 min at 3000 g to precipitate cell walls. The Zn concentration of cell walls was determined by AAS.
Statistical analysis
The experiment was set up according to a completely randomized factorial design; each treatment contained four replicates. Mean separations were performed by Fisher's protected Least Significant Difference (LSD) method at a significance level of 0.05 (SAS Institute Citation2000).
Results
Growth effect
presents data showing the effects of increasing Zn supply (Zn2+ = 10−11.11, 10−9.11 and 10−8.81 µM) on the root and shoot dry weights of wheat seedlings grown in nutrient solution with or without Cd. The effect of Zn nutrition on the shoot growth was dependent on the wheat genotype and the supplied Zn and Cd level (). The greatest shoot dry weight of all three wheat genotypes was produced at the 10−8.81 µM Zn2+ level. The Zn2+ level of 10−9.11 µM in the nutrient solution was ineffective in increasing the shoot dry weight of all wheat genotypes except “Rushan.”
Table 1. The effect of varied buffered zinc (Zn2+) and cadmium (Cd2+) activities on the shoot and root dry weight (g pot−1) of wheat genotypes*
Application of 1 µM Cd (Cd2+ = 10−10.2 µM) in the nutrient solution produced a significant growth inhibition of “Rushan” wheat plants measured as dry weight of roots and shoots (). However, Cd caused a significant decrease in the root dry matter yield of “Cross” only at the Zn2+ = 10−11.11 µM level. The shoot growth of “Cross” plants was not affected by Cd. In “Arya,” Cd effect on the plant growth was dependent on the Zn level in the nutrient solution. In the Zn-free solution, Cd at 1 µM increased the shoot dry matter weights of “Arya” while it had no effect on or decreased plant growth with Zn treatments.
Zn concentration in roots and shoots of wheat
Increasing Zn2+ activity in the nutrient solution from 10−11.11 µM to 10−8.81 µM resulted in a significant increase in Zn concentration in the root () and shoots () of all three wheat genotypes, although the magnitude of this increase was dependent on the genotype. In general, “Arya” and “Cross” accumulated the greatest and smallest amounts of Zn in their roots, respectively (). The greatest shoot Zn concentration was found in “Rushan,” while “Arya” had the smallest shoot Zn concentration ().
Figure 1. The effect of varied buffered zinc (Zn2+) and cadmium (Cd2+) activities on Zn concentration in the root of wheat genotypes. Same letters above bars indicate no significant difference at P < 0.05. Zn0, Zn10, and Zn20 present free Zn2+activities 10−11.11, 10−9.11, and 10−8.81 µM, respectively. Cd0 and Cd1 present free Cd2+ activities 10−11.21 and 10−10.2 µM, respectively.
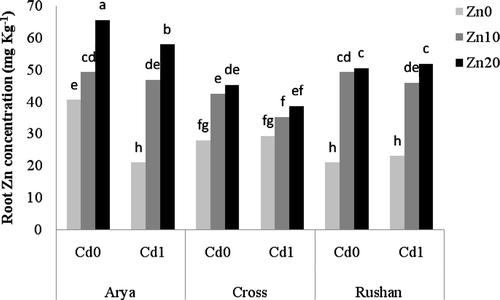
Figure 2. The effect of varied buffered zinc (Zn2+) and cadmium (Cd2+) activities on Zn concentration in the shoot of wheat genotypes. Same letters above bars indicate no significant difference at P < 0.05. Zn0, Zn10, and Zn20 present free Zn2+ activities 10−11.11, 10−9.11, and 10−8.81 µM, respectively. Cd0 and Cd1 present free Cd2+ activities 10−11.21 and 10−10.2 µM, respectively.
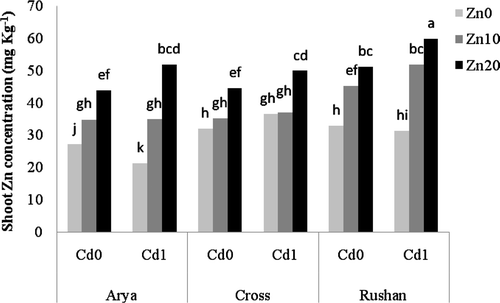
The effect of Cd on Zn concentration varied depending upon the wheat genotype and plant tissue ( and ). Cadmium treatment decreased the concentration of Zn in roots of “Cross” while it had no significant effect on the root Zn concentration in “Rushan.” The effect of Cd addition on Zn concentration in the shoot of “Arya” was dependent on the supplied Zn level. At pZn2+ = 11.11, Cd decreased shoot Zn concentration whereas it had no significant effect and increased shoot Zn concentration at Zn2+ = 10−9.11 µM and Zn2+ = 10−8.81 µM, respectively. Cadmium increased shoot Zn concentration of “Rushan” and “Cross” at Zn2+ = 10−8.81 µM but it had no significant effect on shoot Zn concentration of these genotypes at Zn2+ = 10−9.11 µM ().
Cd concentration in roots and shoots of wheat
Cadmium supply resulted in accumulation of Cd in roots of all wheat genotypes although the magnitude of this increase varied among the genotypes (). “Arya” accumulated significantly greater Cd in its root in comparison with “Cross” and “Rushan.”
Figure 3. The effect of varied buffered zinc (Zn2+) activities on cadmium (Cd) concentration in the root of wheat genotypes. Same letters above bars indicate no significant difference at P < 0.05. Zn0, Zn10, and Zn20 present free Zn2+activities 10−11.11, 10−9.11, and 10−8.81 µM, respectively. Cd0 and Cd1 present free Cd2+ activities 10−11.21 and 10−10.2 µM, respectively. Cadmium concentration in the shoot was less than 0.02 mg kg−1.
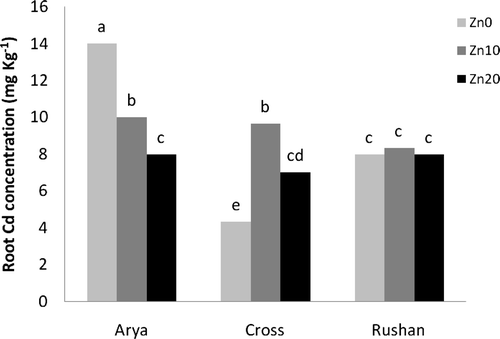
The effect of Zn supply on the tissue Cd concentration varied among the examined wheat genotypes (). Cadmium concentration in the root of “Arya” decreased significantly with increasing Zn activity. The effect of Zn on the accumulation of Cd in roots of “Cross” was dependent on the dose provided, and thereby both synergistic (at Zn2+ = 10−9.11 µM) and antagonistic (at Zn2+ = 10−8.81 µM) interactive effects were found in these genotypes (). Zinc supply had no significant effect on root Cd concentration in “Rushan.” Irrespective of the wheat genotype, Cd concentration in the shoot was less than 0.02 mg kg−1. This shows very limited transport of Cd from the root to the shoot.
Zn concentration in the xylem sap
Concentration of Zn in the xylem sap varied among the studied wheat genotypes (). “Rushan” had the greatest Zn concentration its xylem sap. In “Cross” and “Rushan,” increasing Zn supply caused an increase in Zn concentration of xylem sap, whereas the Zn content in xylem sap of “Arya” was decreased at the Zn2+ = 10−9.11 µM level and thereafter increased at the Zn2+ = 10−8.81 µM level. The effect of Cd on Zn concentration in xylem sap was dependent upon the wheat genotype (). Cadmium treatment reduced Zn concentration in xylem sap of “Arya,” while it tended to increase Zn content in xylem sap of “Cross.”
Figure 4. The effect of varied buffered zinc (Zn2+) and cadmium (Cd2+) activities on Zn concentration in xylem sap of wheat genotypes. Same letters above bars indicate no significant difference at P < 0.05. Zn0, Zn10, and Zn20 present free Zn2+activities 10−11.11, 10−9.11, and 10−8.81 µM, respectively. Cd0 and Cd1 present free Cd2+ activities 10−11.21 and 10−10.2 µM, respectively.
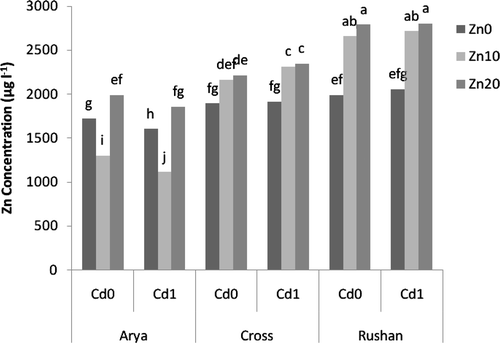
Zn concentration in the root cell wall
The studied wheat genotypes differed in the Zn concentration of the root cell wall (). “Arya” had the greatest Zn concentration in the root cell wall in comparison with the other wheat genotypes. The effect of Zn nutrition on Zn concentration of the root cell wall was dependent on the wheat genotype. Increasing Zn concentration in the nutrient solution significantly increased Zn concentration in the root cell wall of “Cross.” In “Arya,” the concentration of Zn in the root cell wall was increased at Zn2+ = 10−9.11 µM and then decreased with increasing Zn2+ to 10−8.81 µM. When compared to “Arya” and “Cross,” Zn concentration in the root cell wall of “Rushan” showed a significant decrease with increasing Zn2+ activity. As shown in , irrespective of the wheat genotype, Zn concentration in the root cell wall was generally increased by the supply of Cd.
Figure 5. The effect of varied buffered zinc (Zn2+) and cadmium (Cd2+) activities on Zn concentration in root cell wall of wheat genotypes. Same letters above bars indicate no significant difference at P < 0.05. Zn0, Zn10, and Zn20 present free Zn2+activities 10−11.11, 10−9.11, and 10−8.81 µM, respectively. Cd0 and Cd1 present free Cd2+ activities 10−11.21 and 10−10.2 µM, respectively.
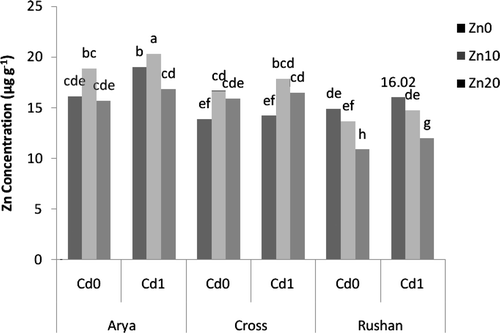
Discussion
Zinc uptake by the root and its translocation to the shoot in Zn-efficient and Zn-inefficient wheat genotypes has been investigated previously (Erenoglu et al. Citation1999). Generally, it has been reported that Zn-efficient genotypes have a greater ability for Zn uptake in comparison with Zn-inefficient genotypes (Khoshgoftar et al. Citation2006). However, the results of the current study showed that in general the uptake of Zn by the roots of “Arya,” as a Zn-inefficient genotype, was higher than that in the Zn-efficient “Rushan” and “Cross” genotypes, suggesting that Zn uptake does not confer Zn efficiency in wheat ( and ).
In line with our results, Hacisalihoglu et al. (Citation2001) reported that the uptake of Zn by roots was similar among the wheat genotypes differing in Zn efficiency and concluded that Zn uptake has no relationship with Zn efficiency in wheat. An important point is that in most studies, the sum of apoplasmic and symplasmic Zn has been measured as the root metal uptake while this is actually pseudo root uptake. Some cations can physically adsorb on the negatively charged surfaces of the Donnan space of root cell apoplasm. In most cases, retention of metal cations by the apoplasm causes a significant reduction of the influx of metal into the cell cytoplasm and its further transport to the shoots (Marschner Citation1995). Accordingly, the apoplasmic Zn was greater in “Arya” in comparison with two other wheat genotypes (data not shown).
Alternatively, Zn efficiency mechanisms might be related to the mobility and distribution of Zn within the shoot (Hacisalihoglu et al. Citation2001). The results of the present study indicated that at the low Zn level (Zn2+ = 10−11.11 µM), shoot Zn concentration in the Zn-inefficient “Arya” was lower than that in the Zn-efficient “Cross” and “Rushan.” This is probably due to greater retention of Zn in roots and lower transport of Zn to shoots in “Arya.” However, in the nutrient solutions containing Zn, shoot Zn concentrations were similar between “Cross” and “Arya” (). Among the wheat genotypes, “Rushan” contained greater Zn in its xylem sap. Accordingly, “Rushan” had higher shoot Zn concentration compared to the other wheat genotypes, which suggests that most of the Zn2+ is available in the xylem sap of this genotype, as shown in .
In “Arya,” the lowest concentration of Zn (in the xylem sap) was determined at Zn2+ = 10−8.81 µM, while the greatest content of Zn (in the cell wall) was recorded in the Zn activity level of the nutrient solution. These results suggest that the greater ratio of Zn retained in the root cell wall apoplasmic spaces of “Arya” is accompanied by lower transport of Zn from root to shoots of this Zn-inefficient genotype, in comparison to other wheat genotypes. It seems that at the Zn deficient condition, a higher ratio of apoplasmic to symplasmic Zn and greater translocation of Zn to the shoot are involved in the higher Zn concentration in the xylem sap of “Arya” at Zn2+ = 10−11.11 µM, in comparison with Zn2+ = 10−8.81 µM. Another possible reason for this phenomenon is greater movement of Zn from the xylem to the phloem within the central stele. It has been reported that transport of Zn and Cd within the plants appears to be largely phloem-mediated (Haslett et al. Citation2001). The ability of the plant to control movement of Zn from the xylem into the phloem is largely dependent on the crop genotype and Zn concentration in the media (Haslett et al. Citation2001). Further research is needed to fully clarify the reason for lower Zn concentration in the xylem sap of “Arya” at Zn2+ = 10−8.81 µM in comparison with Zn2+ = 10−11.11 µM.
In this study, we investigated the interactions of Zn and Cd in uptake and translocation associated with the differential response of wheat genotypes to Zn deficiency stress. Interaction effects of Zn and Cd on plant metal accumulation are common but not consistent. Most of the findings show that Zn reduces the uptake of Cd by both root and shoots (Adriano Citation1986). In contrast, some authors have suggested that the interactive pattern is synergistic and Cd toxicity is enhanced by the addition of Zn (Nan et al. Citation2002; Wu and Zhang Citation2002). The results from our present study indicate that the effects of interaction between Zn and Cd on uptake by the plant is dependent on the wheat genotype and Zn activity in the nutrient solution.
Cadmium treatment decreased the concentration of Zn in roots of “Arya.” These results are in agreement with the findings of Shute and Macie (Citation2006) who reported a significant decrease in tissue concentration of Zn in the presence of Cd. It has been suggested the presence of Cd2+ affects free cations (Zn2+) through antagonistic processes mediated by competition for either binding sites or transporters (Nicole et al. Citation2000). Wu et al. (Citation2004) showed that 1 and 10 µM levels of Cd reduced Zn concentrations in cotton (Gossypium hirsutum). In contrast to effects in “Arya,” the presence of Cd in the nutrient solution had no significant effect on the root Zn concentration of “Rushan” (). This result is in opposition to another study in which a decrease in Zn accumulation in plant tissues by application of Cd was reported (McLaughlin and Singh Citation1999). The effect of Cd on the uptake of Zn by roots of “Cross” was dependent on the Zn level in the media. Shute and Macie (Citation2006) also reported that the effect of Zn on the accumulation of Cd was dependent on the metal supplied level.
This controversy in the results is partly due to different physiological characteristics of the examined plants. Cadmium and Zn translocation in plants appears to be genotype-specific and most likely related to genotypic differences in long-distance transport processes (Tudoreanu and Phillips Citation2004).
In general, wheat plants grown in the Cd-containing solution accumulated greater amounts of Zn in their shoots in comparison with those grown in the Cd-free nutrient solution (). In agreement with our results, Smilde et al. (Citation1992) concluded that Zn uptake by plants increased with applied Cd. Welch et al. (Citation1999) also found that uptake rates of Cd by roots of durum wheat (Triticum durum L.) increased as solution Zn concentrations increased. In a field experiment, Nan et al. (Citation2002) reported that increasing Cd contents in soil could increase the accumulation of Zn in crops. Liu et al. (Citation2006) reported a positive correlation between Cd and iron (Fe) concentration in both roots and leaves, implying that some synergistic interactions occurred in the absorption and translocation of Cd and Fe. According to Kennedy and Gonsalves (Citation1989), low Cd2+ levels hyperpolarize the plasma membranes at the root surface and thereby increase the transmembrane potential. The transmembrane potential is an energy source for cation uptake. The positive effect of Cd2+ exposure on Fe, and probably Zn, absorption may be partly due to increase in the transmembrane potential.
In “Rushan” and “Cross,” due to a lower capacity of root apoplasm for retaining the metals, it seems that Cd could release Zn from the apoplasmic space of the roots and thereby increase the influx of Zn into the root cell cytoplasm. Accordingly, the application of Cd in the nutrient solution was accompanied by an increase in the root-to-shoot translocation of Zn in “Rushan” and “Cross.” In contrast, a decrease in the root-to-shoot translocation of Zn in “Arya” was found in the presence of Cd. This decrease in root-to-shoot translocation of Zn can be explained by a greater concentration of Zn in the root cell wall and, thus, a reduced Zn content in the xylem sap of “Arya.”
The greater ability of “Arya” to uptake Cd from the growth media is partly related to the greater capacity of its roots for retaining Cd in the apoplasmic spaces. The mechanisms of uptake and translocation of Cd are poorly understood. However, most of the plants retain more than 50% of the absorbed Cd in the roots (Obata and Umebayashi Citation1993). These results agreed with the previous reports of other researchers (Clarke et al. Citation1997a, Citationb; Hocking and McLaughlin Citation2000; Arao and Ishikawa Citation2006) who reported that some crops such as sunflower (Helianthus annuus L.), flax (Linum usitatissimum L.), rice (Oryza sativa L.), and durum wheat have a relatively high ability to accumulate Cd (more than 0.10 mg Cd kg−1 dry matter) in their tissues. In general, roots accumulate the highest proportion of Cd compared to other plant tissues. This is because of precipitation and/or adsorption of Cd on the root surface and within the symplasm of root cells. Cadmium is also sequestered and immobilized by phytochelatins in the vacuoles of roots cells. Tudoreanu and Phillips (Citation2004) suggested that the primary mechanism for intracellular immobilization of Cd is through the formation of Cd phytochelatin complexes, which deposit Cd in vacuoles. In some plants, such as durum wheat genotypes, the synthesis of phytochelatins increases in the apoplasm of root cell walls in the presence of Cd (McLaughlin and Singh Citation1999). In “Arya,” the addition of Cd to the nutrient solution decreased the total Zn concentration in the roots () while it increased the distribution of Zn in the root cell walls. It seems that the presence of Cd stimulated the synthesis of phytochelatins in the roots of “Arya.” Antagonistic effects of Cd on Zn uptake by roots in “Arya” might have resulted from the competitive transport and absorption interaction between these two metal ions (Nan et al. Citation2002). Tudoreanu and Phillips (Citation2004) concluded that Cd ions may compete with other cations (e.g., Na+, Ca2+ and Zn2+) for transmembrane carriers. Some metal transporters belonging to the NRAMP (natural resistance-associated macrophage protein) and ZIP (zinc-regulated transporter/iron-regulated transporter-related protein) families have recently been identified as transporters of Zn2+ as well as of Cd2+ (Nicole et al. Citation2000). Lasat et al. (Citation1996) have cloned a high-affinity Zn transporter (ZNTI) in Thlaspi caerulescens and demonstrated that ZNTI mediates Zn uptake as well as Cd uptake with a much lower affinity.
Our results suggest that the Cd uptake system in Zn-efficient genotypes is different from that in the Zn-inefficient genotypes (Oliver et al. Citation1995; Hart et al. Citation1998). The nature of Cd uptake saturability may be different among wheat genotypes.
As Zn activity increased in the culture solution, the Cd concentration in the root for “Arya” decreased, “Cross” showed an increase and “Rushan” did not change. In “Arya,” Zn could substitute for Cd in the apoplasmic space of roots and thereby decreased root Cd concentration. On the other hand, Cd could reduce Zn uptake by the roots of “Arya.” These results confirm the antagonistic relationship between Cd and Zn for uptake in “Arya.” The antagonistic relationship between Zn and Cd uptake in this wheat genotype suggests that enhanced Zn concentrations in solution inhibit root Cd2+ uptake in Zn-inefficient genotypes, which could be attributed to competition for carriers across the root membrane. In fact, Zn2+ and Cd2+ each inhibit the uptake of the other in roots of wheat (Hart et al. Citation2002) because the affinity of the membrane transporter is similar for the two ions. Some studies have shown that membrane transporter affinity is greater for Cd than for Zn (Homma and Hirata Citation1984; Hart et al. Citation2002).
In “Cross,” the interaction of Zn and Cd was dependent on the applied rate of Zn so that the Zn2+ = 10−9.11 µM treatment synergistic interaction, and the Zn2+ = 10−8.81 µM antagonistic interaction occurred in the absorption of Cd and Zn. The positive effect of Zn2+ at 10−9.11 µM on root Cd uptake seems to be partly due to an increase in hyper-polarization of the plasma membranes at the root surface. At a higher rate of Zn2+ (10−8.81 µM), competition between Zn and Cd for carriers across root membrane caused a reduction in root Cd concentration. In the “Rushan” genotype, increasing the Zn level in the nutrient solution had no effect on Cd uptake by roots. This suggests a different transport system for Cd and Zn in this genotype, or the inability of Zn at such concentrations to compete with Cd for the carriers. It is interesting that while the affinity of the Zn/Cd carrier is quite high for Cd2+, relatively high Zn2+ activities may be required to inhibit Cd2+ uptake (Hacisalihoglu et al. Citation2001; Hart et al. Citation2002). Further research is necessary to elucidate the transport systems of Cd and Zn in different Zn efficiency wheat genotypes.
References
- Adriano , DC . 1986 . Trace Elements in The Terrestrial Environment , New York : Springer-Verlag .
- Alloway , BJ and Steinnes , E . 1999 . “ Anthropogenic additions of cadmium to soils ” . In Cadmium in Soils and Plants , Edited by: McLaughlin , MJ and Singh , BR . 97 – 123 . Dordrecht, Netherlands : Kluwer Academic Publishers .
- Arao , T and Ishikawa , S . 2006 . Genotypic differences in cadmium concentration and distribution of soybeans and rice . Jpn. Agri. Res. Q. , 40 : 21 – 30 .
- Brown , DA , Bay , SM and Hershelman , GP . 1990 . Exposure of scorpionfish (Scorpaena guttata) to cadmium: effects of acute and chronic exposures on the cytosolic distribution of cadmium, copper and zinc . Aquat. Toxicol. , 16 : 295 – 310 .
- Brown , JW , Ambler , JE , Chaney , RL and Foy , CD . 1972 . “ Differential responses of plant genotypes to micronutrients ” . In Micronutrients in Agriculture , Edited by: Mortvedt , JJ , Giordana , PM and Lindsay , WL . 389 – 418 . Madison, , WI : Soil Science Society of America .
- Cakmak , I , Ekiz , H , Yilmaz , A , Torun , B , Koleli , N , Gultekin , I , Alkan , A and Ekern , S . 1997 . Differential response of rye, bread and durum wheats to zinc deficiency in calcareous soils . Plant Soil , 188 : 1 – 10 .
- Cakmak , I , Sari , N , Marschner , H , Ekiz , H , Kalayci , M , Yilmaz , A and Braun , HJ . 1996 . Phytosiderophore release in bread and durum wheat genotypes differing in zinc efficiency . Plant Soil , 180 : 183 – 189 .
- Chaney , RL . 1988 . “ Metal speciation and interaction among elements affect trace element transfer in agricultural and environmental food-chains ” . In Metal Precipitation: Theory, Analysis, and Application , Edited by: Kramer , JR and Allen , HE . 219 – 259 . Boca Raton, , FL : Lewis Publications .
- Chaoui , A , Ghorbal , MH and Ferjani , EE . 1997 . Effects of cadmium-zinc interactions on hydroponically grown bean (Phaseolus vulgaris L.) . Plant Sci. , 126 : 21 – 28 .
- Chapman , HD and Pratt , PF . 1961 . Methods of Analysis for Soil, Plant and Water , California : Division of Agriculture Science, California University, Riverside .
- Choudhary , M , Bailey , LD and Grant , CA . 1994 . Effect of zinc on cadmium concentration in the tissue of durum wheat . Canadian J. Plant Sci. , 74 : 549 – 552 .
- Clarke , JM , Leisle , D , DePauw , RM and Thiessen , LL . 1997a . Registration of five pairs of durum wheat genetic stocks near-isogenic for cadmium concentration . Crop Sci. , 37 : 297
- Clarke , JM , Leisle , D and Kopytko , GL . 1997b . Inheritance of cadmium concentration in five durum wheat crosses . Crop Sci. , 37 : 1722 – 1726 .
- Erenoglu , B , Cakmak , I , Romheld , V , Derici , R and Rengel , Z . 1999 . Uptake of zinc by rye, bread wheat and durum wheat cultivars differing in zinc efficiency . Plant Soil , 209 : 245 – 252 .
- Gustafsson , JP . 2007: Visual MINTEQ. http://www.lwr.kth.se/ English/OurSoftware/vminteq/ index.htm (January 2007)
- Hacisalihoglu , G , Hart , JJ and Kochian , LV . 2001 . High- and low-affinity zinc transport systems and their possible role in zinc efficiency in bread wheat . Plant Physiol. , 131 : 595 – 602 .
- Hart , JJ , Norvell , WA , Welch , RM , Sullivan , LA and Kochian , LV . 1998 . Characterization of zinc uptake, inducing, and translocation in intact seedlings of bread and durum wheat cultivars . Plant Physiol. , 118 : 219 – 226 .
- Hart , JJ , Welch , RM , Norvell , WA and Kochian , LV . 2002 . Transport interactions between cadmium and zinc in roots of bread and durum wheat seedlings . Physiol. Plant. , 116 : 73 – 78 .
- Haslett , BS , Reid , RJ and Rengel , Z . 2001 . Zinc mobility in wheat: uptake and distribution of zinc applied to leaves or roots . Ann. Bot. , 87 : 379 – 386 .
- Hinsley , TD , Redborg , KE , Pietz , RC and Ziegler , EL . 1984 . Cadmium and zinc uptake by corn (Zea mays L.) with repeated applications of sewage sludge . J. Agr. Food Chem. , 32 : 155 – 163 .
- Hocking , PJ and McLaughlin , MJ . 2000 . Genotypic variation in cadmium accumulation by seed of linseed, and comparison with seeds of some other crop species . Aust. J. Agric. Res. , 51 : 427 – 33 .
- Homma , Y and Hirata , H . 1984 . Kinetics of cadmium and zinc absorption by rice seedling roots . Soil Sci. Plant Nutr. , 30 : 527 – 532 .
- Kalayci , M , Torun , B , Eker , S , Aydin , M , Ozturk , L and Cakmak , I . 1999 . Grain yield, zinc efficiency and zinc concentration of wheat cultivation grown in a zinc-deficient calcareous soil in field and greenhouse . Field Crops Res. , 63 : 87 – 98 .
- Kennedy , CD and Gonsalves , FAN . 1989 . The action of divalent Zn, Cd, Hg, Cu and Pb ions on the ATPase activity of a plasma membrane fraction isolated from roots of Zea mays . Plant Soil , 117 : 167 – 175 .
- Khoshgoftar , AH , Shariatmadari , H and Karimian , N . 2006 . Responses of wheat genotypes to zinc fertilization under saline soil conditions . J. Plant Nutr. , 27 : 1 – 14 .
- Lasat , MM , Baker , AJM and Kochian , LV . 1996 . Physiological characterization of root Zn2+ absorption and translocation to shoots in Zn hyperaccumulator and nonaccumulator species of Thlaspi . Plant Physiol. , 112 : 1715 – 1722 .
- Liu , DH , Wang , M , Zou , JH and Jiang , WS . 2006 . Uptake and accumulation of cadmium and some nutrient ions by roots and shoots of maize (Zea mays L.) . J. Bot. , 38 : 701 – 709 .
- Marschner , H . 1995 . Mineral Nutrition of Higher Plants, , 2nd , London : Academic Press .
- McKenna , I , Chaney , R and Williams , FM . 1993 . The effects of cadmium and zinc interactions on the accumulation and tissue distribution of zinc and cadmium in lettuce and spinach . Environ. Pollut. , 79 : 113 – 120 .
- McLaughlin , MJ and Singh , BR . 1999 . “ Cadmium in soils and plants ” . In Cadmium in soils and plants , Edited by: McLaughlin , MJ and Singh , BR . 257 – 267 . Dordrecht, Netherlands : Kluwer Academic Publishers .
- Nan , ZR , Li , JJ , Zhang , JM and Cheng , GD . 2002 . Cadmium and zinc interactions and their transfer in soil-plant system under actual field conditions . Sci. Total Environ. , 285 : 187 – 195 .
- Nicole , SP , Larsen , PB , Stephen , D , Deborah , E , Letham , LD , Lasat , MM , Garvin , DF , Eide , D and Kochian , LV . 2000 . The molecular physiology of heavy metal transport in the Zn/Cd hyperaccumulator Thlaspi caerulescens . Proc. Natl. Acad. Sci. USA. , 97(9) : 4956 – 4960 .
- Norvell , WA and Welch , RM . 1993 . Growth and nutrient uptake by barley (Hordeum vulgare L. cv. Herta): Studies using an N-(2-hydroxyethyl) ethylenedinitrilotriacetic acid-buffered nutrient solution technique. I. Zinc ion requirements . Plant Physiol. , 101 : 619 – 625 .
- Norvell , WA , Wu , J , Hopkins , DG and Welch , RM . 2000 . Association of cadmium in durum wheat grain with soil chloride and chelate-extractable soil cadmium . Soil Sci. Soc. Am. J. , 64 : 2162 – 2168 .
- Obata , H and Umebayashi , M . 1993 . Production of SH compounds in higher plants of different tolerance to Cd . Plant Soil , 155/156 : 533 – 536 .
- Oliver , DP , Hannam , R , Tiller , KG and Wilhelm , NS . 1994 . The effects of zinc fertilization on cadmium concentration in wheat grain . J. Environ. Qual. , 23 : 705 – 711 .
- Oliver , DP , McFarlane , J , Ascher , J , Kidd , C , Cozens , G and Tiller , K . 1995: The effect of manganese and copper nutrition on cadmium concentration in wheat grain [Restricted]. Technical Report. Division of Soils. CSIRO Australia (6/1/1995)
- Institute , SAS . 2000: The SAS System for Windows, Release 8.0. Statistical Analysis Systems Institute, Carry, NC
- Sharma , RK and Agrawal , M . 2006 . Effects of single and combined treatment of Cd and Zn on carrots: uptake and bioaccumulation . J. Plant Nutr. , 29 : 1791 – 1804 .
- Shute , T and Macie , SM . 2006 . Cadmium and zinc accumulation in soybean: A threat to food safety? . Sci. Total Environ. , 37 : 63 – 73 .
- Smilde , KW , Luit , BV and Driel , WV . 1992 . The extraction by soil and absorption by plants of applied zinc and cadmium . Plant Soil , 143 : 233 – 238 .
- Takkar , PN and Walker , CD . 1993 . “ The distribution and correction of zinc deficiency ” . In Zinc in Soils and Plants , Edited by: Robson , AD . 151 – 166 . Dordrecht, Netherlands : Kluwer Academic Publishers .
- Tudoreanu , L and Phillips , CJC . 2004 . Modeling cadmium uptake and accumulation in plants . Adv. Agron. , 84 : 121 – 157 .
- USEPA 1995: Method 3051: Microwave assisted acid digestion of sediments, sludges, soils, and oils. http://www.epa.gov/SW-846/pdfs/3051.pdf (July 2004). USEPA, Washington, DC
- Welch , RM , Allaway , WH , House , WA and Kubota , J . 1991: Geographic distribution of trace element problems. In Micronutrients in Agriculture SSSA Book Ser. 5., Ed. Mortvedt JJ, pp. 31–58. SSSA, Madison, WI
- Welch , RM , Hart , JJ , Norvell , WA , Sullivan , LA and Kochian , LV . 1999 . Effects of nutrient solution zinc activity on net uptake, translocation, and root export of cadmium and zinc by separated sections of intact durum wheat (Triticum turgidum L . var durum) seedling roots. Plant Soil , 208 : 243 – 250 .
- Welch , RM and Norvell , WA . 1993 . Growth and nutrient uptake by barley (Hordeum vulgare L. cv Herta): Studies using an N. (2-hydroxethyl)) ethylenedinitrilotriacetic acid-buffered nutrient solution technique II. Role of zinc in the uptake and root leakage of mineral nutrients . Plant Physiol. , 102 : 627 – 631 .
- White , JG and Zasoski , RJ . 1999 . Mapping soil micronutrients . Field Crop Res. , 60 : 11 – 26 .
- Wu , FB and Zhang , GP . 2002 . Alleviation of cadmium-toxicity by application of zinc and ascorbic acid in barley . J. Plant Nutr. , 25 : 2745 – 2761 .
- Wu , J , Zhang , X , Nie , Y , Jin , S and Liang , S . 2004 . Factors affecting somatic embryogeneis and plant regeneration from a range of recalcitrant genotypes of Chinese cottons (Gossypium hirsutum L.) . In Vitro Cell. Biol. , 40 : 371 – 377 .