Abstract
In forest soils contaminated by radiocesium (134Cs and 137Cs), deposition from the Fukushima nuclear accident, clay minerals might play important roles in long-term cesium (Cs) dynamics through sorption. To determine whether radiocesium can be retained within the organic layer and the upper mineral soil layers in the Fukushima region, we investigated the vertical distribution of 134Cs and 137Cs and the clay mineral composition in five soil profiles of varying radiocesium deposition levels and vegetation types. X-ray diffraction analyses and oxalate extraction suggested that hydroxy-interlayered vermiculites and short-range-ordered aluminum (Al) and iron (Fe) compounds (i.e, allophane and ferrihydrite) were major clay mineral species of the upper soil layers. The vertical soil distribution of 134Cs and 137Cs suggested that most of them were retained in the organic layer and upper mineral soil layer under different levels of deposition. Within 1.5 years after the accident, both 134Cs and 137Cs were leached from the organic layer, and most of these (59–73%) were accumulated in the upper soil layer (0–5 cm). The proportion of 137Cs (or 134Cs) leaching from the organic layer was greater at sites receiving greater amounts of precipitation. The substantial accumulation of 137Cs in the upper soil layer, irrespective of the 137Cs deposition level or clay mineral composition, suggests that sorption capacities of clays and organic matter are sufficiently high to retain 137Cs in the surface soil during at least the initial stage of contamination.
Key words:
INTRODUCTION
The accident at the Fukushima Dai-ichi Nuclear Power Plant (FNPP), which resulted from the large tsunami after the March 11, 2011 Tohoku-Oki earthquake, led to the environmental contamination of radiocesium species (134Cs and 137Cs) (Yasunari et al. Citation2011; 6–36 × 1015 Bq from Adachi et al. Citation2013). Because 134Cs and 137Cs have relatively long half-lives (2.06 and 30.17 yr, respectively), their contamination has the potential to persist for years to decades (Schimmack et al. Citation1997; Hashimoto et al. Citation2013). Therefore, monitoring the initial deposition of 134Cs and 137Cs and their migration is crucial to forestry and agriculture for the prediction of short- and long-term dynamics within a forest ecosystem and transfer to downstream ecosystems.
Most of the radiocesium deposited onto the canopy and litter layers is predicted to translocate into the mineral soil within the forest ecosystem (Hashimoto et al. Citation2013). Monitoring of 137Cs dynamics in Chernobyl forests has also shown that soils have been the dominant sink of 137Cs during the decades following the accident (Konopleva et al. Citation2009). However, the cesium (Cs) retention capacities of soils do vary depending on climate, vegetation and soil types (Rosén et al. Citation1999). The larger amounts of precipitation can increase Cs leaching, especially in organic soils (Sigurgeirsson et al. Citation2005). The smaller 137Cs retention capacities were also reported for the Fukushima forest soils rich in organic matter (Koarashi et al. Citation2012). The dynamics of radiocesium need to be studied for Fukushima soils, which are influenced by large amounts of precipitation and volcanic ash deposition (Shoji et al. Citation1985).
The retention of radiocesium in soil can occur mainly by sorption onto clays (Cremers et al. Citation1988). Due to the larger surface areas of finer soil particles, a dominant proportion of 137Cs was reported to be accumulated within the clay fraction [e.g., 41% of 137Cs in 12% clay from Livens and Baxter (Citation1988); 69–93% of 137Cs in 24–27% clay from Gerzabek et al. (Citation1992)]. Because 137Cs retention in the surface soil layers can increase with increasing clay content (Koarashi et al. Citation2012), clay content has been considered as one of important factors regulating Cs retention. Further, the laboratory studies revealed that clay mineral composition, especially abundance of the micaceous clay mineral (or illite), can also have influence on long-term 137Cs stabilization (Konopleva et al. Citation2009; Giannakopoulou et al. Citation2012), although its effects on initial Cs dynamics have not been observed from the field survey (Matsunaga et al. Citation2013).
Of a variety of clay minerals, mica and its weathered products may play important roles in Cs retention in soil profiles due to a high layer charge of 2:1 phyllosilicate (Hird et al. Citation1996; Konopleva et al. Citation2009). Illite exhibits the highest selectivity of Cs sorption (Delvaux et al. Citation2001). Cs is selectively sorbed and fixed in wedge-shaped expanded zones of illite clay interlayers, called frayed edge sites (FES) (Cremers et al. Citation1988; Hird et al. Citation1996). FES account for a small proportion (ca. 1%) of the cation exchange capacity (CEC) but dominate the sorption of trace amounts of Cs (Cremers et al. Citation1988). Mica weathering products [especially vermiculite and hydroxy-interlayered vermiculite (HIV)] exhibit lower Cs selectivity than illite, however, they remain illitic in their core structure, and Cs fixation can occur on vermiculitic sites neighboring illitic wedge zones (Delvaux et al. Citation2001). The volcanic ash soils are typically poor in FES from illite clays, and the abundant clay mineral species, allophane, generally exhibit smaller Cs sorption capacities (Vandebroek et al. Citation2012). These factors, along with low pH, high organic matter content and high extent of aluminum (Al) interlayering (formation of HIV minerals), can be hypothesized to limit Cs retention in the surface layers of volcanic soils (Maes et al. Citation1999; Staunton et al. Citation2002; Nakao et al. Citation2008, Citation2009). The mobility of 134Cs and 137Cs in Fukushima forest soils might differ from that in Chernobyl grassland or forest soils derived from loess and glacial deposits.
In addition to being affected by chemical equilibria between Cs and clays, the depth distribution of radiocesium in soil profiles is regulated by various physical and biological processes, such as the accumulation and decomposition of organic matter, migration of particulate matter and recycling via litterfall (Nimis Citation1996; Kruyts and Delvaux Citation2002). Also, the migration of radiocesium from organic layers into the mineral soil can vary with vegetation type, depending on the thickness and decomposition rate of the organic layers (Andolina and Guillitte Citation1990; Rafferty et al. Citation2000). Larger proportions of 137Cs were reported to be held within thick (moder-type) organic layers even 2 years after the Chernobyl accident (Andolina and Guillitte Citation1990). Because organic layers are usually thin in Japanese forests (Takahashi et al. Citation2010), the migration rates into the mineral soil layers of Cs might be different from those in Chernobyl grassland or forest soils.
In the present study, we investigated clay mineral composition and the initial vertical distribution of 134Cs and 137Cs in five forest soil profiles, which were selected based on the gradient of radiocesium deposition level and vegetation type. We sought to address three questions: (1) whether 137Cs (or 134Cs) could be retained within the soil profiles under different levels of radiocesium deposition, (2) whether 137Cs deposited onto organic layers could migrate into mineral soil layers within the initial period following the accident and (3) whether the potential adsorbents of radiocesium, mica and its weathering products, are present or not in the Fukushima forest soils affected by volcanic materials.
MATERIALS AND METHODS
Experimental sites
We selected three study sites, Kawauchi, Ohtama and Tadami, along the deposition-level gradient (). Deposition of 134Cs and 137Cs at the forest sites amounted to 1020 kBq m−2, 73 kBq m−2, and 16 kBq m−2 at Kawauchi, Ohtama and Tadami, respectively [Forestry and Forest Products Research Institute (FFPRI) Citation2011a, Citation2011b]. The Tadami site was located on a steep slope (29°), whereas the other sites were located on moderate slopes having an average inclination of 8° to 16°. The Kawauchi site [37°17’N, 140°47’E, 660 m above sea level (a.s.l.)] consisted solely of Japanese cedar plantation (KC plot; 43-year-old Cryptomeria japonica D. Don). The KC soil, derived from volcanic ash and granite, has been classified as Andisols (Soil Survey Staff Citation2006) or Brown forest soils (Forest Soil Division Citation1975; Kaneko et al. Citation2013). The Ohtama site (37°34’N, 140°18’E, 730–780 m a.s.l.) included three plots: one of pine forest (OP), one of mixed deciduous forest (OM), and one of cedar forest (OC). The OP plot was dominated by 43-year-old Pinus densiflora S. et Z., the OM plot was dominated by broad-leaved species such as Quercus serrata M. and Castanea crenata S. et Z. mixed with Pinus densiflora, and the OC plot was a 42-year-old Cryptomeria japonica plantation. The soils of the OP, OM and OC plots were derived from volcanic ash and andesite. The OP and OM soils have been classified as Andisols or Brown forest soils. The OC soil, in which a buried A horizon was observed in the subsoil (49–82 cm), was classified as an Andisol or Black soil. The Tadami site (37°19′N, 139°31′E; 680 m a.s.l.) consisted solely of cedar plantation (TC plot; 41-year-old Cryptomeria japonica). The TC soil, which was derived from volcanic ash and tuffaceous sandstone, has been classified as Inceptisols or Brown forest soils. The mean annual air temperature in the most recent 10 years at the study sites ranged from 10.3 to 12.2°C. Annual precipitation was 1546 mm yr−1 at the KC site and 1261 mm yr−1 at the Ohtama site, but it was considerably higher (2573 mm yr−1) at the TC site due to large amounts of snowfall.
Soil sampling
The inventory study of radiocesium (kBq m−2; aboveground biomass and soil) was carried out by FFPRI (Citation2011a, Citation2011b). For measurement of soil radiocesium activity, both the organic layers and mineral soil layers were sampled from August to September of 2011 and 2012, i.e., 0.5 and 1.5 years after the accident, at all sites. Briefly, organic layers were sampled from 25-cm quadrates in 12 replicates. Surface soil layers were collected using a core sampler (diameter of 110 cm and height of 5 cm) in 12 replicates, whereas deeper soil layers (5–10, 10–15 and 15–20 cm) were collected in three replicates. For analyses of physicochemical and mineralogical properties, soil samples were collected from pedogenetic horizons. The data of physicochemical properties from pedogenetic horizons were converted to depth increments (0–5, 5–10, 10–15 and 15–20 cm). All soil samples were air-dried and crushed to pass through a 2-mm sieve and were analyzed for radiocesium activity and physicochemical and mineralogical properties.
Radiocesium activity in organic and mineral soil materials
Each soil sample (ca. 50 g) was compressed into a plastic container (100 mL), and the total concentration of 134Cs and 137Cs in the bulk soil of the core samples was determined using a high-purity Ge gamma-ray detector (GC2020-7500SL-2002CSL, CANBERRA, Meriden, USA) connected to a multichannel analyzer system. The stocks of 134Cs and 137Cs in the soil layers (0–5 cm, 5–10 cm, 10–15 cm and 15–20 cm) were calculated using the respective concentrations and bulk density of the soil. The downward fluxes of 137Cs (or 134Cs) into the organic layer and the mineral soil layer (0–5 cm) were calculated from the net increase of 137Cs (or 134Cs) in the underlying soil layers. To estimate the radioactive decay of the stocks, the remaining proportions of 134Cs and 137Cs at time t (yr) were calculated as follows:
where N(t) and N(0) represent the activities of radiocesium at time t (yr) and time 0, respectively, and λ represents the decay constant (λ of 134Cs = 0.346 and λ of 137Cs = 0.023).
Physicochemical properties of soils
Soil pH was measured using a soil to solution [H2O or 1 M potassium chloride (KCl)] ratio of 1:5 (w/v) after shaking for 1 h. Total carbon (C) and nitrogen (N) contents in soils were determined using a CN analyzer (Thermo Fisher Scientific, Flash EA 1112). Particle size distribution was determined by a pipette method. The exchangeable basic cations and CEC were determined using the ammonium acetate (1 M and pH 7.0) method. The short-range-ordered Al, iron (Fe), and silicon (Si) compounds (Alo, Feo, and Sio) were determined by extraction in the dark with acid (pH 3) 0.2 M ammonium oxalate (McKeague and Day Citation1966). The concentrations of Al, Fe and Si in extracts were measured using inductively coupled plasma atomic emission spectrometry (ICP-AES, Thermo Fisher Scientific, iCAP6500Duo). The contents of allophane in the soil samples were estimated by multiplying Sio with a factor (7.14), while those of ferrihydrite were estimated by multiplying Feo with a factor (1.7) (Parfitt and Henmi Citation1982; Parfitt and Childs Citation1988). The contents of crystalline clay minerals were estimated by subtracting the contents of allophane and ferrihydrite from the clay content, which was re-calculated on the basis of bulk soil.
Mineralogical properties of soils
The clay mineral composition of selected soil samples was examined using the X-ray diffraction (XRD) method on parallel-oriented clay (< 2 μm) specimens subjected to magnesium (Mg) saturation, potassium (K) saturation, glycerol solvation and heat treatment (300°C, 550°C) for K-saturated specimens. XRD patterns were obtained using an X-ray diffractometer (Rigaku, RINT 2000) with CuKα radiation. The relative contents of illite, vermiculite (Vt) and quartz (Qz) were estimated based on the following formulae (Matsue and Wada Citation1988, Citation1989):
where I1.0 and I1.4 are the XRD intensities (peak heights) of K- and Mg-saturated and air-dried clay samples at 1.0 and 1.4 nm, respectively. The other 1.4-nm clay minerals, HIV, have been estimated from the 1.4-nm peak height of K-saturated clay samples when chlorite (Ch) was negligible (Matsue and Wada Citation1989; Ohta et al. Citation1993). Because small amounts of chlorite were detected in our study, the relative contents of HIV and Ch were estimated from the 1.4-nm peak height of K-saturated clay samples. The degrees of Al interlayering were estimated using the intensity ratio of HIV and Ch to 1.4-nm clay minerals (Matsue and Wada Citation1988):
The sum of relative contents of Illite, Vt, HIV and Ch equals 1.
Calculations and statistics
All results were expressed on an oven-dried (24 h, 105°C) weight basis and as means ± standard error (SE) of 12 determinations for organic layers, nine determinations for surface soil layers (0–5 cm) and three determinations for deeper soil layers (5–10, 10–15 and 15–20 cm). The significance of differences in mean values between groups (horizons, sites and 2011 vs. 2012) were tested using an analysis of variance (ANOVA) at the p < 0.05 significance level for Cs concentrations and stocks. Spearman’s linear correlations were used to assess the relationships between Cs loading and soil Cs stock, and between Cs loading and soil Cs fluxes. The statistical analyses were performed using SYSTAT ver. 11.0 (SPSS Inc., 2008).
RESULTS
Soil physicochemical properties
The soils were moderately acidic (pH of 4.4 to 5.2), consistent with low base saturation (). Exceptionally, base saturation [especially exchangeable calcium (Ca)] was high in the surface layers of the OC soil due to incorporation of Ca-rich cedar litter. Soil C contents were high from the top to the 15-cm depth at all sites except for TC soil, which was derived from sandstone. The fine fraction (clay + silt) was high at all sites (45 to 75%), but it was lower at the TC site than at the other sites, probably due to the influence of the bedrock (tuffaceous sandstone) (). Soil C contents were positively correlated with fine (clay + silt) fractions (r = 0.68, p < 0.01, n = 15). Variable but high contents of Sio indicated that most of the soil horizons have been influenced by volcanic materials (). The contents of oxalate-extractable Al and Fe compounds (Alo + 1/2 Feo) satisfied the criterion of andic soil properties (> 20 g kg−1 soil) throughout the profiles of all sites, except for the A horizon of the KC soil and the TC soil profile (). The contents of Alo + 1/2Feo in the TC soil were low due partly to surface erosion on the steep slope.
Table 1 Physicochemical properties of the five forest soils
Clay mineralogical properties
Allophane and ferrihydrite accounted for a significant proportion of the total clay fractions for all of the soil samples (). Especially in the deeper soil layers of Ohtama three plots, allophane and ferrihydrite accounted for high proportions (23 to 55%) of clay fractions. The clay mineral species are dominated by crystalline clay minerals in the upper mineral soil layers at all plots ().
Table 2 Physical and mineralogical properties of the five forest soils
According to XRD patterns of clay fractions, the illite peak was identified from the reflection at 1.0 nm (), but the relative contents of illite in our study were small for all of the clay samples (). The absence of a peak at 1.8 nm after glycerol solvation suggests that smectite was negligible for all of the samples (not shown). The chlorite peak was identified from the 1.4-nm reflection when the K-saturated clay samples were heated at 550°C (). At the same time, the intensities of the 1.2-nm reflection, which corresponds to HIV, increased after heating (550°C) of the K-saturated clay samples at the expense of the 1.4-nm reflection at 20 and 300°C (not shown). The chlorite peak was consistently small and the relative contents of HIV and chlorite corresponded predominantly to HIV for all the samples studied (). Quartz was identified from reflections of 0.43 nm () and 0.33 nm (not shown). The decrease of the 1.4-nm reflection after K saturation was small, and the degree of Al interlayering was consistently high for the clay samples studied. The degree of Al interlayering was positively correlated with the contents of Alo (r = 0.61, p < 0.01, n = 15) and Alo + 1/2Feo (r = 0.66, p < 0.01, n = 15) ( and ). Accordingly, the relative contents of vermiculite were negatively correlated with these parameters ( and ).
Figure 2 X-ray diffraction patterns of clay fractions from the surface soil layers of three cedar sites of Kawauchi, Ohtama, and Tadami (KC, OC and TC). “Mg-” and “K-” represent magnesium saturation and potassium saturation, respectively, and the following numbers, “20” or “550”, are the temperatures (°C) at which the samples were dried or heated.
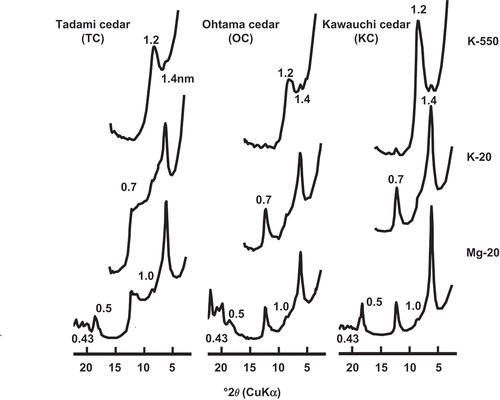
Initial depth distribution and migration of radiocesium within the soil profiles
In 2011, the concentrations of radiocesium (134Cs and 137Cs) in the soils increased toward FNPP in the following order: KC > OP, OM, OC > TC. At all sites, the concentrations of both 134Cs and 137Cs were largest in the organic layer and decreased with depth (). The concentrations of 134Cs and 137Cs decreased significantly (p < 0.05) in the organic layers from 2011 to 2012, while they increased significantly (p < 0.05) for the upper mineral soil layer (0–5cm). Significant differences in 137Cs (or 134Cs) concentrations between 2011 and 2012 could not be observed for the deeper soil layers (below 5 cm); Cs concentrations were highly variable in these layers.
Table 3 Depth distribution of 134Cs and 137Cs in the soil profiles
Because the vertical distribution patterns for 134Cs and 137Cs were similar, the 137Cs stocks in the soil are mainly discussed hereafter. The total 137Cs stock in the soil (August 2011, organic layer to 20 cm depth) varied widely from 12 kBq m−2 (TC) to 558 kBq m−2 (KC) (). Regarding the 137Cs concentration, the total stock of 137Cs in the soil increased toward FNPP. Within the soil profiles, 137Cs stocks in 2011 were largest in the organic layers at all sites. When the total soil 137Cs stock was regarded as the “137Cs loading” by neglecting the pre-accident 137Cs stock, the 137Cs stocks in the organic layer corresponded to 44 to 64% of the 137Cs loadings at the point of August 2011 (after the initial 0.5 years from the accident).
Table 4 Percentages of 137Cs retention in the soil compartments relative to 137Cs loading
After the subsequent 1 year (August 2011 to August 2012), the total 137Cs stock in the soil (organic layer to 20 cm depth) increased significantly (p < 0.05) due to radiocesium input from throughfall and litterfall (). However, the 137Cs stock in the organic layer decreased significantly (p < 0.05) from 2011 to 2012 at all sites (). The proportions of 137Cs stocks relative to their loadings decreased from 44–64% in 2011 to 14–27% in 2012 (). When 137Cs retention in the organic layer was compared among the five sites, the remaining proportion of 137Cs in the organic layer was lower at the KC and TC sites, which received higher amounts of precipitation (1546 and 2573 mm yr−1) than the three Ohtama sites. For the three Ohtama sites, the remaining proportion of 137Cs in the organic layer did not vary significantly between vegetation types ().
Figure 3 Vertical distribution of radiocesium in the five soil profiles from 2011 to 2012. Bars represent standard errors [n = 12 for organic layer and soil (0–5 cm); n = 3 for the deeper soil layers].
![Figure 3 Vertical distribution of radiocesium in the five soil profiles from 2011 to 2012. Bars represent standard errors [n = 12 for organic layer and soil (0–5 cm); n = 3 for the deeper soil layers].](/cms/asset/faee15b3-761e-4aba-b055-2d2f0790610a/tssp_a_926781_f0003_b.gif)
When the radioactive decay of the radiocesium stocks after 1 year (August 2011 to August 2012) was calculated using Eq. 1, the stocks of 134Cs and 137Cs would decrease by 29% and 2%, respectively. In the case of the upper 0–5-cm soil layer of KC, the decrease of 137Cs stock by radioactive decay amounted to 4 kBq m−2. Despite this, the 137Cs stock in the upper soil layer increased significantly (p < 0.05) from 159 kBq m−2 (2011) to 422 kBq m−2 (2012). Similarly, the 134Cs stock also increased from 135 to 265 kBq m−2, despite greater radioactive decay (40 kBq m−2). In 2012, the 134Cs and 137Cs stocks of the upper soil layer exceeded those of the organic layer. This trend was observed at all sites, although the magnitude tended to decrease with increasing distance from FNNP (). No significant increase in 137Cs (or 134Cs) stocks was observed for the deeper soil layers (). The 137Cs retention in the upper soil layer (0–5 cm) increased from 29–41% (2011) to 60–72% (2012) of the 137Cs loading (). The percentages of 137Cs (or 134Cs) retention in the upper soil layers had no correlation with the relative contents of clay minerals, the contents of allophane and clay percentages, respectively ( and ).
DISCUSSION
Characteristics of clay mineral composition in Fukushima soils
The clay mineralogy of forests soils in the Fukushima region is strongly influenced by volcanic ash deposition. The results of XRD and oxalate extraction showed that the major clay mineral species in our study were HIV and short-range-ordered Al and Fe compounds (i.e., allophane and ferrihydrite), irrespective of the variation of bedrock (; ). The relative contents of illite in our study were lower than those in soils from sedimentary rocks (0.19 to 0.85; Matsue and Wada Citation1989; Ohta et al. Citation1993) due partly to the lower contents of illite in volcanic materials. However, it should be noted that abundance of illite could not be evaluated solely from XRD patterns (Thompson and Ukrainczyk Citation2002). The greater degree of Al interlayering in the soils from our study (), compared to other soil types (0.12–0.38 from Pai et al. Citation2004), is consistent with the finding that HIV is the most abundant clay mineral species of nonallophanic Andisols in the Fukushima region (Shoji et al. Citation1985). This can be supported by (1) less acidic soil conditions (pH of 4.6 to 5.8 from Rich Citation1968; Bain et al. Citation1990), (2) low concentrations of chelating organic acids (Malcolm et al. Citation1969; Fujii et al. Citation2008, Citation2010), and (3) a supply of Al from short-range-ordered Al compounds. Regarding the third point above, the high amount of hydroxyl-interlayered vermiculite requires sources of micaceous minerals and interlayer Al compounds. Micas and chlorite can be supplied from bedrock and aeolian dust (Bautista-Tulin and Inoue Citation1997), whereas interlayer Al compounds can be supplied from short-range-ordered Al compounds (Ndayiragije and Delvaux Citation2003; Yagasaki et al. Citation2006). The high amounts of Al released from short-range-ordered Al compounds are favorable for Al interlayering of vermiculite, as suggested by the positive correlation between the degree of Al interlayering and Alo contents ().
Effects of deposition levels on the distribution of radiocesium in Fukushima soil profiles
The temporal fluctuation of radiocesium in soils after the accident needs to be discussed along with the pre-accident stocks and the time-dependent decrease caused by radioactive decay (Almgren and Isaksson Citation2006). The pre-accident concentration of 137Cs, which was derived largely from atmospheric nuclear tests and the Chernobyl accident, has been reported to be ~48 Bq kg−1 in Japanese forest soils (Takenaka et al. Citation1998; Yamaguchi et al. Citation2012). Assuming a similar pre-accident fallout level at our sites, most of the measured radiocesium would have originated from FNPP (). Accordingly, the total stock of 137Cs in the soil profile (organic layer to 20-cm depth), which can be regarded as the 137Cs loading to the soil, increased with decreasing distance from FNNP ().
About 50–62% of the 137Cs loading were retained in the organic layer after the initial 0.5 years (March 2011 to August 2011). The stock of 137Cs (or 134Cs) in the organic layer in 2011 increased with increasing 137Cs loading (deposition level) (). This suggests deposition of 137Cs from FNPP and its accumulation in the organic layer. After the following 1 year (August 2011 to August 2012), 137Cs stock in the organic layer decreased to 14–27% of the 137Cs loading at all sites. The significant loss of 137Cs in the organic layer could be caused by both radioactive decay and leaching (Nakanishi et al. Citation2014). Judging from the facts that (1) the 1-year radioactive decay can account for only a 29% loss of 134Cs and a 2% loss of 137Cs and (2) the dominant sink of 134Cs and 137Cs changed from the organic layer to the upper soil layer (), the loss of radiocesium from the organic layers probably resulted largely from leaching.
The proportions of 134Cs and 137Cs stock in the mineral soil layer relative to their loading increased from 29–43% in 2011 to 59–73% in 2012 (). Radioactive decay could cause a decrease in the 134Cs and 137Cs stocks by 29% and 2%, respectively, from 2011 to 2012; however, these increased in the upper 0–5-cm soil layers (). The significant gain of radiocesium in this layer within the 1 year could have resulted from an overwhelming magnitude of leaching from the organic layer. The changes in the 134Cs and 137Cs stocks between 2011 and 2012 indicated input of radiocesium from aboveground biomass (canopy) and organic layer and their accumulation in the mineral soil (). Although the downward flux of 137Cs (or 134Cs) from the organic layer increased with 137Cs loading, most of the flux was retained in the upper 0–5-cm soil layer irrespective of deposition level (; ). The markedly low mobility of Cs relative to the water flux suggests that Cs mobility is strongly limited by chemical processes (i.e., sorption), rather than physical or biological processes. Even with a high 137Cs loading, the upper layer of the KC soil exhibited high efficiency of retention without approaching saturation (). Although Andisols typically exhibit low sorption capacity of 137Cs (Vandebroek et al. Citation2012), a variety of retention sites and processes in the volcanic soils can contribute to 137Cs retention (Joussein et al. Citation2004; Sigurgeirsson et al. Citation2005), as is discussed later. The 137Cs accumulation within the upper 5-cm soil layer in our study () is consistent with reports for grasslands and forests in Germany and agricultural lands in Sweden, where most of the 137Cs was retained even 7 to 20 years after the Chernobyl accident (Schimmack et al. Citation1997; Almgren and Isaksson Citation2006; Konopleva et al. Citation2009). Although the greater precipitation and volcanic soils at our sites are different from the conditions of their studies, low mobility of 137Cs might be common to soils rich in sorption and fixation sites, which contrasts with the rapid migration of 137Cs observed in Histosols or Spodosols (Rosén et al. Citation1999; Sigurgeirsson et al. Citation2005).
Role of organic layer thickness in 137Cs retention
Both 134Cs and 137Cs exhibited similar patterns of initial migration and retention in our study; however, an increasingly large gap will occur due to their differences in half-life. 137Cs retention within the soil needs to be focused to predict long-term dynamics. The factors regulating 137Cs retention and migration in the organic and mineral soil layers are discussed in the present and following sections to answer the questions (1) and (2) raised in the introduction.
Because 137Cs retention capacities in organic layers are typically small compared to those in mineral soil layers (Shand et al. Citation1994), the migration of 137Cs into mineral soil layers is hypothesized to be rapid in the case of a thin organic layer. In accordance with this, 137Cs retention in the organic layer was consistently small 1.5 years after the accident (). The initial vertical migration of 137Cs from the organic layer is driven by (a) initial washout and (b) decomposition and leaching. Regarding (b), the mass of the organic layer is mostly small in Fukushima forest soils (Kaneko et al. Citation2013), suggesting a rapid turnover of litter. The rapid loss of 137Cs (or 134Cs) from the organic layer is considered to be caused by rapid wash-out of deposited 137Cs from the thin organic layer and rapid decomposition of litter, which can mobilize 137Cs associated with organic matter (Nakanishi et al. Citation2014). Small retention capacities of 137Cs were also reported from a thin (mull-type) organic layer, but this contrasts with the greater retention (60 to 75%) in a thick organic layer (moder-type; 6 cm thick) 2 to 5 years after the Chernobyl accident (Andolina and Guillitte Citation1990; Rafferty et al. Citation2000). Thick organic layers can retain higher amounts of 137Cs due to sorption onto clay minerals admixed into humified internal layers (Shand et al. Citation1994). In our study, larger proportions of 137Cs were leached from the organic layer at the TC and KC sites, which received greater precipitation than the OC site (), highlighting the importance of precipitation as a key factor regulating 137Cs leaching flux from the organic layer. Small Cs retention and rapid leaching appear to be common in “mull-type” organic layers in this region, irrespective of vegetation species.
Potential importance of clay minerals in soil 137Cs dynamics
The clay content and clay mineral composition can influence 137Cs retention and stabilization process (Rosén et al. Citation1999; Konopleva et al. Citation2009). In our study, irrespective of clay properties or 137Cs deposition levels, 137Cs retention in the surface soil layer (59 to 73% of 137Cs deposition) was consistently high at all sites (). Although volcanic soils typically exhibit small 137Cs fixation capacities (Vandebroek et al. Citation2012), the upper mineral soil layers appeared to have retention capacities of 137Cs (). The possible processes of 137Cs retention in soil are fixation on the vermiculitic sites associated with illitic wedge zones and sorption on exchangeable sites derived from organic matter and clay minerals (Chorover et al. Citation1999; Matsunaga et al. Citation2013). The importance of mica and its weathering products (illite, vermiculite and HIV) in 137Cs stabilization is supported by the positive correlations between FES and abundance of illite and mixed-layer minerals (Cremers et al. Citation1988), vermiculite (Delvaux et al. Citation2001) and illite (Nakao et al. Citation2014). The abundant 2:1 phyllosilicate mineral species in our study, HIV, generally exhibits considerably smaller fixation capacities (Maes et al. Citation1999; Nakao et al. Citation2009), but it can retain 137Cs through fixation in FES situating at the fringe of non-interlayered structures. The fixation of 137Cs within illite and vermiculite could not be supported in our study due to their small XRD peak intensities (; ); however, this might be due to the limitations of XRD detection (Thompson and Ukrainczyk Citation2002). In addition, illite in minor abundance, which is not detectable from XRD patterns, may possess sufficient fixation capacities relative to trace amounts of 137Cs (Hird et al. Citation1995). Allophane, which is another major constituent of clay minerals in our study (), can also possess low but significant amounts of FES (De Koning et al. Citation2007). Thus, these clay minerals have the potential to be 137Cs reservoirs in the volcanic soils studied. The high CEC from organic matter and clays can also contribute to 137Cs retention in the surface soil layers (). Based on these findings, the high 137Cs retention in our study can be explained in part by the presence of selective sorption sites from a variety of clays (e.g., HIV, illite, allophane) and exchangeable sites from clays and organic matter in all surface soil samples (; ), although quantitative importance of the individual processes remains to be evaluated.
When the amount of 137Cs retention and the number of Cs-specific sorption sites are compared at the KC site, the 137Cs concentration (31,448 Bq kg−1) in the 0–5-cm layer is calculated to be 7.2 × 10−8 mmol kg−1 (1 Bq 137Cs ≡ 2.3 × 10−15 mol). The number of 137Cs-specific sorption sites is reported to range from 0 to 1 × 10−5 mmol kg−1 in the organic layer (Hird et al. Citation1996; De Koning et al. Citation2007) and from 3.0 × 10−2 to 2.8 mmol kg−1 soil in the mineral soil layer (Cremers et al. Citation1988; Hird et al. Citation1996). These data suggest that the sorption capacity of clays could be sufficiently high to fix 137Cs in even highly contaminated soil. However, our data showed only that most of the radiocesium can be retained in the surface soil layer during at least the initial stage of contamination. Histosol and sandy Spodosol soils devoid of FES were reported to exhibit a deeper distribution of 137Cs eight years after the Chernobyl accident (Rosén et al. Citation1999).
To predict the long-term 137Cs dynamics in Fukushima forests, long-term monitoring and evaluation of FES are required for the volcanic soils. In addition, there are several uncertainties regarding the contribution of fixation to whole 137Cs retention, fixation/desorption of mobile 137Cs with aging, and mobility of 137Cs (e.g., physical migration of 137Cs-fixed particles by preferential water flow, erosion and mixing by soil animals) in volcanic soils. With regard to 137Cs cycling between plant and soil, as the organic layer acts as a source of mobile 137Cs for plants (Kruyts and Delvaux Citation2002), the migration of 137Cs into the mineral soil layer is expected to decrease the risks of 137Cs transfer to plants in our study. An understanding of the mobility and bioavailability of 137Cs in relation to retention forms for volcanic soils is also needed.
CONCLUSIONS
All of the soil horizons contained hydroxy-interlayered vermiculites and short-range-ordered Al and Fe compounds including allophane and ferrihydrite. The deposited 137Cs was leached from the organic layer, and most of the 137Cs accumulated in the upper soil layer within 1.5 years after the accident. A larger proportion of 137Cs was leached from the organic layers at sites receiving greater amounts of precipitation. Accumulation of 137Cs in the upper 0–5 cm soil layer, irrespective of 137Cs loading and clay mineral composition, suggests that the sorption capacities of clays and organic matter are sufficiently high to retain 137Cs even in the upper mineral layers. However, it should be noted that the results obtained during the initial stage of contamination could not be extrapolated for long-term 137Cs dynamics; therefore, long-term monitoring is warranted.
ACKNOWLEDGMENTS
This study was conducted as an FFPRI project study (F1P07) and was also funded by the Forestry Agency. We would like to thank the Kanto Regional Forest Office and the Rural Development Division of Kawauchi Village for allowing us to conduct studies at the experimental sites. We are also grateful to the members of FFPRI for the field sampling and measurements.
REFERENCES
- Adachi K, Kajino M, Zaizen Y, Igarashi Y 2013: Emission of spherical cesium-bearing particles from an early stage of the Fukushima nuclear accident. Sci. Rep., 3, 2554. doi:10.1038/srep02554
- Almgren S, Isaksson M 2006: Vertical migration studies of 137Cs from nuclear weapons fallout and the Chernobyl accident. J. Environ. Radioact., 91, 90–102. doi:10.1016/j.jenvrad.2006.08.008
- Andolina J, Guillitte O 1990: Radiocesium availability and retention sites in forest humus. In Transfer of radionuclides in natural and semi-natural environments, Ed. Desmet G et al., pp. 135–142. Elsevier, London and New York.
- Bain DC, Mellor A, Wilson MJ 1990: Nature and origin of an aluminous vermiculitic weathering product in acid soils from upland catchments in Scotland. Clay Min., 25, 467–475. doi:10.1180/claymin.1990.025.4.05
- Bautista-Tulin AT, Inoue K 1997: Hydroxy-Interlayered minerals in Japanese soils influenced by eolian deposition. Soil Sci. Soc. Am. J., 61, 631–640. doi:10.2136/sssaj1997.03615995006100020037x
- Chorover J, Dichiaro MJ, Chadwick OA 1999: Structural charge and cesium retention in a chronosequence of tephritic soils. Soil Sci. Soc. Am. J., 63, 169–177. doi:10.2136/sssaj1999.03615995006300010024x
- Cremers A, Elsen A, De Preter P, Maes A 1988: Quantitative analysis of radiocaesium retention in soils. Nature, 335, 247–249. doi:10.1038/335247a0
- De Koning A, Konoplev AV, Comans RNJ 2007: Measuring the specific caesium sorption capacity of soils, sediments and clay minerals. Appl. Geochem., 22, 219–229. doi:10.1016/j.apgeochem.2006.07.013
- Delvaux B, Kruyts N, Maes E, Smolders E 2001: Fate of radiocesium in soil and rhizosphere. In Trace Elements in the Rhizosphere, Ed. Gobran GR et al., pp. 61–91. CRC Press, London.
- Forest Soil Division 1975: Classification of Forest Soil in Japan. Bull. Gov. For. Exp. Stat., 280.
- Forestry and Forest Products Research Institute 2011a : Report on radionuclide distribution in Fukushima forests. (in Japanese) http://www.ffpri.affrc.go.jp/press/2011/20110930/documents/110930-01.pdf (October, 2013)
- Forestry and Forest Products Research Institute 2011b : Report on radionuclide distribution in Fukushima forests. (in Japanese) http://www.rinya.maff.go.jp/j/press/hozen/pdf/111227_2-01.pdf (October, 2013)
- Fujii K, Funakawa S, Hayakawa C, Kosaki T 2008: Contribution of different proton sources to pedogenetic soil acidification in forested ecosystems in Japan. Geoderma, 144, 478–490. doi:10.1016/j.geoderma.2008.01.001
- Fujii K, Hayakawa C, Van Hees PAW, Funakawa S, Kosaki T 2010: Biodegradation of low molecular weight organic compounds and their contribution to heterotrophic soil respiration in three Japanese forest soils. Plant Soil, 334, 475–489. doi:10.1007/s11104-010-0398-y
- Gerzabek MH, Mohamad SA, Muck K 1992: Cesium‐137 in soil texture fractions and its impact on cesium‐137 soil‐to‐plant transfer. Commun. Soil Sci. Plant Anal., 23, 321–330. doi:10.1080/00103629209368591
- Giannakopoulou F, Gasparatos D, Haidouti C, Massas I 2012: Sorption behavior of cesium in two Greek soils: Effects of Cs initial concentration, clay mineralogy, and particle size fraction. Soil Sedim. Contam., 21, 937–950. doi:10.1080/15320383.2012.714418
- Hashimoto S, Matsuura T, Nanko K, Linkov I, Shaw G, Kaneko S 2013: Predicted spatio-temporal dynamics of radiocesium deposited onto forests following the Fukushima nuclear accident. Sci. Rep., 3, 2564. doi:10.1038/srep02564
- Hird AB, Rimmer DL, Livens FR 1995: Total caesium-fixing potentials of acid organic soils. J. Environ. Radioact., 26, 103–118. doi:10.1016/0265-931X(94)00012-L
- Hird AB, Rimmer DL, Livens FR 1996: Factors affecting the sorption and fixation of caesium in acid organic soil. Eur. J. Soil Sci., 47, 97–104. doi:10.1111/j.1365-2389.1996.tb01376.x
- Joussein E, Kruyts N, Righi D, Petit S, Delvaux B 2004: Specific retention of radiocesium in volcanic ash soils devoid of micaceous clay minerals. Soil Sci. Soc. Am. J., 68, 313–319. doi:10.2136/sssaj2004.3130
- Kaneko S, Ikeda S, Akama A, Miura S, Takahashi M 2013: Soil physicochemical properties of experimental sites in forests contaminated by radioactive materials due to the Fukushima Daiichi Nuclear Power Plant accident. Jpn. J. For. Environ., 55, 75–81. (in Japanese with English summary).
- Koarashi J, Atarashi-Andoh M, Matsunaga T, Sato T, Nagao S, Nagai H 2012: Factors affecting vertical distribution of Fukushima accident-derived radiocesium in soil under different land-use conditions. Sci. Total Environ., 431, 392–401. doi:10.1016/j.scitotenv.2012.05.041
- Konopleva I, Klemt E, Konoplev A, Zibold G 2009: Migration and bioavailability of 137Cs in forest soil of southern Germany. J. Environ. Radioact., 100, 315–321. doi:10.1016/j.jenvrad.2008.12.010
- Kruyts N, Delvaux B 2002: Soil organic horizons as a major source for radiocesium biorecycling in forest ecosystems. J. Environ. Radioact., 58, 175–190. doi:10.1016/S0265-931X(01)00065-0
- Livens FR, Baxter MS 1988: Particle size and radionuclide levels in some west Cumbrian soils. Sci. Total Environ., 70, 1–17. doi:10.1016/0048-9697(88)90248-3
- Maes E, Vielvoye L, Stone W, Delvaux B 1999: Fixation of radiocaesium traces in a weathering sequence mica → vermiculite → hydroxy interlayered vermiculite. Eur. J. Soil Sci., 50, 107–115. doi:10.1046/j.1365-2389.1999.00223.x
- Malcolm RL, Nettleton WD, McCrachen RJ 1969: Pedogenic formation of montmorillonite from A 2:1–2:2 intergrade clay mineral. Clays Clay Min., 16, 405–414. doi:10.1346/CCMN.1969.0160602
- Matsue N, Wada K 1988: Interlayer materials of partially interlayered vermiculites in dystrochrepts derived from Tertiary sediments. J. Soil Sci., 39, 155–162. doi:10.1111/j.1365-2389.1988.tb01202.x
- Matsue N, Wada K 1989: Source minerals and formation of partially interlayered vermiculites in Dystrochrepts derived from Tertiary sediments. J. Soil Sci., 40, 1–7. doi:10.1111/j.1365-2389.1989.tb01248.x
- Matsunaga T, Koarashi J, Atarashi-Andoh M, Nagao S, Sato T, Nagai H 2013: Comparison of the vertical distributions of Fukushima nuclear accident radiocesium in soil before and after the first rainy season, with physicochemical and mineralogical interpretations. Sci. Total Environ., 447, 301–314. doi:10.1016/j.scitotenv.2012.12.087
- McKeague JA, Day JH 1966: Dithionite- and oxalate-extractable Fe and Al as aids in differentiating various classes of soils. Can. J. Soil Sci., 46, 13–22. doi:10.4141/cjss66-003
- Nakanishi T, Matsunaga T, Koarashi J, Atarashi-Andoh M 2014: 137Cs vertical migration in a deciduous forest soil following the Fukushima Dai-ichi Nuclear Power Plant accident. J. Environ. Radioact., 128, 9–14. doi:10.1016/j.jenvrad.2013.10.019
- Nakao A, Funakawa S, Kosaki T 2009: Hydroxy-Al polymers block the frayed edge sites of illitic minerals in acid soils: studies in southwestern Japan at various weathering stages. Eur. J. Soil Sci., 60, 127–138. doi:10.1111/j.1365-2389.2008.01097.x
- Nakao A, Ogasawara S, Sano O, Ito T, Yanai J 2014: Radiocesium sorption in relation to clay mineralogy of paddy soils in Fukushima, Japan. Sci. Total Environ., 468–469, 523–529. doi:10.1016/j.scitotenv.2013.08.062
- Nakao A, Thiry Y, Funakawa S, Kosaki T 2008: Characterization of the frayed edge site of micaceous minerals in soil clays influenced by different pedogenetic conditions in Japan and northern Thailand. Soil Sci. Plant Nutr., 54, 479–489. doi:10.1111/j.1747-0765.2008.00262.x
- Ndayiragije S, Delvaux B 2003: Coexistence of allophane, gibbsite, kaolinite and hydroxy-Al-interlayered 2:1 clay minerals in a perudic Andosol. Geoderma, 117, 203–214. doi:10.1016/S0016-7061(03)00123-X
- Nimis PL 1996: Radiocesium in plants of forest ecosystems. Stud. Geobot., 15, 3–49.
- Ohta S, Effendi S, Tanaka N, Miura S 1993: Ultisols of lowland Dipterocarp forest in East Kalimantan, Indonesia. Soil Sci. Plant Nutr., 39, 1–12. doi:10.1080/00380768.1993.10416969
- Pai CW, Wang MK, King HB, Chiu CY, Hwong JL 2004: Hydroxy-interlayered minerals of forest soils in A-Li Mountain, Taiwan. Geoderma, 123, 245–255. doi:10.1016/j.geoderma.2004.02.010
- Parfitt RL, Childs CW 1988: Estimation of forms of Fe and Al - a review, and analysis of contrasting soils by dissolution and Mossbauer methods. Aust. J. Soil Res., 26, 121–144. doi:10.1071/SR9880121
- Parfitt RL, Henmi T 1982: Comparison of an oxalate-extraction methon and an infrared spectroscopic method for determining allophane in soll clays. Soil Sci. Plant Nutr., 28, 183–190. doi:10.1080/00380768.1982.10432435
- Rafferty B, Brennan M, Dawson D, Dowding D 2000: Mechanisms of 137Cs migration in coniferous forest soils. J. Environ. Radioact., 48, 131–143. doi:10.1016/S0265-931X(99)00027-2
- Rich CI 1968: Hydroxy interlayers in expansible layer silicates. Clays Clay Min., 16, 15–30. doi:10.1346/CCMN.1968.0160104
- Rosén K, Öborn I, Lönsjö H 1999: Migration of radiocaesium in Swedish soil profiles after the Chernobyl accident, 1987–1995. J. Environ. Radioact., 46, 45–66. doi:10.1016/S0265-931X(99)00040-5
- Schimmack W, Flessa H, Bunzl K 1997: Vertical migration of Chernobyl-derived radiocesium in Bavarian grassland soils. Naturwissenschaften, 84, 204–207. doi:10.1007/s001140050379
- Shand CA, Cheshire MV, Smith S, Vidal M, Rauret G 1994: Distribution of radiocaesium in organic soils. J. Environ. Radioact., 23, 285–302. doi:10.1016/0265-931X(94)90067-1
- Shoji S, Ito T, Saigusa M, Yamada I 1985: Properties of nonallophanic Andosols from Japan. Soil Sci., 140, 264–277. doi:10.1097/00010694-198510000-00005
- Sigurgeirsson MA, Arnalds O, Palsson SE, Howard BJ, Gudnason K 2005: Radiocaesium fallout behaviour in volcanic soils in Iceland. J. Environ. Radioact., 79, 39–53. doi:10.1016/j.jenvrad.2004.05.014
- Soil Survey Staff 2006: Keys to Soil Taxonomy, 10th edn. United States Department of Agriculture Natural Resources Conservation Service, Washington.
- Staunton S, Dumat C, Zsolnay A 2002: Possible role of organic matter in radiocaesium adsorption in soils. J. Environ. Radioact., 58, 163–173. doi:10.1016/S0265-931X(01)00064-9
- Takahashi M, Ishizuka S, Ugawa S, Sakai Y, Sakai H, Ono K, Hashimoto S, Matsuura Y, Morisada K 2010: Carbon stock in litter, deadwood and soil in Japan’s forest sector and its comparison with carbon stock in agricultural soils. Soil Sci. Plant Nutr., 56, 19–30. doi:10.1111/j.1747-0765.2009.00425.x
- Takenaka C, Onda Y, Hamajima Y 1998: Distribution of cesium-137 in Japanese forest soils: Correlation with the contents of organic carbon. Sci. Total Environ., 222, 193–199. doi:10.1016/S0048-9697(98)00305-2
- Thompson ML, Ukrainczyk L 2002: Micas. In Soil mineralogy with environmental applications, Ed. Dixon JB, Schultz DG, pp. 431–466. Soil Science Society of America, Madison, WI.
- Vandebroek L, Van Hees M, Delvaux B, Spaargaren O, Thiry Y 2012: Relevance of Radiocaesium Interception Potential (RIP) on a worldwide scale to assess soil vulnerability to 137Cs contamination. J. Environ. Radioact., 104, 87–93. doi:10.1016/j.jenvrad.2011.09.002
- Yagasaki Y, Mulder J, Okazaki M 2006: Comparing the activity of aluminum in two B horizons developed from volcanic ash deposits in Japan, dominated by short-range ordered aluminosilicates and crystalline clay minerals, respectively. Geochim. Cosmochim. Acta, 70, 147–163. doi:10.1016/j.gca.2005.08.026
- Yamaguchi N, Eguchi S, Fujiwara H, Hayashi K, Tsukada H 2012: Radiocesium and radioiodine in soil particles agitated by agricultural practices: Field observation after the Fukushima nuclear accident. Sci. Total Environ., 425, 128–134. doi:10.1016/j.scitotenv.2012.02.037
- Yasunari TJ, Stohl A, Hayano RS, Burkhart JF, Eckhardt S, Yasunari T 2011: Cesium-137 deposition and contamination of Japanese soils due to the Fukushima nuclear accident. PNAS, 108, 19530–19534. doi:10.1073/pnas.1112058108