Abstract
Animal manure contains significant amounts of nitrogen (N) and phosphorus (P) that can be utilized as fertilizer. However, manure application rates are generally set to meet crop N demand, which results in excess P application which increases the risk of P loss into bodies of water. We examined the application method of manure compost based on crop P demand on the dry matter yield of silage corn (Zea mays L. var. indentata), on nutrient uptake and on soil P accumulation in an upland Andosol with a high P-fixing capacity. Cattle and poultry manure composts (CMC and PMC) with different P solubility were applied to meet the crop’s N requirements (N-based application) or P requirements (P-based application) in 3 consecutive years. Supplemental N was applied using polyolefin-coated urea in the P-based treatments. The dry matter yields and nutrient uptakes of silage corn in the P-based CMC and PMC application systems were similar to those in the corresponding N-based systems. The average relative efficiency of N in CMC (33%) was close to the predicted value (30%) during the study. On the other hand, the relative efficiency of N in PMC (42%) was higher than the predicted value (28%). The average relative efficiency of P from CMC and PMC was 109% and 50%, respectively. These were higher than the predicted values based on the available P proportion (the sum of water- and sodium bicarbonate-soluble P) for CMC (78%) and PMC (34%), indicating that the available P fraction in the manure composts was more effective than that in P fertilizer for corn growth or that the other P fractions were also effective for corn. P-based manure compost application lowered the soil P accumulation to between 41 and 43% of the value in the N-based accumulation (versus a value of between 31 and 40% of that value for soil Truog-P). Compared to N-based manure compost application, P-based manure compost application with supplemental N fertilizer produced similar dry matter yields of corn and suppressed soil P accumulation in the upland Andosol field.
INTRODUCTION
The quantity of animal manure discharged annually in Japan has reached about 8.4 × 107 Mg fresh weight (MAFF Citation2011). Mishima et al. (Citation2009) showed that the animal manure contained 6.8 × 105 Mg of nitrogen (N) and 1.2 × 105 Mg of phosphorus (P). It is very important to utilize this animal manure as fertilizer or as the raw material to produce fertilizer because Japan has limited natural fertilizer resources.
On the other hand, N and P inputs to agricultural land that exceed the amounts that can be taken up by crops present a serious environmental concern. Mishima et al. (Citation2010) showed that the P surplus was larger than the N surplus in Japan. As a result, the concentration of available P in agricultural soil has been increasing (Obara and Nakai Citation2004). Mishima et al. (Citation2010) calculated that 20% of the P applied to agricultural land was derived from animal manure, and that vegetable crops in Japan received especially large amounts of P (122 kg P ha−1 year−1) through animal manure amendments. Livestock manure therefore contributed significantly to the observed soil P accumulation. Sharpley et al. (Citation2004) surveyed the total phosphorus (TP) concentrations in soils with and without manure addition in 20 agricultural fields in the United States, and found that the manure-amended soils contained more P (2480 mg P kg−1 on average) than the soils without manure (407 mg P kg−1). This P accumulation in agricultural soils can increase the potential risk of P losses into lakes and streams and can accelerate their eutrophication (Carpenter et al. Citation1998).
Most animal manure in Japan is composted to reduce its carbon (C)/N ratio, decompose toxic substances, kill weed seeds and pathogens, and improve handling of the manure. During composting, some N is lost through ammonia volatilization, but P loss is rare. As a result, animal manure compost usually has a lower N/P ratio than the raw manure. From a survey of the chemical properties of livestock manure composts throughout Japan (LIEO Citation2007), the N/P weight ratios of composts made from dairy cattle, beef cattle, swine, layer and broiler manures averaged 2.4, 1.9, 1.4, 1.1 and 2.1, respectively. These ratios are much smaller than those defined based on crop needs. The N/P ratios for the grains of wheat (Triticum aestivum L.), corn (Zea mays L.) and sorghum (Sorghum bicolor L.) were 4.5, 5.9, and 4.5, respectively (Gilbertson et al. Citation1979). The difference in the N/P ratio between the manure composts and crop needs results in excess P input to crop needs when composts are applied to meet crop N requirements (hereafter, N-based application). Phosphorus-based manure compost application determines the compost requirements based on the crop’s P needs, but because of the low N/P ratios, supplemental inorganic N fertilizer is required. Nonetheless, this approach can effectively control P buildup in agricultural soils while maintaining crop yields (Eghball and Power Citation1999; Toth et al. Citation2006). Eghball and Power (Citation1999) showed that P-based manure or compost application with supplemental N fertilizer resulted in soil-test P levels similar to the original soil P levels after 4 years of cultivation, and produced corn yields similar to those produced by inorganic fertilizers in all 4 years in an Argiudoll soil. The P solubility or availability in manure or manure compost is the key for defining P-based compost management, but this property varies among manure types and composting methods (Ito et al. Citation2010). The fractionation method of Frossard et al. (Citation1994) has been widely used for evaluating P characteristics in manure compost. Frossard et al. (Citation1994) modified the soil P fractionation method developed by Hedley et al. (Citation1982) for the evaluation of P in organic matter. The procedure involves sequential extraction by water (H2O), sodium bicarbonate (NaHCO3), sodium hydroxide (NaOH) and hydrochloric acid (HCl). Turner and Leytem (Citation2004) showed that H2O and NaHCO3-soluble P in manure composts were labile and available for plants. In addition, Komiyama et al. (Citation2014) demonstrated that NaHCO3-extractable P was dissolved slowly by large amounts of water, and the maximum amounts of water-extractable P were able to be estimated by the sum of H2O- and NaHCO3-soluble P. Ito et al. (Citation2010) showed that the ratio of the sum of H2O- and NaHCO3-soluble P to total P in dairy cattle, beef cattle, swine, layer and broiler manure compost was 65, 72, 49, 30 and 31%, respectively, and the ratio of NaOH-soluble P, which is assumed to be iron and aluminum-bound P, was less than 10% in all types of compost. The HCl-soluble P in animal manure compost, which is assumed to be calcium phosphate with a high calcium (Ca)/P ratio, is estimated to be very low in solubility to water and bioavailability for a short period. Komiyama et al. (Citation2013) demonstrated that the acid-soluble P with low water solubility in manure compost was not available for Japanese mustard spinach (Komatsuna; Brassica rapa L. var. perviridis) by the cultivation experiment. Sharpley et al. (Citation2004) surveyed P characteristics of manure-applied soils, and showed that HCl-soluble P is accumulated even in non-calcareous soils. From the results of these studies, it seems to be appropriate to regard labile P, the sum of H2O- and NaHCO3-extractable P, as a plant-available P. There is no study which examined the effects of the application of manure composts based on labile P contents of them on crop yield and soil P accumulation.
Andosols have a high P-fixing capacity, which are derived from volcanic ash, and a major soil type in upland agricultural fields in Japan. The bioavailability of P in inorganic fertilizer and manure composts varies among soil types (Indiati et al. Citation1995; Sharpley and Sisak Citation1997). It is not clear whether P-based manure compost application can maintain high crop yields in Andosols while preventing the accumulation of soil P. In this study, we investigated the effects of P-based manure compost application on the yield of silage corn (Zea mays L.) and on the accumulation of soil P in upland Andosols using two manure composts with different P solubility.
MATERIALS AND METHODS
Soil properties in the experimental field
A field experiment was conducted for 3 years (2004, 2005 and 2006) at the Field Science Center, Graduate School of Tohoku University, Japan (38°74′50”N, 140°75′74”E). The soil was a Melanic Aluandic Andosol in the taxonomy of the IUSS (International Union of Soil Sciences) Working Group WRB (World Reference Base for Soils) (Citation2006). This soil showed a high P-fixing capacity and a large amount of organic matter. The plow layer of the soil (to a depth of 15 cm) had a pH of 5.9 (in water), a soil-test P (Truog-P; Truog Citation1930) of 57 mg P kg−1 and a cation exchange capacity of 38.2 cmol kg−1. The critical value of Truog-P needed for maintaining crop productivity is 44 mg P kg−1 (100 mg P2O5 kg−1) for agricultural upland Andosols based on the Soil Fertility Enhancement Act in Japan. The TP content, which was measured using the method of Legg and Black (Citation1955), was 1.93 g P kg−1. In the methods, TP was determined by measuring colorimetrically the P concentration of the solution extracted by hot hydrochloric acid after combusting the soil. The large difference between the Truog-P and TP contents is due to the large amount of active aluminum (Al) and the high P-fixing capacity of these soils. The phosphate adsorption coefficient, which is widely used to define a soil’s P-fixing capacity in Japan, and determined with addition of 11.7 g P kg−1 soil, was 8.6 g P kg−1 for the soil. The acid–ammonium oxalate extractable Al (Alo) and the pyrophosphate-extractable Al (Alp) (Blackmore et al. Citation1981) were 22.1 and 14.4 g kg−1, respectively. The high ratio of Alp to Alo means that Al-humus complexes represent the major source of active Al in this soil.
Animal manure composts
The cattle manure compost (CMC) used in this study was produced at the Field Science Center, Graduate School of Agriculture, Tohoku University. Fresh mixed beef and dairy cattle manure was composted at an open-type high-rate composting facility, with the addition of sawdust as an amendment to control the moisture content. The sawdust seems to add negligible amounts of nutrients to the compost. The poultry manure compost (PMC) was produced at a layer farm in Miyagi Prefecture, Japan. The PMC was composted with no additives and with intermittent turning.
Manure compost analysis and evaluation of available nutrients
Before the field experiment each year, we measured the available N and P concentrations of the manure composts to determine the required application rates. The total nitrogen (TN) content was determined by dry combustion using an NC-80S NC analyzer (Sumika Chemical Analysis Service, Ltd., Tokyo, Japan). After wet digestion of the compost samples with a nitric acid-perchloric acid mixture (Page et al. Citation1982), the P in the solution was measured colorimetrically using the molybdenum blue method (Murphy and Riley Citation1962) and was used as the TP concentration. The potassium (K) concentration in the digested solution was measured by atomic absorption spectrometry (Z6000; Hitachi, Tokyo, Japan). The available N was estimated according to the recommendation of NGRI (National Grassland Research Institute) (Citation1983) for CMC and according to the results of Tanahashi and Yano (Citation2004) for PMC. NGRI (Citation1983) showed that the N mineralization rate of CMC was 30% of TN. Tanahashi and Yano (Citation2004) recommended that available N in PMC could be estimated by the sum of quickly mineralized N (4 weeks incubation at 30°C) calculated with the equation below and the amount of N mineralized slowly regardless of TN content (3g-N kg−1):
Based on these previous studies, the available N in the CMC and PMC used in this study averaged 30 and 28% of TN, respectively, during the 3 years of our study (). We used the modified method of Hedley et al. (Citation1982) to characterize the P fractionation in the manure composts. In the fractionation method (Frossard et al. Citation1994), we sequentially extracted the manure composts with H2O (1:200 weight/volume), 0.5 mol L−1 NaHCO3 (pH = 8.5), 0.1 mol L−1 NaOH and 1 mol L−1 HCl. After each extraction, which was conducted with continuous shaking for 16 h at 25°C, the P concentration of the supernatant was measured colorimetrically (Murphy and Riley Citation1962). In this study, we considered the sum of H2O- and NaHCO3-extractable P to be the available P for crops according to the results of Turner and Leytem (Citation2004) and Komiyama et al. (Citation2014).
Table 1 Characteristics of the CMC and PMC used in the field experiment
Field experiment
We conducted a field cultivation experiment with six treatments: (1) N-based CMC application (N-CMC), (2) P-based CMC application (P-CMC), (3) N-based PMC application (N-PMC), (4) P-based PMC application (P-PMC), (5) inorganic fertilizer (N-P-K = 150-65.5-62 kg ha−1; N-P2O5-K2O = 150-150-150 kg ha−1) (IF) and (6) no compost or inorganic fertilizer (the control). The standard application rates of N and K were based on a previous study conducted in a nearby field (Inoue et al. Citation2000). We lowered the P application rate from 87.3 kg P ha−1 (200 kg P2O5 ha−1) to 65.5 kg P ha−1 because the available P in soil was more than the critical value (44 mg P kg−1). In the N-based treatments, manure composts were applied so that the available N derived from the composts would be 150 kg N ha−1 (). In other words, inorganic N was substituted by compost N completely in N-based treatments (N substitution rate: 100%). The annual compost application rates on a dry matter basis averaged 18.1 and 21.4 Mg ha−1 across 3 years in the N-CMC and N-PMC treatments, respectively. In the P-based treatments, manure composts were applied so that the available P derived from the composts would be 65.5 kg P ha−1 (P substitution rate: 100%). The annual compost application rates averaged 7.7 and 8.1 Mg ha−1 across the 3 years in P-CMC and P-PMC, respectively. The application rates of compost in the P-based treatments were less than half of those in the N-based treatments. Because the available N from the manure composts did not reach 150 kg N ha−1 in the P-based treatments, supplemental inorganic fertilizer was provided using polyolefin-coated urea (PCU). Two types of slow-release PCU (JCAM AGRI. Co. Ltd., Tokyo) were used: one with 80% dissolution of the total N after 70 days and the other with 80% dissolution after 40 days, both at 25°C. These were blended at a ratio of 2:1 weight/weight. In the IF treatment, the PCU mixture, double superphosphate and potassium chloride were used as the N, P and K sources, respectively.
Table 2 Application rates of the manure composts and inorganic fertilizer, and the associated available N and available P
Manure compost and fertilizer were scattered on the fields and incorporated into the plow layer by rototilling just before sowing. Each treatment had three replicates, in the same field, that each covered 70 m2 (length: 7 m north to south, width: 10 m east to west), in a randomized complete block design. The same plots were used for each treatment in each year.
Silage corn (Zea mays L. cv. Pioneer32K61) was planted at a rate of 71,000 seeds ha−1, with a row spacing of 0.75 m in all years. The planting dates were May 19, 2004, May 25, 2005, and May 15, 2006. Herbicide was sprayed before the corn germinated each year. Corn was harvested on August 20, 2004, September 13, 2005, and September 4, 2006. Lodging of the corn occurred in 2004 by the influence of a typhoon, so the plants were harvested at the dough-ripe stage, a few weeks earlier than the ideal harvest time. In 2005 and 2006, corn was harvested at the yellow-ripe stage, the proper stage of harvesting for silage corn. To determine yields, we collected 40 plants from each plot (at two places in each plot) and measured the fresh weights of each plant after dividing the aboveground biomass into stover and ear. Four plants whose fresh weights were closest to the average values in each plot were selected and dried at 72°C to determine the dry weight ratio. The dry matter yield in each plot was then calculated by multiplying the fresh weight of the 40 plants by the dry weight ratios determined using the four oven-dried plant samples.
Analysis of plants and soil
The four oven-dried plants (divided into ear and stover) were ground and analyzed to determine TN and TP. TN was determined by the dry combustion method using the NC-80S NC analyzer. TP was determined colorimetrically after Sulfuric acid - Hydrogen peroxide (H2SO4-H2O2) digestion (Minami and Mizuno, Citation1980) . We collected soil samples from the plow layer (to a depth of 15 cm) at five places in each plot, and composited them into a single sample to determine the changes in soil nutrients after 3 years of cultivation; this was done before compost or fertilizer application in 2004 and after harvesting in 2006. The samples were air-dried and passed through a 2-mm mesh, but without grinding to exclude coarse particles. The available P was determined as Truog-P (Truog Citation1930). The total inorganic P content was measured colorimetrically after extracting the soil with hot hydrochloric acid. The total organic P content was determined by subtracting total inorganic P from TP (Legg and Black Citation1955). The amount of total inorganic P, total organic P and Truog-P changed by the applications of manure or fertilizer was calculated from the difference of those contents before and after 3 years’ cultivation in each treatment (the average of three replications).
Apparent nutrient recovery and relative efficiency
The apparent N and P recovery rates in the manure compost and inorganic fertilizer treatments were calculated as follows:
The relative efficiency of the nutrients in the manure composts was calculated as follows:
Statistical analysis
We tested for significant differences among the treatments in the means for silage corn yield and nutrient uptake using two-way analysis of variance (ANOVA) followed by Tukey’s honestly significant difference (HSD). We used the same test for soil P concentrations (Tukey’s HSD). All analysis was conducted using version 4.0.5.J of the JMP software (SAS Institute Citation2001).
RESULTS
Characteristics of the animal manure composts
The chemical properties of CMC and PMC used in this study did not differ greatly during the 3 years (). LEIO (Citation2007) surveyed a large number of manure composts in Japan and showed the average nutrient content. According to the results, the averaged TN content of CMC and PMC was 22 and 29 g kg−1, respectively. The TN of CMC used in this study was a little bit higher than the national average, whereas that of PMC was the same as the average. The TP contents of both of composts were similar to the national averages. The TN and available N did not differ between the two composts. However, TP in PMC was more than twice the corresponding value in CMC in all 3 years. The ratio of available P to TP was lower in PMC (34%) than in CMC (78%) because the layer in PMC contained more Ca, which forms insoluble calcium phosphate and decreases the solubility of P (Ito et al. Citation2010). As a result, the available P did not differ greatly between the composts.
Dry matter yield of silage corn
The dry matter yields of silage corn (the sum of ear and stover weights) were affected both by the year and by the fertilizer treatment (). The dry matter yield of silage corn was highest in 2005 in all treatments. The cumulative air temperatures during plant growth (between sowing of seeds and harvesting) were 1916, 2287 and 2217°C in 2004, 2005 and 2006, respectively. Precipitation totaled 560, 720 and 655 mm, respectively. Sunshine hours during this period were 421, 375 and 359 hours, respectively. Because the harvest was moved forward in 2004 because of lodging of the corn caused by a typhoon, the dry matter yield was lowest in this year in most treatments. There was no significant difference in the dry matter yield between the four compost treatments in all years. The two CMC treatments (N-CMC and P-CMC) produced higher yields than the IF treatment in all years, but the differences were not significant. The two PMC treatments (N-PMC and P-PMC) also increased the dry matter yield compared to the IF treatment, but the difference was only significant for N-PMC in 2005 and 2006.
Table 3 Total dry matter yield of silage corn (ear and stover) and the nitrogen (N) and phosphorus (P) uptake
N and P uptake by corn
N uptake by the corn differed significantly (P < 0.001) among the years and treatments (). N uptake was lowest in 2006 in all treatments. Total N uptake was greatest in N-PMC in all years, followed by P-PMC, but the differences were not significant. N uptakes in the N-CMC and P-CMC treatments were similar to those in the IF treatment in all 3 years.
P uptake was also highest in 2005, followed by 2004 and 2006, in all treatments. P uptake in all of the compost treatments exceeded that in the IF treatment in all years. In particular, P uptake in N-PMC in 2005 and N-CMC in 2006 were significantly (P < 0.05) higher than those in IF. There was no significant difference in P uptake among the four compost treatments, except for a significantly higher value in N-PMC than in P-CMC in 2005.
In the control, N and P uptake decreased greatly in 2006, and this may explain the smallest yield in that year. The tendency of the yield to decline during continuous cultivation may be due to a decrease in soil fertility in terms of available N or P. Inoue et al. (Citation2000) also showed that the dry matter yields of silage corn decreased after 4 years of continuous cultivation with conventional fertilization in an Andosol field near our study site. In the P-based treatments (P-CMC and P-PMC), both N and P uptake did not differ significantly from those in the IF treatment in all 3 years.
shows the N and P recovery rates and the relative efficiency calculated from the amounts of total nutrients input and uptake for 3 years. In this study, the recovery and efficiency values were averaged over 3 years (Eq. 2 and 3). For N uptake, the apparent recovery and relative efficiency were calculated using only the results of the N-based treatments (N-CMC and N-PMC) because the P-based treatments received both manure N and inorganic fertilizer N. The apparent N recovery rates from N-CMC, N-PMC and inorganic fertilizer were 11, 14 and 35%, respectively. The relative efficiencies of N from CMC and PMC were 33 and 42%, respectively. We calculated the relative efficiency of P from compost using the data from the P-based treatments because the P application rate was closer to that in the inorganic fertilizer. The relative efficiency averaged 109 and 50% through the 3 years of the study for CMC and PMC, respectively.
Table 4 Total amounts of N and P application and uptake, N and P recovery rates and relative efficiency for 3 years
Soil P accumulation
Soil P accumulation was evaluated from the changes in TP and Truog-P. shows the changes in total organic and inorganic P in the plow layer after 3 years of cultivation. TP content in the plow layer in N-PMC was significantly highest of all treatments. TP content in N-CMC was significantly higher than those in P-CMC, IF and control treatments. TP increased compared with the initial value (1.93 g P kg−1) in P-CMC (+0.17 g P kg−1), but was only 41% of the increase in N-CMC (+0.41 g P kg−1). TP also increased compared with the initial value in P-PMC (+0.40 g P kg−1), and was only 43% of the increase in N-PMC (+0.94 g P kg−1). TP change in IF and Control treatments were +0.20 g P kg−1 and –0.03 g P kg−1, respectively.
Figure 1 Total organic and inorganic phosphorus (P) contents in the plow layer soil (to a depth of 15 cm) after cultivation in 2006. Values are means ± standard deviations for total P. Values labeled with the same letter did not differ significantly (Tukey’s test, P < 0.05). Capital letters represent the differences in total P; lower-case letters represent the differences in total organic and total inorganic P.
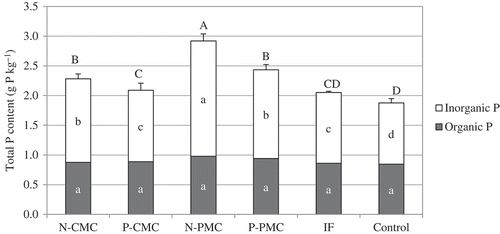
The concentration of Truog-P in the plow layer was also increased by the continuous application of the manure composts (). The change in Truog-P (the difference between the value after cultivation in 2006 and the initial value (44 mg P kg−1) in 2004) was +54 mg P kg−1 in N-CMC, +17 mg P kg−1 in P-CMC, +150 mg P kg−1 in N-PMC, +60 mg P kg−1 in P-PMC, +29 mg P kg−1 in IF and +2 mg P kg−1 in the control treatment. P-based management therefore limited the increase in Truog-P to 31 to 40% of the increase in the corresponding N-based management.
Figure 2 Soil-test phosphorus (Truog-P) content in the plow layer soil (to a depth of 15 cm) after cultivation in 2006. Values are means ± standard deviations. Bars labeled with different letters differ significantly (Tukey’s test, P < 0.05).
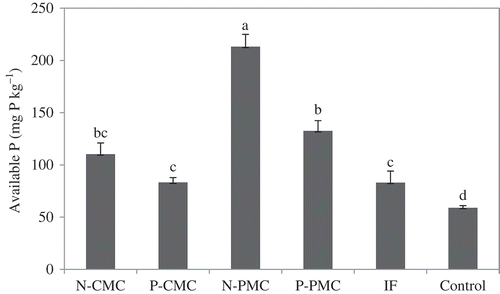
We determined the P budget in the experimental fields using the difference between the total P input from compost or fertilizer and the total P withdrawal by crop uptake shown in . For the 3 years of our study, the budget totaled 504 kg P ha−1 for N-CMC, 162 kg P ha−1 for P-CMC, 1561 kg P ha−1 for N-PMC, 526 kg P ha−1 for P-PMC, 111 kg P ha−1 for IF and –73 kg P ha−1 for the control. There was a strong relationship between the P budget and the increase of TP content in plow layer soil (). The increase in Truog-P was also strongly related to the P budget (R2 = 0.989). The P accumulations in P-based treatments were less because of a lower input of P compared with the N-based treatments.
Figure 3 Relationship between the phosphorus (P) budget (total applied P – total P uptake) and the increase in TP content in the plow layer soil (to a depth of 15 cm) after 3 years of continuous cultivation. Values are means ± standard deviations for the increase in total P.
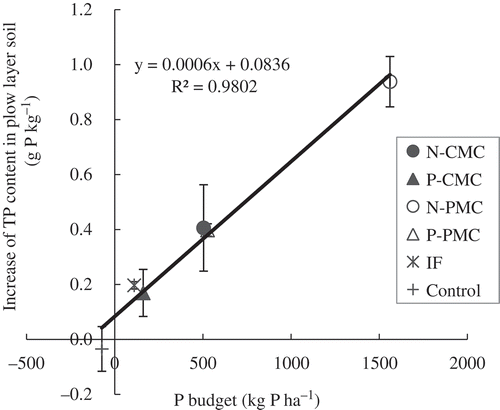
DISCUSSION
Productivity of silage corn in P-based management
The dry matter yield was highest in 2005 because the climate conditions appear to have been best during the growing period. However, the tendency of the yield between the treatments was same in all 3 years. Based on the results of dry matter yield and nutrient uptakes, P-based manure compost application with supplemental N fertilizer can supply adequate amounts of N and P and produce yields of silage corn similar to those obtained with N-based manure compost application or inorganic fertilizer application in the Andosols of the study area ().
Availability of N and P in compost
The relative efficiency of N from CMC (33%, ) was similar to the available N rate (30% of TN) that we assumed in this study. Muñoz et al. (Citation2004) reported a 32% relative efficiency of N from dairy cattle manure for corn in a field experiment. Eghball and Power (Citation1999) estimated the apparent N recovery and relative efficiency from beef CMC (4-year averages) as 15 and 30%, respectively, in an N-based application. The C/N ratios of the CMC used in the present study (11–12) were similar to those (9–11) in the study of Eghball and Power (Citation1999). On the other hand, the relative efficiency of N uptake from PMC (42%, ) was higher than the estimated mineralization rate (a 3-year average of 28% of TN). Tanahashi and Yano (Citation2004) estimated the available N of compost based on the relationship between TN content and mineralized N rate in PMC. For the regression (n = 32), some PMCs may deviate from the regression line. The N mineralization rates of the composts were estimated based on the incubation experiment, where temperature and soil moisture was maintained constantly. The evaluation error for the regression analysis and differences in decomposition of composts between in field and in the incubation experiment may partly explain the underestimation of the available N contents for PMC in our field experiment.
The relative efficiencies of P () were higher than the available P fraction (the sum of the H2O- and NaHCO3-extractable P) for the composts (78% for CMC, 34% for PMC), suggesting that the available P fraction of the manure compost was absorbed more effectively by the corn than the P in inorganic fertilizer, or that the other P fractions with lower solubility (NaOH-extractable P, HCl-extractable P and organic P) were also utilized. Oyanagi et al. (Citation2005) also reported that the efficiencies of P in manure composts were higher than superphosphate or fused magnesium phosphate. Kato et al. (Citation2010) showed that P derived from animal manure composts was absorbed more slowly than the readily soluble P from inorganic fertilizer. This result suggests that corn can take up the available P fraction of the manure compost more effectively than the P in inorganic fertilizer. However, it will be necessary to examine the availability of the less-soluble compost P fractions for crops in future research.
The K content of CMC used in this study () was higher than the national average (23 g K kg−1) because urine was not separated in the composting operation. In this study, the K content was not taken into account in the experimental design. In some cases, it may be necessary to apply animal manure composts to meet the K requirement of crops to avoid grass tetany syndrome in the livestock.
Phosphorus accumulation in the soil
The P-based management effectively controlled the increase of TP accumulation in the plow layer (41~43% of that in the N-based). The TP contents of the soil were higher in the PMC plots than in the CMC plots in 2006, after cultivation (). In the N-based application, more P was supplied by the N-PMC than by the N-CMC because the ratio of TP to available N was higher in PMC (). In the P-based application, the higher TP concentration (25.8 g P kg−1 on average) and the lower percentage of available P (34%) in the PMC resulted in greater P accumulation in the P-PMC plots than in the P-CMC plots (). The organic P content did not change significantly after 3 years of cultivation in all treatments (). Soil P accumulation caused by the manure compost applications was therefore derived mainly from increased inorganic P (). Hirata et al. (Citation1999) and Gale et al. (Citation2000) also reported an increase in inorganic P concentrations in the soil after continuous application of manures or composts. The inorganic P was 87% of TP in CMC and 83% of TP in PMC. The present results and those from previous studies indicate that inorganic P is the main fraction that accumulates as a result of the application of manure composts.
The strong and positive relationship between the P budget and the increase of TP content in the plow layer soil indicates that the amount of P budget influenced the P accumulation level in the plow layer (to a depth of 15 cm). To discuss the amount of accumulated P at the field scale, the P accumulation in the plow layer and subsoil should be examined as Tani et al. (Citation2011) showed in upland low-humic Andosols. However, in this study, TP in the plow layer was examined from the viewpoint of assessing the risk of P runoff by soil erosion. The buildup of TP in the surface soil increases the risk of P runoff into bodies of water. For CMC and PMC, the budgets were 342 and 1034 kg P ha−1 lower in the P-based application than in the N-based application, but these budgets were still 51 and 416 kg P ha−1 higher than that for inorganic fertilizer (). This suggests that monitoring will be required after subsequent crops to ensure that excessive P accumulation does not occur. The Truog-P value in the P-PMC treatment was 2.5–3 times the value in the P-CMC treatment even though the same amount of available P derived from the composts was applied in both treatments (). One reason for the much larger increase in Truog-P in the P-PMC plot is the possibility that the insoluble P derived from PMC (the HCl-extractable P) was partly dissolved by the acidic Truog solution.
An increase in Truog-P as a result of continuous application of manures or composts has been reported in some previous studies in Japan [Ito et al. Citation1982 (CMC, Andosol); Ohashi and Okamoto Citation1985 (cattle manure compost mixed with sawdust, Gleysols); Kaji et al. Citation1990 (several manures, Andosol); Taki et al. Citation2006 (CMC, Fluvisol); Murakami and Kuroyanagi Citation2009 (several animal manure composts, Andosol); Kasuya et al. Citation2011 (swine manure compost, Cambisol)]. Kanazawa (Citation2009) surveyed the average application rate for CMC and found application rates of 17 Mg ha−1 for Japanese radish (Raphanus sativus L. var. longipinnatus) (autumn harvest; n = 15), 24 Mg ha−1 for Chinese cabbage (Brassica rapa L. var. pekinensis) (autumn harvest; n = 17), 20 Mg ha−1 for sweet corn (Zea mays L. var. saccharata) (n = 20), 20 Mg ha−1 for cabbage (Brassica oleracea L. var. capitata) (spring harvest; n = 17), 29 Mg ha−1 for sorghum (n = 9), 48 Mg ha−1 for grass (n = 5) and 21 Mg ha−1 for silage corn (n = 9). Based on the results of these previous studies and our study, it is clear that the application of animal manure composts causes soil P enrichment when the composts are applied at the Japanese standard rates (Kanazawa Citation2009) with conventional inorganic P fertilizer. However, few studies have proposed application methods for manure composts to control available P in the Andic soil. In the present study, we clarified that a P-based application system was effective in controlling the P accumulation of soil and producing silage corn yields similar to those with an N-based application system in an upland Andosol. However, even the P-based application gradually increased the total and available P contents, especially for PMC. To further reduce the accumulation of soil P, it is therefore necessary to clarify the availability of the low-solubility compost P fractions (e.g., the HCl-extractable P) and to develop a P-based compost management system that accounts for this less-soluble P fraction in the composts.
CONCLUSIONS
We examined the effects of labile P-based manure compost application using two types of manure composts with different P solubility on the dry matter yield of silage corn and P accumulation in an upland Andosol in Japan. The dry matter yield and the N and P uptake of silage corn in the P-based compost treatments did not differ significantly from those in the N-based compost treatments and in conventional fertilization over 3 years. However, after 3 years of corn cultivation, the P-based manure compost application system reduced the accumulation of soil P to between 41 and 43% of the value in the N-based system (versus values of between 31 and 40% of that value for soil Truog-P). Thus, P-based manure compost application with supplemental N fertilizer can maintain corn silage yields at levels comparable to those achieved with N-based manure compost application and inorganic fertilizer, while repressing abrupt soil P accumulation in upland Andosols.
REFERENCES
- Blackmore LC, Searle PL, Daly BK 1981: Methods for Chemical Analysis of Soils, New Zealand Soil Bureau Scientific Report 10 A. Department of Scientific and Industrial Research (DSIR), Wellington, NZ.
- Carpenter SR, Caraco NF, Correll DL, Howarth RW, Sharpley AN, Smith VH 1998: Nonpoint pollution of surface waters with phosphorus and nitrogen. Ecol. Applic., 8, 559–568. doi:10.1890/1051-0761(1998)008[0559:NPOSWW]2.0.CO;2
- Eghball B, Power JF 1999: Phosphorus- and nitrogen-based manure and compost applications corn production and soil phosphorus. Soil Sci. Soc. Am. J., 63, 895–901. doi:10.2136/sssaj1999.634895x
- Frossard E, Tekely P, Grimal JY 1994: Characterization of phosphate species in urban sewage sludges by high-resolution solid-state 31P-NMR. Eur. J. Soil. Sci., 45, 403–408. doi:10.1111/j.1365-2389.1994.tb00525.x
- Gale PM, Mullen MD, Cieslik C, Tyler DD, Duck BN, Kirchner M, McClure J 2000: Phosphorus distribution and availability in response to dairy manure applications. Commun. Soil Sci. Plant Anal., 31, 553–565. doi:10.1080/00103620009370459
- Gilbertson CB, Norstadt FA, Mathers AC et al. 1979: Animal waste utilization on cropland and pastureland: a manual for evaluating agronomic and environmental effects. Utilization Research Report no. 6, USDA, Washington, DC.
- Hedley MJ, Stewart JWB, Chauhan BS 1982: Changes in inorganic and organic soil phosphorus fractions induced by cultivation practices and by laboratory incubations. Soil Sci. Soc. Am. J., 46, 970–976. doi:10.2136/sssaj1982.03615995004600050017x
- Hirata H, Watanabe K, Fukushima K, Aoki M, Imamura R, Takahashi M 1999: Effect of continuous application of farmyard manure and inorganic fertilizer for 9 years on changes in phosphorus compounds in plow layer of an upland Andosol. Soil Sci. Plant Nutr., 45, 577–590. doi:10.1080/00380768.1999.10415821
- Indiati R, Sharpley AN, Izza C, Figliolia A, Felici B, Sequi P 1995: Soil phosphorus sorption and availability as a function of high phosphorus fertilizer additions. Commun. Soil Sci. Plant Anal., 26, 1863–1872. doi:10.1080/00103629509369413
- Inoue H, Ito T, Saigusa M 2000: Yield and uptake of dent corn (Zea mays L.) in no-tillage cultivation with single basal co-situs fertilization. Jpn. J. Soil Sci. Plant Nutr., 71, 674–681. (in Japanese with English summary).
- Ito T, Komiyama T, Saigusa M, Morioka M 2010: Phosphate composition of swine and poultry manure composts. Jpn. J. Soil Sci. Plant Nutr., 81, 215–223. (in Japanese with English summary).
- Ito Y, Shiozaki H, Hashimoto H 1982: Effects of continuous heavy-application of farmyard manure on the fertility of a humus-rich volcanic ash soil. Bull. Kyusyu Agric. Exp. Sta., 22, 259–320. (in Japanese with English summary).
- IUSS Working Group WRB 2006: World reference base for soil resources 2006. 2nd edition. World Soil Resources Reports No. 103. FAO, Rome.
- Kaji T, Ikeda K, Kusamizu T 1990: Effect of successive application on various animal manures on the yield of crop plants and chemical property of soil. Bull. Kagoshima Agric. Exp. Stn., 18, 33–50. (In Japanese with English summary).
- Kanazawa K 2009: Usage of two databases, one containing prefectural recommendations of fertilizers and manure application, and the other containing nutrient contents of crops in Japan. Bull. Natl. Agric. Res. Cent., 12, 27–50. (In Japanese with English summary).
- Kasuya M, Ogino K, Hiroto S, Ishikawa H, Suzuki R 2011: Dynamics of nutrients in a yellow soil vegetable field using cattle or pig feces compost for five years. Res. Bull. Aichi Agric. Res. Ctr., 43, 137–149. (In Japanese with English summary).
- Kato M, Komiyama T, Fujisawa E, Morikuni H 2010: Repressions of adsorptions of available phosphate derived from fertilizer by combined applications with cow or poultry manure composts in upland soil conditions. Jpn. J. Soil Sci. Plant Nutr., 81, 367–371. (In Japanese).
- Komiyama T, Ito T, Saigusa M 2014: Measurement of the maximum amount of water-extractable phosphorus in animal manure compost by continuous and sequential water extraction. Soil Sci. Plant Nutr., 60, 196–207. doi:10.1080/00380768.2013.879836
- Komiyama T, Niizuma S, Fujisawa E, Morikuni H 2013: Phosphorus compounds and their solubility in swine manure compost. Soil Sci. Plant Nutri., 59, 419–426. doi:10.1080/00380768.2013.789397
- Legg JO, Black CA 1955: Determination of Organic Phosphorus in Soils: II. Ignition Method. Soil Sci. Soc. Am. Proc., 19, 139–142. doi:10.2136/sssaj1955.03615995001900020007x
- LIEO 2007: The Nutrient List and the Usage Method that Adopted Fertilizer Efficiency of Animal Manure Compost. Livestock Industry Environmental Organization, Tokyo.
- MAFF 2011: Status of Emission and Management of Livestock Manure. Ministry of Agriculture, Forestry and Fisheries, Tokyo. http://www.maff.go.jp/j/chikusan/kankyo/taisaku/t_mondai/02_kanri/index.html (in Japanese).
- Mishima S, Endo A, Kohyama K 2010: Nitrogen and phosphate balance on crop production in Japan on national and prefectural scales. Nutr. Cycl. Agroecosyst., 87, 159–173. doi:10.1007/s10705-009-9324-1
- Mishima S, Endo A, Shirato Y, Kimura DS 2009: Quantity of organic waste resources in Japan and capacity of local farmyard to receive composted wastes. Jpn. J. Soil Sci. Plant Nutr., 80, 226–232. (in Japanese with English summary).
- Minami M, Mizuno N 1980: The use of H2SO4-H2O2 for the destruction of plants matter as a preliminary to determination of N, K, Mg, Ca, Fe, Mn. Jpn. J. Soil Sci. Plant Nutr., 51, 418–420. (in Japanese).
- Muñoz GR, Kelling KA, Powell JM, Speth PE 2004: Comparison of estimates of first-year dairy manure nitrogen availability or recovery using nitrogen-15 and other techniques. J. Environ. Qual., 33, 719–727. doi:10.2134/jeq2004.7190
- Murakami H, Kuroyanagi Y 2009: Effects of animal manure application on soil environment in cabbage field. Bull. Natl. Inst. Veg. & Tea Sci., 8, 139–156. (in Japanese with English summary).
- Murphy J, Riley JP 1962: A modified single solution method for the determination of phosphate in natural waters. Anal. Chim. Acta., 27, 31–36. doi:10.1016/S0003-2670(00)88444-5
- NGRI 1983: The Report of the Study on the Processing and Use of Animal Feces and Urine, 60–61. National Grassland Research Institute, Tokyo. (in Japanese)
- Obara H, Nakai M 2004: Available phosphate of arable lands in Japan. changes of soil characteristics in Japanese arable lands (II). Jpn. J. Soil Sci. Plant Nutr., 75, 59–67. (in Japanese with English summary).
- Ohashi K, Okamoto M 1985: Effects of continuous application of farmyard manure mixed with sawdust on the nutrient uptake by vegetables and on the chemical properties of soil. Jpn. J. Soil Sci. Plant Nutr., 56, 378–383. (In Japanese).
- Oyanagi W, Wada T, Ando Y 2005: Property and fertilizer effect of phosphorus included animal waste compost. Bull. Niigata Animal Husbandry Exp. Sta., 15, 6–9. (In Japanese).
- Page AL, Miller RH, Keeney DR 1982: Methods of Soil Analysis Part 2—Chemical and Microbiological Properties, 2nd Edition, pp. 275–300. American Society of Agronomy, Madison, WI.
- SAS Institute Inc. 2001: JMP User’s Guide, Version 4.0. J. SAS Institute, Cary, NC.
- Sharpley AN, McDowell RW, Kleinman PJA 2004: Amounts, forms, solubility of phosphorus in soils receiving manure. Soil. Sci. Soc. Am. J., 68, 2048–2057. doi:10.2136/sssaj2004.2048
- Sharpley AN, Sisak I 1997: Differential availability of manure and inorganic sources of phosphorus in soil. Soil Sci. Soc. Am. J., 61, 1503–1508. doi:10.2136/sssaj1997.03615995006100050030x
- Taki N, Kumagai C, Hatanaka A 2006: Phosphorus accumulation after continuous application of animal manure compost to grey lowland soil of upland field. Bull. Miyagi Pref. Furukawa Agric. Exp. Sta., 6, 35–41. (in Japanese).
- Tanahashi T, Yano H 2004: The method of presuming the effect of nitrogen based on the nitrogen content of poultry manure compost. Jpn. J. Soil Sci. Plant Nutr., 75, 257–260. (in Japanese).
- Tani M, Mizota C, Yagi T, Fueki N, Tamura H, Kato T, Koike M 2011: Accumulated amount and formation of phosphate in cultivated upland low-humic Andosols through continuous application of inorganic fertilizers and cattle manure for 25-years. Jpn. J. Soil Sci. Plant Nutr., 82, 224–227. (in Japanese).
- Toth JD, Dou ZX, Ferguson JD, Galligan DT, Ramberg CF 2006: Nitrogen- vs. phosphorus-based dairy manure applications to field crops: nitrate and phosphorus leaching and soil phosphorus accumulation. J. Environ. Qual., 35, 2302–2312. doi:10.2134/jeq2005.0479
- Truog E 1930: The determination of the readily available phosphorus of soils. J. Am. Soc. Agron., 22, 874–882. doi:10.2134/agronj1930.00021962002200100008x
- Turner BL, Leytem AB 2004: Phosphorus compounds in sequential extracts of animal manures: chemical speciation and a novel fractionation procedure. Environ. Sci. Technol., 38, 6101–6108. doi:10.1021/es0493042