Abstract
The effect of soil exchangeable potassium (K) and cesium (Cs) levels on Cs uptake and accumulation in different parts of rice (Oryza sativa L.) plants were examined using paddy soils with diverse exchangeable K and Cs in pot experiments. Aboveground Cs uptake decreased with higher exchangeable K and was linearly correlated with exchangeable Cs/K ratios, indicating competitive absorption of these elements by roots. Variation in Cs concentration in brown rice among soils was also related to the exchangeable Cs/K ratio. The exchangeable Cs/K ratio was positively reflected in the Cs/K concentration ratio in each plant part, with a specific slope, suggesting that Cs transport was coordinated with K transport and that there were regulated discriminations of Cs against K in the translocation process among parts. The Cs/K ratio was higher in brown rice and dead leaves than in active leaves, stems and husks. The distribution of Cs accumulation in brown rice was 14.5% on average, but it was variable and negatively related to K concentration in the stem. The Cs distribution in aboveground plant parts also decreased with higher K concentration in the root. These results imply the importance of the competitiveness with K in the root absorption and translocation of Cs within the plant. Based on the observed relationship between Cs and K, effective K management and other measures to control Cs accumulation in plant parts are discussed.
INTRODUCTION
It is essential to understand the dynamics of cesium (Cs) in soil–plant systems to establish an effective measure to limit radioactive Cs accumulation in crops. Surveys in the areas affected by the Fukushima Daiichi Nuclear Power Station accident have shown that the transfer factor (TF) of radioactive Cs from soil to brown rice (Oryza sativa L.) varied greatly among fields and locations (Kato Citation2012; Saito et al. Citation2012). Soil potassium (K) availability is known to be a primary factor affecting the variation in Cs uptake in rice and other crops (Tsumura et al. Citation1984; Zhu and Smolders Citation2000; Tsukada et al. Citation2002b; Kato Citation2012; Saito et al. Citation2012). Our previous study, which examined four cesium-137 (137Cs)-contaminated paddy soils in Fukushima prefecture, indicated that Cs accumulation in brown rice was closely related to the exchangeable Cs/K ratio, for both 137Cs and cesium-133 (133Cs) in the soil (Kondo et al. Citation2014). This suggests that Cs and K in soil interactively determine the transfer of Cs from soil to plant. Although Cs and K have basically similar behaviors in the soil–plant system because of their similar atomic properties, it is known that the Cs/K ratio in rice plants varies by plant parts and growing conditions in rice, which indicates the different behaviors of Cs and K (Tsumura et al. Citation1984; Tsukuda et al. Citation2002a). The variability in (Cs/K in plant)/(Cs/K in substrate), noted as the discrimination factor (DF), was widely recognized among crop species and plant parts (Smolders and Shaw Citation1995; Zhu and Smolders Citation2000). However, the variability in Cs and K accumulation in different parts of rice plants is not well understood when the rice is grown under diverse soil conditions with different Cs and K availabilities. Understanding the mechanisms for Cs allocation into each part of the plant, particularly in brown rice, is critical to control Cs accumulation in rice grain in contaminated soils.
The aims of this study are to validate the exchangeable Cs/K ratio as a soil index to estimate Cs uptake and accumulation in plants using soils with a wide range of exchangeable K and Cs, and also to examine the variation in Cs/K in plant parts, including roots, to elucidate comparative dynamics of Cs and K in the soil–plant system. The variability in Cs distributions in grains and shoots was also examined in comparison with K and other elements. Based on the observed relationship between Cs and K in soil and plant parts, possible effective measures to control Cs accumulation by K management were discussed. In this study, the behavior of 133Cs was investigated in soils which were not recently contaminated with 137Cs, with the assumption that the behavior of 133Cs can be a surrogate for that of 137Cs, because no large isotope effect has been reported in uptake and distribution in rice plants.
MATERIALS AND METHODS
Soil properties
Pot experiments were conducted using nine paddy soils (S1–S9) with varying K levels, which originated from Ibaraki, Saitama and Tochigi Prefectures, Japan (). Exchangeable Cs also varied among soils. The fraction of exchangeable forms in total concentrations of Cs varied from 0.93% in S4 to 7.58% in S8.
Table 1 Soil properties
Plant culture
Rice (O. sativa L. cv. Hitomebore) plants were grown in plastic Wagner pots (1/5000a) containing soil (2.4 kg pot−1) in naturally lit chambers with daily average temperatures of approximately 23°C. Three 14-day-old seedlings were transplanted into each pot and grown under submerged conditions until maturity. Nitrogen (N; controlled-release urea) and phosphorus (P; single superphosphate) were applied as basal applications at 0.9 g N pot−1 and 0.11 g P pot−1, respectively.
Plant analysis
Aboveground plant parts and roots were sampled at maturity 132 d after transplantation and were separated into different parts. The aboveground part was separated into active leaf, dead leaf, stem (culm and leaf sheath), rachis, husk and brown rice. The dead leaf was distinguished from the active leaf when more than half of the leaf area was in senescence. The root was carefully washed until soil particles were no longer visible on the root surface. Plant samples were dried at 70°C and dry weight was determined. Samples were then finely ground using a milling machine (TI-100, Fujiwara Scientific Co., Japan). The ground sample was used for determination of Cs using Inductively coupled plasma - mass spectrometry (ICPMS) (ELAN DRC Plus, Perkin Elmer Co. USA), and other essential elements (K, Ca, Mg, Mn, S, Al, Zn, and P) by ICP-AES (Optima 3000, Perkin Elmer Co. USA) after digestion with nitric acid (HNO3). N concentration in plants was determined using Isotope-ratio mass spectrometry (IRMS) (Delta XP, ThermoFinnigan, Co. Germany). All measurements had four replications.
Clay mineralogy
Clay mineralogy of the soils was investigated following the methods adapted in a previous report (Kondo et al. Citation2014).
Data analysis
All data were subjected to analysis of variance (ANOVA), and the nine soils were compared by Tukey’s multiple range test (P < 0.05) using the statistical software Statistix (v. 9, Analytical Software Co., USA).
RESULTS
Clay mineralogy
Clay mineralogy is presented in . S1, with the lowest exchangeable Cs concentration, and S4, with the lowest fraction of exchangeable Cs/total Cs, both originated from Konosu and appeared to contain mica and vermiculite to a higher extent than did the other soils.
Table 2 Comparison of clay mineralogy of soils
Effect of exchangeable Cs and K on Cs uptake
Plant growth at maturity is shown in . Dry weights in aboveground parts and roots were similar among soils, except S8 in which the plant showed P deficiency because of low soil-available P.
Table 3 Dry weight and panicle number at maturity
Aboveground Cs uptake decreased with increasing exchangeable K (). S4, with high mica and vermiculite, exhibited low Cs uptake relative to exchangeable K levels, according to the regression line. Aboveground Cs uptake tended to increase with increasing exchangeable Cs in the soil, but a significant correlation was not obtained (). Cs uptake in aboveground parts showed a linear correlation with the exchangeable Cs/K ratio, including in S4 ().
Relationship of Cs in plants with soil exchangeable Cs and K
As in aboveground Cs uptake, Cs concentration in brown rice was linearly correlated with the exchangeable Cs/K ratio (). The linear relationship in this experiment was fairly similar to those obtained in a previous study using four soils from Fukushima Prefecture (Kondo et al. Citation2014).
Figure 3 Relationship between cesium-133 (133Cs) concentration in brown rice and exchangeable cesium-133/potassium (133Cs/K) ratio among soils (** indicates P < 0.01).
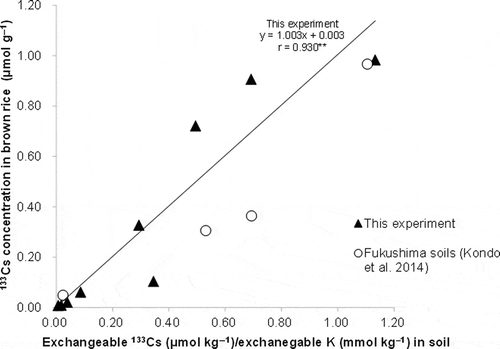
Because the ratio of Cs/K concentration in roots and all aboveground parts and the exchangeable Cs/K ratio showed significant linear correlation, the slope in the equation between them was calculated as follows and is presented in :
where Y is the Cs/K concentration ratio in plant parts, a is a slope and X is the exchangeable Cs/K ratio in the soil.
The slope was considerably higher in roots than in aboveground parts. Among aboveground parts, the slopes in brown rice and dead leaf were larger than those for active leaf, stem, rachis and husk, indicating Cs enrichment relative to K in these organs.
Cs concentration in brown rice was closely related to that in the shoot (stem and leaves; ). In contrast, the K concentration in brown rice was almost constant regardless of that in the shoots, except in S8 with the P-deficient soil (), which indicates the stability of K concentration in brown rice.
Cs distribution in plants
The distributions of Cs and other elements are compared in brown rice and in aboveground parts, presented on average for the nine tested soils (). There were considerable differences among elements in their distribution in brown rice relative to that for dry matter. P and N showed higher distribution than dry matter. Mn, Ca, Al, and iron (Fe) showed a distribution rate lower than 10% in all soils. Cs distribution in brown rice was 14.5% on average, which was less than dry matter and in a similar range to K. There was considerable variation in Cs distribution in brown rice among soils. Cs distribution in brown rice was negatively related to K concentration in the stem (). Cs distribution in aboveground parts, calculated as:
was also negatively related to K concentration in the root ().
Figure 5 Distribution into brown rice in aboveground parts in terms of dry matter (DW) and essential elements in comparison with cesium-133 (133Cs) on the average of nine soils (bars indicate maximum and minimum values).
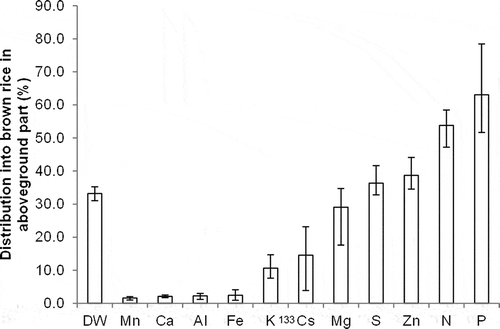
DISCUSSION
Cs uptake and soil exchangeable Cs and K
Cs uptake decreased with higher exchangeable K (), which is also well documented in past reports (Tsumura et al. Citation1984; Kato Citation2012; Saito et al. Citation2012). The results of this study agreed with field observations that the TF for brown rice tended to increase with low levels of exchangeable K, particularly in the range below 5.3 mmol K kg−1 (Kato Citation2012). Exchangeable Cs tended to show a positive effect on Cs uptake, although the correlation coefficient was smaller than that for K (). Aboveground Cs uptake was linearly related to the exchangeable Cs/K ratio, including in S4, which had a lower Cs uptake relative to exchangeable K levels (). This relationship possibly reflects the competitive uptake of Cs with K and the importance of the exchangeable Cs levels in addition to exchangeable K in soil.
There were considerable differences in exchangeable Cs and the fraction of exchangeable Cs in total Cs among soils. It is known that the abundance of frayed-edge sites (FES) is one of the critical factors that determine Cs exchangeability (Sawhney Citation1964; Tsumura et al. Citation1984; Cremers et al. Citation1988; Nakao et al. Citation2008). Cs fixation is generally strong in specific clay types with high FES frequency, such as mica and vermiculite. The soils originating from Konosu, S1 and S4, showed relatively lower exchangeable forms and fraction of exchangeable forms, respectively, which were probably due to the higher content of mica and vermiculite compared with other soils. There were also large variations in exchangeable Cs in other soils, which could not be fully explained in this study. Further investigation of soil characteristics and amendments affecting Cs exchangeability would be useful in evaluating the soil Cs-supplying capacity.
Validation of exchangeable Cs/K ratio as an index for Cs concentration in brown rice
As with Cs uptake, a linear correlation was observed between Cs concentrations in brown rice and the exchangeable Cs/K ratio (). This reconfirms the results of the previous study (Kondo et al. Citation2014) using 137Cs and 133Cs in four soils from Fukushima Prefecture, which had a narrower range of exchangeable K than those in this experiment. The linear correlation was fairly similar between the two experiments. The slight differences observed between the two experiments can possibly be attributed to the different K fertilization and growing conditions. The relationship between Cs in brown rice and the Cs/K ratio seemed to be relatively stable over a wide range of K levels when the same variety was used. This suggests the possible use of exchangeable Cs/K as a soil index to estimate Cs in brown rice in diverse soils. It is necessary to further confirm the reproducibility of the results using other rice varieties and growing conditions.
Variation in Cs/K in plant parts in relation to soil exchangeable Cs/K
There were similarities and discrepancies in Cs and K distribution throughout plant parts (Smolders and Shaw Citation1995; Tsukada et al. Citation2002a; Yoshida and Takahashi Citation2012). The ratio of Cs/K concentration in different plant parts reflected the soil exchangeable Cs/K ratio with different slopes (). The slope can be considered an apparent discrimination rate of Cs against K of the transfer from soil to the plant, which is generally denoted as DF (Zhu and Smolders Citation2000). Tensho et al. (Citation1961) reported that DF, calculated as Cs/K in plant/exchangeable Cs/K, in lowland rice was considerably higher than in upland rice and other upland crops. In this study, a significant linear correlation was obtained in all plant parts (), suggesting that DF was almost constant over the wide range of exchangeable Cs/K in soil. This indicates that the Cs/K ratio in the plant was largely dependent on exchangeable Cs/K ratio in soil and variable among plant parts.
Table 4 Slope in the linear regression between cesium-133/potassium (133Cs/K) concentration in plant part and exchangeagble cesium-133/potassium (133Cs/K) in soil
Different slopes among plant parts were obtained in this study, implying that there was variation in the Cs/K ratio among parts, as previously reported in rice (Tensho et al. Citation1961; Tsumura et al. Citation1984; Tsukada et al. Citation2002a). The larger slope in the roots than the aboveground parts indicates a higher Cs/K ratio in the roots than in aboveground parts. Although the possibility of overestimation of Cs/K in roots due to contamination of soil particles could not be totally excluded, it was assumed that soil contamination was minimal because neither the concentration of Al nor that of Fe had a clear relationship with Cs in the roots. A higher Cs/K ratio in the roots than in the aboveground parts signified a discrimination of Cs against K in the transfer from roots to aboveground parts.
Among the aboveground parts, the slope was larger in dead leaves than in active leaves. This difference is in agreement with previous studies (Tsukada et al. Citation2002a) that demonstrated higher Cs in old, lower leaves, with an opposite trend for K. These results signify that Cs in leaves is less mobile than K at leaf senescence, although further careful examination is needed to compare the changes in Cs and K concentration in different leaf positions during emergence to senescence of leaves. Preferential K remobilization at leaf senescence may be one factor explaining the higher Cs/K in the dead leaves. It would be interesting to compare the chemical form and dynamics in the leaves between Cs and K.
As indicated by the difference in the slopes, the Cs/K ratio in brown rice was higher than that in the husk, which showed a similar Cs/K ratio to the stem and active leaves. The transport of Cs into brown rice from the shoots and husks may have a different mode to that of K. The larger Cs/K ratios observed in brown rice than in vegetative tissues are in agreement with other investigations (Tensho et al. Citation1961; Tsumura et al. Citation1984; Tsukada et al. Citation2002a). The Cs concentration in brown rice increased almost proportionally to that in the shoot, whereas the K concentration in brown rice was rather constant regardless of the shoot K concentration ( and ). A stable K concentration in brown rice has been observed across cultivational and soil conditions (Tsukada et al. Citation2002a). It is considered that localizations of K and Cs in brown rice are basically similar and both elements primarily accumulate in the aleurone layer (Ogawa et al. Citation1979; Tsukada et al. Citation2002a). The reason for the difference in the accumulation between Cs and K in brown rice remains for further study, and comparative analysis is desirable, with a focus on the stages and the form of Cs and K accumulated in brown rice, especially in the aleurone layer.
Variation in Cs distribution among plant parts
Among tested elements, Cs and K had moderately high distributions in brown rice (). A greater Cs distribution in brown rice compared with K was observed in 137Cs (Tsukada et al. Citation2002a). In this study, Cs distribution in brown rice was also slightly higher than that of K on average in soils, but with considerable variation (). A negative relationship between Cs distribution in brown rice and stem K concentration was found (), which signified the suppressive effect of high K status in plants on Cs translocation into brown rice. In addition, the negative relationship between Cs distribution in aerial parts and root K concentration () suggests a suppressive effect of high K status on Cs translocation from roots to aerial parts. Decreasing K supply increased root:shoot Cs concentration in wheat (Triticum aestivum L.) (Smolders et al. Citation1996).
Control of Cs accumulation based on competitive dynamics between Cs and K
In this study, we investigated 133Cs using soils which were not exposed to serious contamination with 137Cs, assuming that the results obtained in this study with 133Cs can be a basis for the control of 137Cs accumulation in soils affected by 137Cs contamination. There were similarities found between 133Cs and 137Cs in distribution among plant parts, and in the relationship between plant uptake and exchangeable Cs contents (Tsukada et al. Citation2002a; Kondo et al. Citation2014). On the other hand, it was found that there were discrepancies between 133Cs and 137Cs in accumulations by rice varieties under the field conditions in Fukushima Prefecture, which was affected by the accident at the Fukushima Daiichi Nuclear Power Station in 2011 (Ohmori et al. Citation2014). This discrepancy was, as the authors speculated, probably due to the difference in distribution in soil profile and availability to the rice between 133Cs and 137Cs, because 137Cs had been freshly added to the soil surface, and not due to the isotopic effect.
This study, together with the previous study (Kondo et al. Citation2014), showed the significance of soil exchangeable Cs/K and the competitive dynamics between Cs and K in root absorption and translocation in plant to determine Cs accumulation in rice plant. These results suggest a possible direction for efficient K application methods, diagnosis of soil and plants, and other measures to control Cs accumulation in each part of plants. The K application rate can be adjusted with consideration of not only exchangeable K, but also exchangeable Cs levels. It would also be useful to control the Cs/K ratio in shoots by K application to effectively suppress Cs levels in brown rice. The close relationship between Cs and K absorptions suggested that to suppress Cs uptake, maintaining K levels in soil solution is probably important in the vicinity of the root that is actively absorbing Cs, although further trials in the field are needed. For the selection and development of low Cs-accumulating varieties, evaluation of varietal differences in competitiveness between Cs and K in root absorption and accumulation can be utilized.
At present, the precise mechanisms underlying the competitive relationships between Cs and K in root absorption and translocation processes are not fully understood. Our understanding of the physiological mechanisms for uptake and transport of Cs has been advanced in recent years (Zhu and Smolders Citation2000; Yamaguchi et al. Citation2012). It is assumed that root uptake and Cs transport are predominantly mediated by K transporters, K channels and voltage-independent cation channels (VICC), and the affinity and selectivity for Cs and K possibly differ among these systems (White and Broadley Citation2000; Zhu and Smolders Citation2000; Broadley et al. Citation2001; Qi et al. Citation2008). Genetic analysis in Arabidopsis thaliana (L.) indicated that several quantitative trait loci (QTLs), including AtCNGC1, a VICC, are involved in controlling Cs concentration in plants (Kanter et al. Citation2010). Further information on molecular mechanisms for the competitive absorption and translocation of Cs and K would provide a physiological basis to enhance the development of efficient Cs-controlling measures by cultural management and genotypic improvement.
ACKNOWLEDGMENTS
This work was partly funded by “Research and development projects for application in promoting new policy of Agriculture Forestry and Fisheries,” Ministry of Agriculture, Forestry and Fisheries (MAFF), Japan. The authors thank Ms. S. Saka, Ms. M. Ishida and other staff of the Institute of Crop Science (NICS) for technical assistance in plant and soil analysis.
REFERENCES
- Broadley MR, Escobar-Gutierrez AJ, Bowen HC, Willey NJ, White P 2001: Influx and accumulation of Cs+ by the akt1 mutant of Arabidopsis thaliana (L.) Heynh. Lacking a dominant K+ transport system. J. Exp. Bot., 52, 839–844.
- Cremers A, Elsen A, De Preter P, Maes A 1988: Quantitative analysis of radiocaesium retention in soils. Nature, 335, 247–249. doi:10.1038/335247a0
- Kanter U, Hauser A, Michalke B, Dräxl S, Schäffner AR 2010: Caesium and strontium accumulation in shoots of Arabidopsis thaliana: genetic and physiological aspects. J. Exp. Bot., 61, 3995–4009. doi:10.1093/jxb/erq213
- Kato N 2012: Countermeasures to reduce radiocaesium contamination in paddy rice, soybean and cabbage. In Abstract, International science symposium on combating radionuclide contamination in Agro-soil environment. 3–10 March 2012 Koriyama, Fukushima, pp 317–318.
- Kondo M, Maeda H, Goto A et al 2014. Exchangeable Cs/K ratio in soil is an index to estimate accumulation of radioactive and stable Cs in rice plant. Soil Sci. Plant Nutri., doi:10.1080/00380768.2014.973347
- Nakao A, Thiry Y, Funakawa S, Kosaki T 2008: Characterization of the frayed edge site of micaceous minerals in soil clays influenced by different pedogenetic conditions in Japan and northern Thailand. Soil Sci. Plant Nutri., 54, 479–489. doi:10.1111/j.1747-0765.2008.00262.x
- Ogawa M, Tanaka K, Kasai Z 1979: Accumulation of phosphorus, magnesium and potassium in developing rice grains: followed by electron microprobe X-ray analysis focusing on the aleurone layer. Plant Cell Physiol., 20, 19–27.
- Ohmori Y, Inui Y, Kajikawa M et al 2014: Difference in cesium accumulation among rice cultivars grown in the paddy field in Fukushima Prefecture in 2011 and 2012. J. Plant Res., 127, 57–66.
- Qi Z, Hampton CR, Shin R, Barkla BJ, White PJ, Schachtman DP 2008: The high affinity K+ transporter AtHAK5 plays a physiological role in planta at very low K+ concentrations and provides a caesium uptake pathway in Arabidopsis. J. Exp. Bot., 59, 595–607. doi:10.1093/jxb/erm330
- Saito T, Ohkoshi S, Fujimura S, Iwabuchi K, Saito M, Nemoto T, Sato M, Sato M, Yoshioka K, Tsukada H 2012: Effect of potassium application on root uptake of radiocesium in rice. In Proc. International symposium on environmental monitoring and dose estimation of residents after accident of TEPCO’s Fukushima Daiichi Nuclear Power Stations [CD-ROM]. Kyoto University Research Reactor Institute, Osaka.
- Sawhney BL 1964: Sorption and fixation of microquantities of cesium by clay minerals: effect of saturating cations. Proc. Soil Sci. Soc. Am., 28, 183–186. doi:10.2136/sssaj1964.03615995002800020017x
- Smolders E, Shaw G 1995: Changes in radiocaesium uptake and distribution in wheat during plant development: a solution culture study. Plant Soil, 176, 1–6. doi:10.1007/BF00017669
- Smolders E, Kiebooms L, Buysse J, Merckx R 1996: 137Cs uptake in spring wheat (Triticum aestivum L. cv Tonic) at varying K supply I. The effect in solution culture. Plant Soil, 181, 205–209. doi:10.1007/BF00012054
- Tensho K, Yeh K-L, Mitsui S 1961: The uptake of strontium and cesium by plants from soil with special reference to the unusual cesium uptake by lowland rice and its mechanism. Soil Plant Food, 6, 176–183. doi:10.1080/00380768.1961.10430944
- Tsukada H, Hasegawa H, Hisamatsu S, Yamasaki S 2002a: Rice uptake and distributions of radioactive 137Cs, stable 133Cs and K from soil. Environ. Poll., 117, 403–409. doi:10.1016/S0269-7491(01)00199-3
- Tsukada H, Hasegawa H, Hisamatsu S, Yamasaki S 2002b: Transfer of 137Cs and stable Cs from paddy soil to polished rice in Aomori, Japan. J. Env. Radio., 59, 351–363. doi:10.1016/S0265-931X(01)00083-2
- Tsumura A, Komamura M, Kobayashi H 1984: Behavior of radioactive Sr and Cs in soils and soil-plant systems. Bull. Natl. Inst. Agric. Sci. Ser., B, 36, 57–113. In Japanese.
- White PJ, Broadley MR 2000: Tansley Review No. 113. New Phytologist, 147, 241–256. doi:10.1046/j.1469-8137.2000.00704.x
- Yamaguchi N, Takata Y, Hayashi K et al. 2012: Behavior of radiocaesium in soil-plant systems and its controlling factor. A review. Bulletin of National Institute for Agro-Environmental Sciences, 31, 75–129. In Japanese
- Yoshida N, Takahashi Y 2012: Land-surface contamination by radionuclides from the Fukushima Daiichi nuclear power plant accident. Elements, 8, 201–206. doi:10.2113/gselements.8.3.201
- Zhu YG, Smolders E 2000: Plant uptake of radiocaesium: a review of mechanisms, regulation and application. J. Exp. Bot., 51, 1635–1645. doi:10.1093/jexbot/51.351.1635