Abstract
We investigated the effects of various soil amendments on the concentration of arsenic (As) in soil solutions under flooded anaerobic conditions. Ten amendments (six As adsorbents and four general agricultural amendments) were used with two soil samples (Aquepts). The time-course changes in the concentrations of As, iron (Fe) and silicon (Si) in soil solutions with or without amendments were measured after incubations of approximately 30, 60 and 100 d. We conclude that a precipitate of polysilicate-iron solution (1.1 mol L‒1 Fe) shows promise as a novel As adsorbent for flooded anaerobic soils such as paddy soils. The precipitate is present in sludge from water purification plants and is therefore potentially cost effective. Moreover, it could increase the concentration of Si in soil solution, which could decrease As uptake by rice plants. Ferrihydrite and aluminum-substituted ferrihydrite were also effective for decreasing the dissolved As in both soils and nano-sized layered double hydroxides, and magnesium oxides worked in one soil. Poultry manure significantly increased dissolved As in both soils, but composted bark and silicate fertilizers did not change As in either soil. Some amendments had different effects in the two soil samples; further comprehensive studies will need to focus on how soil properties influence the effects of amendments.
1. INTRODUCTION
Arsenic (As) is a non-threshold carcinogen and a cause of some diseases. Rice is the most important staple food in Japan, and the Japanese people obtain about 6.3% of their total As intake from rice [Ministry of Agriculture, Forestry and Fisheries of Japan (MAFF) Citation2014]. The Codex Alimentarius Commission decided in 2014 what the permitted concentration of inorganic As would be in rice grains (0.2 mg kg‒1 as polished rice grains). Therefore, reducing the concentration of As in rice is important to decreasing the health risk posed by As. In addition, about half of the total intake in Japan of cadmium (Cd), a hazardous heavy metal, comes from the consumption of rice (MAFF Citation2006). This indicates the necessity of reducing Cd, as well as As, in rice grains.
Under anaerobic conditions, Cd precipitates as a barely soluble sulfide (Iimura Citation1993), resulting in a low Cd availability for rice plants. For example, field experiments by Inahara et al. (Citation2007) showed that flooded cultivation during the entire rice growing period, or the latter part of it, significantly decreased Cd in rice grains by more than 80%. MAFF therefore recommended flooded cultivation of rice for 3 weeks before and after ear emergence to reduce Cd in rice grains. Flooded cultivation, however, increases As solubility through the reduction of As(V) to As(III) and the reductive dissolution of As-bearing iron (Fe)-oxides in soils (Masscheleyn Citation1991). As expected, flooded cultivation increased As in rice grains, whereas aerobic cultivation increased Cd (Arao et al. Citation2009).
One possible strategy for the simultaneous decrease of As and Cd is a combination of flooded cultivation to decrease Cd and the application of soil amendments to decrease As. There have been many laboratory- or field-scale tests of adsorbents to remove As from water systems (e.g., wastewater and groundwater) and to stabilize As in polluted soils (Mench et al. Citation2003; Yang et al. Citation2005; De La Fuente et al. Citation2010). Examples of tested adsorbents are clays, aluminum (Al) oxides, layered double hydroxides (LDHs), manganese (Mn) oxides and Fe-type adsorbents (e.g., Fe oxides, zero-valent iron and Fe sulfide; Wang and Mulligan Citation2006a; Mohan and Pittman Citation2007; Komárek et al. Citation2013). On the other hand, few reports have focused on As stabilization in anaerobic and slightly or moderately polluted soils, which pose a potential risk of producing rice that exceeds the permitted levels for As, especially where flooded cultivation is implemented to restrict Cd uptake.
In many cases, Fe-type adsorbents were considered promising as amendments. Ferrihydrite has a large specific surface area (200‒500 m2 g‒1; Schwertmann and Taylor Citation1989), and efficiently adsorbs both arsenite and arsenate (Raven et al. Citation1998). The Fe in ferrihydrite can be replaced by Al, changing some characteristics of the compound. Ekstrom et al. (Citation2010) showed that an Al-substituted ferrihydrite was more resistant to dissimilatory microbial reduction. This indicates that Al substitution might extend the effective period of ferrihydrite as an As adsorbent. Polysilicate-iron (PSI) solutions are used as flocculants in some water-purification plants in Japan. The resulting sludge contains large amounts of Fe and polysilicate with suspended-solid-like clay minerals, and therefore might act as an As adsorbent.
Layered double hydroxides, also known as hydrotalcite-like minerals, have the general structure of [M2+1‒x M3+x (OH)2]x+ [(An‒)x/n·yH2O] (e.g., Mg6Al2(OH)16CO3 · 4H2O), where M2+, M3+ and An‒ represent divalent cations, trivalent cations and n-valent anions, respectively. They have a stacked structure with alternating “host” layers [M2+1‒x M3+x (OH)2]x+ and “guest” layers [(An‒)x/n·yH2O]. A portion of the divalent cations in the host layer are substituted with trivalent cations, resulting in a permanent positive charge; thus, LDH can adsorb anions, including AsO33‒ and AsO43‒, in their interlayer through an anion exchange reaction (Goh et al. Citation2008).
As a soil amendment, magnesium oxide (MgO) reacts with water and soil components, forming materials such as magnesium hydroxide [Mg(OH)2]. These materials could decrease As solubility in soils (Wada and Morishita Citation2013). Moreover, the increase in pH by the addition of MgO might inhibit the reductive dissolution of As-bearing Fe oxides and subsequent As release.
Organic amendments are routinely applied to agricultural fields to modify soil properties and to supply nutrients. They can, however, increase As solubility, for example by promoting the reductive dissolution of As-bearing Fe oxides (Wang and Mulligan Citation2006b). For example, Norton et al. (Citation2013) reported that the addition of farmyard manure increased As in rice grains. It is well known that silicon Si is not essential for rice growth but is useful because it strengthens plant tissues. However, because silicate ions adsorb onto Fe oxide and block As adsorption (Waltham and Eick Citation2002), silicate application increases dissolved As in soils (Seyfferth and Fendorf Citation2012). Nevertheless, As in rice plant tissues decreased with increase in silicic acid in soil solution (Bogdan and Schenk Citation2008).
Against such a background, the main objectives of this study were (1) to screen prospective As adsorbents under flooded anaerobic conditions, and (2) to clarify the effects of some general agricultural amendments on dissolved As under these conditions. We additionally discuss the effects of these adsorbents and amendments on Si concentrations in soil solution.
2. MATERIALS AND METHODS
2.1. Soil samples
Soil samples were collected from the plowed layer of a paddy field in Chugoku district (soil A) and in Tohoku district (soil B), Japan. The soil samples were screened through a 2-mm-mesh sieve. Both soils were classified as Aquepts in the United States Department of Agriculture (USDA) soil taxonomy system (Soil Survey Staff Citation1998). The sieved samples were stored at 4°C until use.
Soil pH was measured by a pH meter with a glass electrode in a suspension of 1 g soil in 2.5 mL water. The total carbon (C) and nitrogen (N) contents in the samples were determined by a dry combustion method (Sumigraph NC-22 F, Shimadzu, Kyoto, Japan). The clay content was measured using a pipette method (Gee and Bauder Citation1986). Dithionite-citrate-, oxalate- and pyrophosphate-extractable elements (Al, Fe, Mn and Si) were extracted as described by Blakemore et al. (Citation1987). Hydrochloric acid (HCl)-soluble As was extracted in 1 mol L‒1 HCl at a soil-to-solution ratio of 1:10 (5 g soil in 50 mL HCl) with mechanical shaking at 30°C for 1 h. The concentrations of the elements in these extracts and digests were measured by inductively coupled plasma–optical emission spectrometry (ICP–OES; Vista-Pro, Varian, Palo Alto, CA, USA).
2.2. Preparation and properties of amendments
Six adsorbents and four general agricultural amendments were used (). Four of the adsorbents were synthesized in our laboratory; the other products were commercially obtained.
Table 1 General information about the amendments used
Ferrihydrite (Fh) and Al-substituted Fh (Al-Fh) were synthesized as described by Ekstrom et al. (Citation2010). Briefly, a ferric chloride solution or a mixed solution of ferric chloride and aluminum nitrate was rapidly titrated with sodium hydroxide, followed by repeated centrifugations and resuspensions to remove impurities. The precipitates were dried at 30°C for some days and then ground in an agate mortar and passed through a 75-μm-mesh sieve. Polysilicate-iron precipitates (PSIp) were synthesized by slow titration of two kinds of commercial PSI solutions (Suido Kiko Kaisha, Ltd, Tokyo, Japan) using 1 mol L–1 potassium hydroxide (KOH). The precipitates were centrifuged several times for purification. After drying at 30°C for some days, the precipitates were ground in an agate mortar and passed through a 212-μm-mesh sieve. The precipitates from low-Fe (Fe = 0.37 mol L‒1) and high-Fe (Fe = 1.1 mol L‒1) solutions are referred to as PSIp-L and PSIp-H, respectively.
The LDH and MgO amendments were commercially obtained. These are used for removing toxic heavy metals and anions from wastewater or for stabilizing pollutants in soils. The LDH was characterized as a nano-scale crystal size (Ono et al. Citation2011), and is therefore referred to as nano-LDH. The anion species in the guest layer of nano-LDH was chloride. Because these were powdered products, they were used without grinding and sieving.
Two commercial organic amendments were used, namely composted bark (CB) and poultry manure (PM). These were not dried, but were sieved through a 2-mm-mesh sieve and then stored at 4°C until use. Silicate fertilizers were also commercially obtained. The one designated as SiF-1 had silicate potassium as a major component, and that designated as SiF-2 was acid-soluble silicate with an alkaline component. Both of these were ground and passed through a 0.5-mm-mesh sieve.
The total C and N contents of CB and PM (dried at 65°C and ground) were measured by a dry combustion method (Sumigraph NC-22F, Shimadzu). Total concentrations of Fe, Al, Si, Mg and K in Fh, Al-Fh, nano-LDH, MgO, CB and PM, and As in all amendments were determined by digestion in a concentrated nitric acid (HNO3), HNO3–hydrogen fluoride (HF), or HNO3–perchloric acid (HClO4)–HF solution, and measurement by ICP–OES and inductively coupled plasma–mass spectrometry (ICP–MS; Elan DRC-e or Nexion, PerkinElmer, Waltham, MA, USA). The content of these elements (except for As) in PSIp-L, PSIp-H, SiF-1 and SiF-2 was measured with an X-ray fluorescence spectrometer (JSX-3220, JEOL, Tokyo, Japan). The specific surface areas of Fh, Al-Fh, PSIp-L and PSIp-H were determined by 5-point BET measurements with N2 gas adsorption (Autosorb-1, Quantachrome Corporation, Boynton Beach, FL, USA).
2.3. Soil incubation
For each amendment, a moist soil sample (10 g, oven-dried basis) and a measured amount of the amendment () were mixed with ultra-pure water to bring the total water volume up to 30 mL in a 50-mL glass vial. After N2 gas purging for 2 min, the vial was covered with a butyl rubber cap, tightly sealed with an Al cap, and then manually shaken well. The mixture was incubated at 30°C in an incubator, with manual shaking every second or third day. These steps were performed in triplicate. Two sets of controls were prepared (control A and B) for each soil because the samples were incubated in two batches. The incubation periods were about 30, 60 and 100 d. After the incubations, supernatant was collected using a syringe and needle (NN-2360C, Terumo Corporation, Tokyo, Japan) with a 0.2-µm filter (DG2 M-330, Spectrum Laboratories, Inc., Rancho Dominguez, CA, USA) that was purged with N2 gas before use. Approximately 9 mL of filtrate was immediately mixed with 1 mL of 1.7 mol L‒1 HNO3 to avoid the precipitation of Fe hydroxides, and then stored at 4°C until analysis. Eh and pH of the residual suspension were measured using a glass electrode and a platinum electrode, respectively, under an N2 atmosphere.
2.4. Solution analysis
After dilution with 0.16 mol L‒1 HNO3, the concentrations of As, Fe and Si in the filtrates were analyzed by quadruple ICP–MS and ICP–OES.
2.5. Statistical analysis
A t-test was used to compare the differences between the concentrations of As in controls and those in each treatment. Tukey’s test was used to evaluate the differences in decreasing rate of dissolved As among treatments of As adsorbents, using R software (ver. 3.1.1).
3. RESULTS AND DISCUSSION
3.1. Properties of soil samples and amendments
Both soil A and soil B were acidic; the pH of soil A was higher than that of soil B by about 0.5 pH units (). Soil B contained much more total C and N, oxalate-extractable Al and Fe and dithionite-citrate-extractable Fe, whereas both soils had comparable oxalate-extractable Mn and Si, and clay. The amount of HCl-extractable As was the same in both soils, at about 8 mg kg‒1, indicating that the paddy fields were slightly or moderately contaminated with As.
Table 2 Selected properties of soils and amendments
The total C contents of CB and PM were comparable, whereas the total N content of PM was twice as much as that of CB (), resulting in a higher C:N ratio in CB (21.5) than in PM (8.06). The major component of Fh, Al-Fh, PSIp-L and PSIp-H was Fe. Al-Fh contained 30.7 g kg‒1 Al, and the Al:Fe mole ratio was about 0.11. PSIp-L and PSIp-H contained Si at 160 and 57 g kg‒1 respectively, giving Fe:Si mole ratios of 1.3 and 5.2, respectively. The main components of nano-LDH and MgO were Al and Mg, and Mg, respectively. Most amendments contained trace levels (< 1 mg kg‒1) of As, but some amendments contained relatively higher amounts of As (< 12.0 mg kg‒1). The main components of CB and PM were C and N, with minor amounts of metals. The surface areas increased in the order of Fh (295 m2 g‒1) < Al-Fh (336 m2 g‒1) < PSIp-H (361 m2 g‒1) < PSIp-L (416 m2 g‒1).
3.2. pH and Eh
The average pH of control samples was 7.19 after incubation for 30 d, 7.20 after 60 d and 7.11 after 100 d in soil A, and 6.71, 6.81 and 6.78 in soil B. The pH was therefore considered nearly constant, at least from day 30 to day 100. The average Eh of soil A was also nearly constant (‒229, ‒231 and ‒231 mV), whereas that of soil B decreased notably over time (‒149, ‒179 and ‒207 mV; ). In soil A, there were slight differences among treatments in the pH and Eh, which ranged from 6.98 to 7.37 in pH and from ‒210 to ‒254 mV in Eh. In soil B, in contrast, the values ranged from 6.57 to 7.99 in pH and from ‒127 to ‒291 mV in Eh. The Eh decreased with increasing incubation time. The addition of MgO increased pH and decreased Eh; in soil B, in particular, pH showed much higher values, of 7.82 (30 d), 7.99 (60 d) and 7.91 (100 d) and Eh showed lower values of ‒269, ‒275 and ‒291 mV (circled data points in ) than those of controls. In soil B only, the addition of PM clearly promoted a decrease of Eh down to near the minimum obtained in this study (arrow in ). Other amendments did not notably affect pH and Eh in both soils.
3.3. Effects of amendments on Fe and As concentrations in soil solutions
The average concentrations of dissolved Fe were 84.6 (30 d), 92.5 (60 d) and 97.1 mg L‒1 (100 d) in control samples of soil A, and 85.0, 148 and 187 mg L‒1, respectively, in those of soil B (). These results indicate that Fe oxides were reduced rapidly (within 30 d) in soil A, but more slowly (beyond 30 d) in soil B. The dissolved Fe was significantly decreased by the addition of MgO (by 30–50% in soil A and by 90–95% in soil B) because of the increase in pH. In contrast, the addition of PM significantly increased dissolved Fe, except for 100 d in soil B. The increase was up to 260% in case of 30 d in soil B.
Figure 2 The concentration of iron (Fe, top) and silicon (Si, bottom) in solutions of soil A and soil B after incubation for 30 (left), 60 (center) and 100 (right) d. Solid lines and dashed lines indicate samples that were incubated with controls A and B, respectively. Cont., control; Fh, ferrihydrite; Fh-Al, aluminum-substituted ferrihydrite; PSIp-L, “low iron” polysilicate-iron precipitate (Fe:Si = 1.3); PSIp-H, “high iron” polysilicate-iron precipitate (Fe:Si = 5.2); nano-LDH, nano-sized layered double hydroxide; MgO, magnesium oxide; CB, composted bark; PM, poultry manure; SiF-1, silicate fertilizer 1; SiF-2, silicate fertilizer 2.
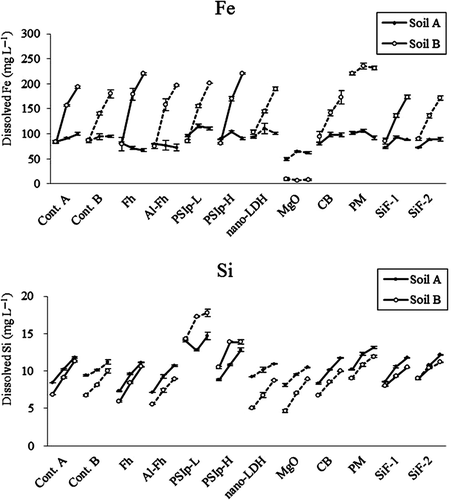
In some preliminary analyses, we found that most of the dissolved As in soil solution was present as arsenite (> 95%, data not shown). Hereafter, therefore, descriptions of dissolved As in our results refer to arsenite.
The relative change over time in dissolved As in control samples of soil B was much larger than that of soil A (); average values in control samples were 659 (30 d), 827 (60 d) and 935 μg L‒1 (100 d) in soil A, and 120, 289 and 442 μg L‒1 in soil B. In soil A, the dissolved As in samples ranged from 272 to 814 (30 d), 522 to 1110 (60 d) and 680 to 1170 μg L‒1 (100 d), whereas those in soil B ranged from 34 to 478, 118 to 418 and 212 to 442 μg L‒1, respectively. Thus, the dissolved As levels in soil B were much lower and more variable than those in soil A, especially during the earlier part of the incubation period. Except for the samples in which As adsorbents were added, the dissolved As closely correlated with dissolved Fe in both soils (R2 = 0.527, P < 0.01 in soil A, R2 = 0.862, P < 0.01 in soil B; n = 18), as reported in past studies (Weber et al. Citation2010; Yamaguchi et al. Citation2011). The positive relationships strongly indicate that dissolved As derived mainly from the dissolution of As-bearing Fe oxides.
Figure 3 The concentration of dissolved arsenic (As) in solutions of (a) soil A and (b) soil B. White bars, mean values after incubation for 30 d; light gray, 60 d; dark gray, 100 d. Stippled bars indicate samples that were incubated with control B. Error bars represent standard deviations (n = 3). Asterisks indicate significant differences from controls at *P < 0.05 and **P < 0.01. Cont., control; Fh, ferrihydrite; Fh-Al, aluminum-substituted ferrihydrite; PSIp-L, “low iron” polysilicate-iron precipitate (Fe:Si = 1.3); PSIp-H, “high iron” polysilicate-iron precipitate (Fe:Si = 5.2); nano-LDH, nano-sized layered double hydroxide; MgO, magnesium oxide; CB, composted bark; PM, poultry manure; SiF-1, silicate fertilizer 1; SiF-2, silicate fertilizer 2.
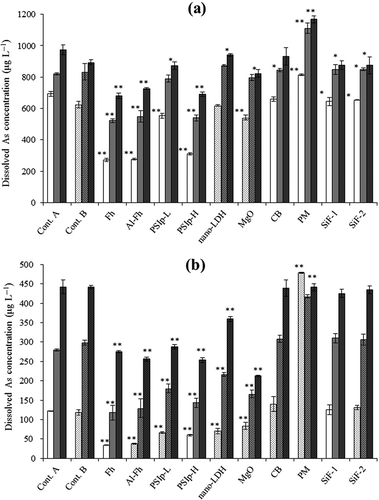
shows the concentration of As in soil solutions after incubation. The addition of Fh and Al-Fh considerably inhibited As solubilization in both soils, although the effects gradually attenuated over time. These amendments decreased dissolved As by about 60% after 30 d, 35% after 60 d and 30% after 100 d in soil A, and by 70, 55 and 40%, respectively, in soil B, relative to the controls (). Because ferrihydrite adsorbs As on both inner-sphere and outer-sphere surface complexes (Arai Citation2010), the decrease in dissolved As was likely caused by this adsorption. Another possible explanation is that the reductive dissolution of these amendments in place of indigenous As-bearing Fe oxides decreased As release from the oxides. The adsorption of As on the hydroxyl sites of Fe oxides is inhibited by coexisting ions such as carbonate species (especially arsenite; Brechbühl et al. Citation2012) and phosphate species (Jain and Loeppert Citation2000). We therefore consider the attenuation of the effects of Fh and Al-Fh (and PSIp, as described below) to be partially caused by the increase of these competing ions, in addition to the decrease in adsorption sites by the reductive dissolution of Fh and Al-Fh. Overall, the effect of Al-Fh addition was slightly less than that of Fh, consistent with the results of Masue et al. (Citation2007), which revealed that the ability of ferrihydrite to remove As from solution decreased with the increase of Al-substitution. Ekstrom et al. (Citation2010) reported that Al-substitution increased the resistance of ferrihydrite to microbial reduction by Shewanella putrefaciens. This suggests that Al-substitution potentially increases the effective period for ferrihydrite as an As adsorbent. In our study, however, the time-course changes in As and Fe showed no clear superiority of Al-Fh over Fh from the standpoint of sustainability.
Table 3 Effects of soil amendments on dissolved arsenic (As) concentrations in soils
Dissolved As decreased with PSIp-H addition by 55.1% (after 30 d), 34.2% (60 d) and 29.1% (100 d) in soil A, and by 51.0, 48.8 and 42.7%, respectively, in soil B. These decreases are comparable to those resulting from addition of Fh and Al-Fh. The effect of PSIp-L was lower than that of PSIp-H in both soils. The As adsorption mechanisms of PSIp are unknown but are likely similar to those of ferrihydrite. The efficiency of PSIp-H for As removal was greater than that of PSIp-L, which has a larger specific surface area; therefore, Fe content might be a more important determinant for As adsorption ability than the total surface area of the PSIp.
We used a pure precipitate of PSI, but the use of PSI-containing sludge from water purification plants is more desirable and realistic from the standpoint of mitigating cost. Judging from previous studies (Horikita et al. Citation2007; Sato et al. Citation2008) and our unpublished data, the sludge is expected to contain more than 300 g kg‒1 of Fe, although the Fe:Si ratio of used PSI solution and the amounts of impurities (e.g. suspended solid in river) should affect the contents. Because settled sludge traditionally has been largely discarded as industrial waste, it could be a low-cost As adsorbent if a supply network could be established. Moreover, PSI-containing sludge significantly increases rice yield (Sato et al. Citation2008), so its application would increase the farmers’ income as well as decreasing the cost of reducing As risks. For this reason, therefore, a field examination is currently under way using PSI-containing sludge in a MAFF-funded research project.
In soil A, nano-LDH had no clear effects on dissolved amounts of As. Conversely, in soil B, it significantly reduced dissolved As, although the effect gradually attenuated with time. In general, As removal by LDH can be explained by two mechanisms: anion exchange and inner-sphere surface complexation (Morimoto et al. Citation2012). You et al. (Citation2001) showed that other oxyanions substantially inhibited arsenite adsorption onto chloride-intercalated LDH, and further that they promoted arsenite desorption. Therefore, the observed attenuation of efficiency is possibly explained by the increases in competing ions such as carbonate species derived from microbial respiration. As mentioned in section 3.1. soil A was much more easily reducible and less acidic than soil B. This difference suggests that the concentration of carbonate anions in the soil solution of soil A could be higher than that in soil B, and thus nano-LDH did not work as well in soil A.
The addition of MgO significantly reduced dissolved As in soil B, although the effect was not as large in soil A (< 14% decrease). The increase in pH should limit the dissolution of Fe oxides and subsequent As release. Unlike arsenate, arsenite adsorption onto Fe oxides increases with an increase in solution pH up to around 9 (Raven et al. Citation1998; Dixit and Hering Citation2003). Therefore, the increase in pH seems to be a main cause for the decrease of dissolved As in soil B. Another possible cause is As adsorption on high-affinity surface hydroxyl groups at the surface of Mg(OH)2 which is formed by hydration of MgO (Liu et al. Citation2011). However, its solubility indicates Mg(OH)2 could not be present in the mixture under these conditions. Moreover, it is unlikely that the formation of “insoluble” Mg-As minerals reduced As concentrations to < 1 mg L‒1, considering their solubilities (Wada and Morishita Citation2013). Because the application of MgO increases soil pH, resulting in a decrease of Cd uptake by rice plants (Kikuchi et al. Citation2008), it would achieve simultaneous decreases of As and Cd in rice plants. However, the pH value (around pH 8) in soil B is possibly too high for rice plants. In practice, the application amount of MgO should be determined carefully from the standpoints of both plant growth and reducing As uptake; probably that for soil A was larger and that for soil B was smaller than the amendment:soil ratio in this study.
The addition of CB did not substantially change dissolved As and Fe during the incubation period. On the other hand, the addition of PM increased dissolved As by 17.4% (after 30 d), 35.4% (60 d) and 19.9% (100 d) in soil A, and by 293, 49.5 and ‒0.265%, respectively, in soil B, similar to the results for Fe. The addition of PM increased dissolved Fe by 165% (after 30 d), 50.3% (60 d) and 19.9% (100 d) in soil B. The difference between CB and PM might be explained by the difference in their C:N ratios: the higher C:N ratio of CB (21.5) than of PM (8.06) did not promote reductive dissolution of Fe oxides and subsequent As release. Dissolved organic matter might have increased the dissolved As through competitive adsorption, by changing the surface hydroxyl charges on minerals, by forming soluble complexes with As mediated by metal bridging, or by reducing As(V) to As(III) (Wang and Mulligan Citation2006b). Moreover, phosphate competes with As on the adsorption site such as surface hydroxyl on Fe oxides (Jain and Loeppert Citation2000); therefore, phosphate from organic amendments potentially increases As solubility. However, the degree of contribution of these mechanisms to our results could not be evaluated.
Our results indicated that composted bark would not affect dissolved As, at least not from a single application, whereas poultry manures may increase the environmental and health risks of As, although the effect would be attenuated within the earlier stages of rice growth. Moreover, the amount of PM added in our incubation experiments—100 mg per 10 g soil, roughly equal to 10 t ha‒1—is several times greater than the usual applications. Although some reports indicate that the application of organic amendments increases As uptake by rice plants (Xie and Huang Citation1998; Norton et al. Citation2013), other studies show the opposite result. For example, Rahaman et al. (Citation2011) reported some organic amendments that decreased the uptake of As by rice plants, and Hosoda (Citation1942) showed that one type of compost markedly reduced As damage to rice plants. The inconsistency in these results might be caused by differences in the amount of amendment applied, water management, or the properties of the soils and amendments. More systematic and comprehensive studies are needed, and appropriate techniques for the application of organic amendments should be established from the standpoint of minimizing health risks of As.
The additions of the silicate fertilizers SiF-1 and SiF-2 did not substantially change the dissolved As. These amendments are possibly more important as Si supplements rather than as As adsorbents, as described in section 3.4.
3.4. Effects of amendments on Si concentration in soil solutions
Dissolved Si concentrations might affect environmental and health risks through changing As mobility and availability to plants. Dissolved Si decreases the rate and amount of As adsorption onto Fe oxides through Si adsorption and subsequent polymerization (Swedlund and Webster Citation1999; Waltham and Eick Citation2002; Christl et al. Citation2012); therefore, amendment with silicates possibly increases As in soil solutions. On the other hand, an increase in dissolved Si results in a decrease in As in rice plant tissues (Bogdan and Schenk Citation2008). Moreover, recent studies have shown that silicate amendment reduces inorganic As but increases the less-toxic organic As in rice tissues (Liu et al. Citation2014). Therefore, it is useful to understand the effects of the amendments that we tested on the concentration of dissolved Si.
Dissolved Si generally increased over the incubation period in each soil and each treatment (, bottom). The average dissolved Si in the control samples was 8.9 (after 30 d), 10.2 (60 d) and 11.5 mg L‒1 (100 d) in soil A, and 6.8, 8.6, and 10.7 mg L‒1, respectively, in soil B. As the soil pH values were nearly constant throughout the incubation period, the increases in Si appear to be caused by the dissolution of indigenous Si-bearing Fe oxides. For most of the amended soil samples over most of the incubation periods, the dissolved Si was comparable to or slightly lower than that in control samples, maybe because of Si adsorption onto the amendments or decreases in reductive dissolution of indigenous Si-bearing Fe oxides.
In contrast, the addition of PSIp-L and PSIp-H substantially increased the dissolved Si, by 24.8–112% and 4.5–53.0%, respectively, and the increase tended to be higher in soil B than in soil A (). Therefore, the uptake of As by rice plants could be reduced not only by a decrease in dissolved As, but also the increase in dissolved Si. As expected, the addition of silicate fertilizers increased dissolved Si overall, but the effects were less clear than those of PSIp-L, especially in soil A. As described above, the concentration of dissolved As did not significantly increase with the silicate fertilizers. Therefore, at least the application amount of 10 mg per 10 g soil (roughly equal to 1 t ha‒1) did not increase As solubility through the competition for adsorption sites with Si. The addition of PM also increased Si in both soils. Because the Fe also increased simultaneously, the increase in the dissolved Si could have resulted from the accelerated dissolution of indigenous Si-bearing Fe oxides. The increase in dissolved Si might be one of the causes of the increase in dissolved As; on the other hand, it would partially offset the effect of an increase in dissolved As on As uptake by rice plants.
4. CONCLUSIONS
The precipitate of a polysilicate-iron solution (high Fe) could be a novel promising As adsorbent for soil under flooded anaerobic conditions. In addition, it could serve as a supply of Si, which possibly reduces As uptake by rice. Ferrihydrite and Al-substituted ferrihydrite were also effective for reducing the dissolved As in both soils tested, and nano-sized layered double hydroxide and magnesium oxides worked in one soil. Poultry manure significantly increased dissolved As in both soils, but composted bark and silicate fertilizers did not change it in either soil. More studies should be carried out, focusing on the applicability of existing or novel amendments to slightly or moderately As-contaminated flooded soils, because of the importance to agriculture and human health, and because of the scarcity of knowledge. Moreover, comprehensive studies are needed to clarify the appropriate combinations of amendments and soil properties.
ACKNOWLEDGMENTS
We acknowledge associate professor Shingo Mastumoto (Shimane University) and Dr. Tadashi Ito and Dr. Shimpei Nakagawa (Akita Agricultural Experiment Station) for soil sampling. The authors also appreciate Dr. Tamao Hatta and Ms. Seiko Nemoto (Japan International Research Center for Agricultural Science) for their support in using the X-ray fluorescence spectrometer. We also thank Dr. Tomohito Arao, Dr. Akira Kawasaki and Dr. Yuji Maejima for their useful suggestions. This work was supported by a Grant-in-Aid for the Research Project for Improving Food Safety and Animal Health, As-210.
REFERENCES
- Arai Y 2010: Arsenic and antimony. In Trace Elements in Soils, Ed. Peter SH, pp. 383–407. John Wiley & Sons, Ltd, Chichester, UK.
- Arao T, Kawasaki A, Baba K, Mori S, Matsumoto S 2009: Effects of water management on cadmium and arsenic accumulation and dimethylarsinic acid concentrations in Japanese rice. Environ. Sci. Technol., 43, 9361–9367. doi:10.1021/es9022738
- Blakemore LC, Searle PL, Darly BK 1987: Extractable iron, aluminum and silicon. In Methods for Chemical Analysis of Soils, New Zealand Soil Bureau Scientific Report 10A, Ed. Blakemore L, pp. 71–76. Lower Hutt, NZ, DSIR.
- Bogdan K, Schenk MK 2008: Arsenic in rice (Oryza sativa L.) related to dynamics of arsenic and silicic acid in paddy soils. Environ. Sci. Technol., 42, 7885–7890. doi:10.1021/es801194q
- Brechbühl Y, Christl I, Elzinga EJ, Kretzschmar R 2012: Competitive sorption of carbonate and arsenic to hematite: combined ATR-FTIR and batch experiments. J. Colloid Interface Sci., 377, 313–321. doi:10.1016/j.jcis.2012.03.025
- Christl I, Brechbühl Y, Graf M, Kretzschmar R 2012: Polymerization of silicate on hematite surfaces and its influence on arsenic sorption. Environ. Sci. Technol., 46, 13235–13243. doi:10.1021/es303297m
- De La Fuente C, Clemente R, Alburquerque JA, Vélez D, Bernal MP 2010: Implications of the use of As-rich groundwater for agricultural purposes and the effects of soil amendments on As solubility. Environ. Sci. Technol., 44, 9463–9469. doi:10.1021/es102012s
- Dixit S, Hering JG 2003: Comparison of arsenic(V) and arsenic(III) sorption onto iron oxide minerals: implications for arsenic mobility. Environ. Sci. Technol., 37, 4182–4189. doi:10.1021/es030309t
- Ekstrom EB, Learman DR, Madden AS, Hansel CM 2010: Contrasting effects of Al substitution on microbial reduction of Fe(III) (hydr)oxides. Geochim. Cosmochim. Acta, 74, 7086–7099. doi:10.1016/j.gca.2010.09.008
- Gee GW, Bauder JW 1986: Particle-size analysis. In Methods of Soil Analysis, Part 1, Physical and Mineralogical Methods, Ed. Klute A, pp. 383–411. American Society of Agronomy, Inc.; Soil Science Society of America, Inc., Madison.
- Goh K-H, Lim -T-T, Dong Z 2008: Application of layered double hydroxides for removal of oxyanions: a review. Water Res., 42, 1343–1368. doi:10.1016/j.watres.2007.10.043
- Horikita T, Ito T, Hasegawa T, Masuda Y, Arai T, Saigusa M 2007: Properties of polysilicate-iron (PSI) sludge from water purification plants: Its effects on rice growth and methane emission. Jpn. J. Soil Sci. Plant Nutr., 78, 261–267 (in Japanese with English summary).
- Hosoda K 1942: Studies on the improvement of mineral polluted soils. (V). J. Sci. Soil Manure, Jpn., 16, 459–466.
- Iimura K 1993: Chemical forms and behavior of heavy metals in soil. Soil Phys. Cond. Plant Growth, Jpn, 67, 19–23 (in Japanese).
- Inahara M, Ogawa Y, Azuma H 2007: Countermeasure by means of flooding in later growth stage to restrain cadmium uptake by lowland rice. Jpn. J. Soil Sci. Plant Nutr., 78, 149–155. (in Japanese).
- Jain A, Loeppert R 2000: Effect of competing anions on the adsorption of arsenate and arsenite by ferrihydrite. J. Environ. Qual., 29, 1422–1430. doi:10.2134/jeq2000.00472425002900050008x
- Kikuchi T, Okazaki M, Kimura S, Motobayashi T, Baasansuren J, Hattori T, Abe T 2008: Suppressive effects of magnesium oxide materials on cadmium uptake and accumulation into rice grains - II: suppression of cadmium uptake and accumulation into rice grains due to application of magnesium oxide materials. J. Hazard. Mater., 154, 294–299. doi:10.1016/j.jhazmat.2007.10.025
- Komárek M, Vaněk A, Ettler V 2013: Chemical stabilization of metals and arsenic in contaminated soils using oxides–a review. Environ. Pollut., 172, 9–22. doi:10.1016/j.envpol.2012.07.045
- Liu W-J, McGrath SP, Zhao F-J 2014: Silicon has opposite effects on the accumulation of inorganic and methylated arsenic species in rice. Plant Soil, 376, 423–431. doi:10.1007/s11104-013-1991-7
- Liu Y, Li Q, Gao S, Shang JK 2011: Exceptional As(III) sorption capacity by highly porous magnesium oxide nanoflakes made from hydrothermal synthesis. J. Am. Ceram. Soc., 94, 217–223. doi:10.1111/j.1551-2916.2010.04043.x
- Masscheleyn P 1991: Effect of redox potential and pH on arsenic speciation and solubility in a contaminated soil. Environ. Sci. Technol., 25, 1414–1419. doi:10.1021/es00020a008
- Masue Y, Loeppert RH, Kramer TA 2007: Arsenate and arsenite adsorption and desorption behavior on coprecipitated aluminum: ironhydroxides. Environ. Sci. Technol., 41, 837–842. doi:10.1021/es061160z
- Mench M, Bussière S, Boisson J 2003: Progress in remediation and revegetation of the barren Jales gold mine spoil after in situ treatments. Plant Soil, 249, 187–202. doi:10.1023/A:1022566431272
- Ministry of Agriculture, Forestry and Fisheries of Japan 2006: Cadmium intake from foods. http://www.maff.go.jp/j/syouan/nouan/kome/k_cd/kaisetu/pdf/A11.pdf (October, 2014; in Japanese)
- Ministry of Agriculture, Forestry and Fisheries of Japan 2014: Arsenic intake from foods. http://www.maff.go.jp/j/syouan/nouan/kome/k_as/exposure.html#1 (October, 2014; in Japanese)
- Mohan D, Pittman CU 2007: Arsenic removal from water/wastewater using adsorbents–A critical review. J. Hazard. Mater., 142, 1–53. doi:10.1016/j.jhazmat.2007.01.006
- Morimoto K, Anraku S, Hoshino J, Yoneda T, Sato T 2012: Surface complexation reactions of inorganic anions on hydrotalcite-like compounds. J. Colloid Interface Sci., 384, 99–104. doi:10.1016/j.jcis.2012.06.072
- Norton GJ, Adomako EE, Deacon CM, Carey A-M, Price AH, Meharg AA 2013: Effect of organic matter amendment, arsenic amendment and water management regime on rice grain arsenic species. Environ. Pollut., 177, 38–47. doi:10.1016/j.envpol.2013.01.049
- Ono M, Sugiyama S, Yokota S, Yamazaki A 2011: Water purification system by using the nano-crystalline layered double hydroxides (LDH). Nendo Kagaku, 49, 108–112 (in Japanese).
- Rahaman S, Sinha A, Mukhopadhyay D 2011: Effect of water regimes and organic matters on transport of arsenic in summer rice (Oryza sativa L.). J. Environ. Sci., 23, 633–639.
- Raven KP, Jain A, Loeppert RH 1998: Arsenite and arsenate adsorption on ferrihydrite: kinetics, equilibrium, and adsorption envelopes. Environ. Sci. Technol., 32, 344–349. doi:10.1021/es970421p
- Sato Y, Ito T, Horikawa T 2008: Effects of application of animal manure composts with polysilicate-iron sludge from water purification plant on rice growth and yield, and methane emission from paddy soil (preliminary report). Bull. Integr. Field Sci. Center, 25, 7–13.
- Schwertmann U, Taylor RM 1989: Iron oxides. In Minerals in Soil Environments, Eds. Dixon JB, Weed SB, pp. 379–438. Soil Science Society of America, Inc., Madison.
- Seyfferth AL, Fendorf S 2012: Silicate mineral impacts on the uptake and storage of arsenic and plant nutrients in rice (Oryza sativa L.). Environ. Sci. Technol., 46, 13176–13183. doi:10.1021/es3025337
- Soil Survey Staff 1998: Keys to Soil Taxonomy 8th Edn. USDA, National Resources Conservation Service, Washington, DC.
- Swedlund PJ, Webster JG 1999: Adsorption and polymerisation of silicic acid on ferrihydrite, and its effect on arsenic adsorption. Water Res., 33, 3413–3422. doi:10.1016/S0043-1354(99)00055-X
- Wada S, Morishita T 2013: Stabilization by magnesium related chemical mineralogical reactions. Nendo Kagaku, 51, 107–117 (in Japanese).
- Waltham C, Eick M 2002: Kinetics of arsenic adsorption on goethite in the presence of sorbed silicic acid. Soil Sci. Soc. Am. J., 66, 818–825. doi:10.2136/sssaj2002.8180
- Wang S, Mulligan CN 2006a: Effect of natural organic matter on arsenic release from soils and sediments into groundwater. Environ. Geochem. Heal., 28, 197–214. doi:10.1007/s10653-005-9032-y
- Wang S, Mulligan CN 2006b: Natural attenuation processes for remediation of arsenic contaminated soils and groundwater. J. Hazard. Mater., 138, 459–470. doi:10.1016/j.jhazmat.2006.09.048
- Weber F-A, Hofacker AF, Voegelin A, Kretzschmar R 2010: Temperature dependence and coupling of iron and arsenic reduction and release during flooding of a contaminated soil. Environ. Sci. Technol., 44, 116–122. doi:10.1021/es902100h
- Xie ZM, Huang CY 1998: Control of arsenic toxicity in rice plants grown on an arsenic‐polluted paddy soil. Commun. Soil Sci. Plant Anal., 29, 2471–2477. doi:10.1080/00103629809370125
- Yamaguchi N, Nakamura T, Dong D, Takahashi Y, Amachi S, Makino T 2011: Arsenic release from flooded paddy soils is influenced by speciation, Eh, pH, and iron dissolution. Chemosphere, 83, 925–932. doi:10.1016/j.chemosphere.2011.02.044
- Yang L, Shahrivari Z, Liu P 2005: Removal of trace levels of arsenic and selenium from aqueous solutions by calcined and uncalcined layered double hydroxides (LDH). Ind. Eng. Chem. Res., 44, 6804–6815. doi:10.1021/ie049060u
- You Y, Zhao H, Vance G 2001: Removal of arsenite from aqueous solutions by anionic clays. Environ. Technol., 22, 1447–1457. doi:10.1080/09593332208618178